OVERVIEW OF BACK AND VERTEBRAL COLUMN
Vertebrae
Structure and Function of Vertebrae
Regional Characteristics of Vertebrae
TABLE 4.1. Cervical Vertebrae
TABLE 4.2. Thoracic Vertebrae
TABLE 4.3. Lumbar Vertebrae
Ossification of Vertebrae
Variations in Vertebrae
BLUE BOX: Vertebrae. Vertebral Body Osteoporosis; Laminectomy; Dislocation of Cervical Vertebrae; Fracture and Dislocation of Atlas; Fracture and Dislocation of Axis; Lumbar Spinal Stenosis; Cervical Ribs; Caudal Epidural Anesthesia; Injury of Coccyx; Abnormal Fusion of Vertebrae; Effect of Aging on Vertebrae; Anomalies of Vertebrae
VERTEBRAL COLUMN
Joints of Vertebral Column
Movements of Vertebral Column
Curvatures of Vertebral Column
Vasculature of Vertebral Column
Nerves of Vertebral Column
BLUE BOX: Vertebral Column. Aging of Intervertebral Discs; Herniation of Nucleus Pulposus; Fracture of Dens of Axis; Rupture of Transverse Ligament of Atlas; Rupture of Alar Ligaments; Fractures and Dislocations of Vertebrae; Injury and Disease of Zygapophysial Joints; Back Pain; Abnormal Curvatures of Vertebral Column 474
MUSCLES OF BACK
Extrinsic Back Muscles
Intrinsic Back Muscles
TABLE 4.4. Superficial Layer of Intrinsic Back Muscles
TABLE 4.5. Intermediate Layer of Intrinsic Back Muscles
TABLE 4.6. Deep Layers of Intrinsic Back Muscles
TABLE 4.7. Principal Muscles Producing Movement of Cervical Intervertebral Joints
TABLE 4.8. Principal Muscles Producing Movements of Thoracic and Lumbar Intervertebral (IV) Joints
Surface Anatomy of Back Muscles
Suboccipital and Deep Neck Muscles
TABLE 4.9. Suboccipital Muscles and Suboccipital Triangle
TABLE 4.10. Principal Muscles Producing Movement of Atlanto-Occipital Joints
TABLE 4.11. Principal Muscles Producing Movement of Atlanto-Axial Joints
TABLE 4.12. Nerves of Posterior Cervical Region, Including Suboccipital Region (Triangles)
BLUE BOX: Muscles of Back. Back Strains, Sprains, and Spasms; Reduced Blood Supply to Brainstem
CONTENTS OF VERTEBRAL CANAL
Spinal Cord
Spinal Nerves and Nerve Roots
TABLE 4.13. Numbering of Spinal Nerves and Vertebrae
Spinal Meninges and Cerebrospinal Fluid (CSF)
TABLE 4.14. Spaces Associated with Spinal Meninges
Vasculature of Spinal Cord and Spinal Nerve Roots
BLUE BOX: Contents of Vertebral Canal. Compression of Lumbar Spinal Nerve Roots; Myelography; Development of Meninges and Subarachnoid Space; Lumbar Spinal Puncture; Spinal Anesthesia; Epidural Anesthesia (Blocks); Ischemia of Spinal Cord; Spinal Cord Injuries
OVERVIEW OF BACK AND VERTEBRAL COLUMN
The back comprises the posterior aspect of the trunk, inferior to the neck and superior to the buttocks. It is the region of the body to which the head, neck, and limbs are attached. The back includes the:
• Skin and subcutaneous tissue.
• Muscles: a superficial layer, primarily concerned with positioning and moving the upper limbs, and deeper layers (“true back muscles”), specifically concerned with moving or maintaining the position of the axial skeleton (posture).
• Vertebral column: the vertebrae, intervertebral (IV) discs, and associated ligaments (Fig. 4.1).
• Ribs (in the thoracic region): particularly their posterior portions, medial to the angles of the ribs.
• Spinal cord and meninges (membranes that cover the spinal cord).
• Various segmental nerves and vessels.
FIGURE 4.1. Vertebral column and its five regions. The isolated vertebrae between (A) and (B) are typical of each of the three mobile regions of the vertebral column. The continuous, weight-bearing column of vertebral bodies and IV discs increases in size as the column descends. Zygapophysial (facet) joints representative of each region are circled. The posterior view (C) includes the vertebral ends of ribs, representing the skeleton of the back. The bisected vertebral column in (D) demonstrates the vertebral canal. The intervertebral (IV) foramina (also seen in B) are openings in the lateral wall of the vertebral canal through which spinal nerves exit.
Because of their close association with the trunk, the back of the neck and the posterior and deep cervical muscles and vertebrae are also described in this chapter. The scapulae, although located in the back, are part of the appendicular skeleton and are considered with the upper limb (Chapter 6).
Study of the soft tissues of the back is best preceded by examination of the vertebrae and the fibrocartilaginous intervertebral discs that are interposed between the bodies of adjacent vertebrae. The vertebrae and IV discs collectively make up the vertebral column (spine), the skeleton of the neck and back that is the main part of the axial skeleton (i.e., articulated bones of the cranium, vertebral column, ribs, and sternum) (Fig. 4.1D). The vertebral column extends from the cranium (skull) to the apex of the coccyx. In adults, it is 72–75 cm long, of which approximately one quarter is formed by the IV discs that separate and bind the vertebrae together. The vertebral column:
• Protects the spinal cord and spinal nerves.
• Supports the weight of the body superior to the level of the pelvis.
• Provides a partly rigid and flexible axis for the body and an extended base on which the head is placed and pivots.
• Plays an important role in posture and locomotion (the movement from one place to another).
VERTEBRAE
The vertebral column in an adult typically consists of 33 vertebrae arranged in five regions: 7 cervical, 12 thoracic, 5 lumbar, 5 sacral, and 4 coccygeal (Fig. 4.1A–D). Significant motion occurs only between the 25 superior vertebrae. Of the 9 inferior vertebrae, the 5 sacral vertebrae are fused in adults to form the sacrum, and after approximately age 30, the 4 coccygeal vertebrae fuse to form the coccyx. The lumbosacral angle occurs at the junction of the long axes of the lumbar region of the vertebral column and the sacrum (Fig. 4.1D). The vertebrae gradually become larger as the vertebral column descends to the sacrum and then become progressively smaller toward the apex of the coccyx (Fig. 4.1A–D). The change in size is related to the fact that successive vertebrae bear increasing amounts of the body’s weight as the column descends. The vertebrae reach maximum size immediately superior to the sacrum, which transfers the weight to the pelvic girdle at the sacro-iliac joints.
The vertebral column is flexible because it consists of many relatively small bones, called vertebrae (singular = vertebra), that are separated by resilient IV discs (Fig. 4.1D). The 25 cervical, thoracic, lumbar, and first sacral vertebrae also articulate at synovial zygapophysial (facet) joints (Fig. 4.2D), which facilitate and control the vertebral column’s flexibility. Although the movement between two adjacent vertebrae is small, in aggregate the vertebrae and IV discs uniting them form a remarkably flexible yet rigid column that protects the spinal cord it surrounds.
FIGURE 4.2. Typical vertebra, represented by the 2nd lumbar vertebra. A. Functional components include the vertebral body (bone color), a vertebral arch (red), and seven processes: three for muscle attachment and leverage (blue) and four that participate in synovial joints with adjacent vertebrae (yellow). B and C. Bony formations of vertebrae are demonstrated. The vertebral foramen is bounded by the vertebral arch and body. A small superior vertebral notch and a larger inferior vertebral notch flank the pedicle. D. The superior and inferior notches of adjacent vertebrae plus the IV disc that unites them form the IV foramen for passage of a spinal nerve and its accompanying vessels. Each articular process has an articular facet where contact occurs with the articular facets of adjacent vertebrae (B–D).
Structure and Function of Vertebrae
Vertebrae vary in size and other characteristics from one region of the vertebral column to another, and to a lesser degree within each region; however, their basic structure is the same. A typical vertebra (Fig. 4.2) consists of a vertebral body, a vertebral arch, and seven processes.1
The vertebral body is the more massive, roughly cylindrical, anterior part of the bone that gives strength to the vertebral column and supports body weight. The size of the vertebral bodies increases as the column descends, most markedly from T4 inferiorly, as each bears progressively greater body weight.
The vertebral body consists of vascular, trabecular (spongy, cancellous) bone enclosed by a thin external layer of compact bone (Fig. 4.3). The trabecular bone is a meshwork of mostly tall vertical trabeculae intersecting with short, horizontal trabeculae. The spaces between the trabeculae are occupied by red bone marrow that is among the most actively hematopoietic (blood-forming) tissues of the mature individual. One or more large foramina in the posterior surface of the vertebral body accommodate basivertebral veins that drain the marrow (see Fig. 4.26).
FIGURE 4.3. Internal aspects of vertebral bodies and vertebral canal. The bodies consist largely of trabecular (spongy) bone—with tall, vertical supporting trabeculae linked by short horizontal trabeculae—covered by a relatively thin layer of compact bone. Hyaline cartilage “end plates” cover the superior and inferior surfaces of the bodies, surrounded by smooth bony epiphysial rims. The posterior longitudinal ligament, covering the posterior aspect of the bodies and linking the IV discs, forms the anterior wall of the vertebral canal. Lateral and posterior walls of the vertebral canal are formed by vertebral arches (pedicles and laminae) alternating with IV foramina and ligamenta flava.
FIGURE 4.26. Venous drainage of vertebral column. A. The venous drainage parallels the arterial supply and enters the external and internal vertebral venous plexuses. There is also anterolateral drainage from the external aspects of the vertebrae into segmental veins. B. The dense plexus of thin-walled vessels within the vertebral canal, the internal vertebral venous plexuses, consists of valveless anastomoses between anterior and posterior longitudinal venous sinuses.
In life, most of the superior and inferior surfaces of the vertebral body are covered with discs of hyaline cartilage (vertebral “end plates”), which are remnants of the cartilaginous model from which the bone develops. In dried laboratory and museum skeletal specimens, this cartilage is absent, and the exposed bone appears spongy, except at the periphery where an epiphysial rim or ring of smooth bone, derived from an anular epiphysis, is fused to the body (Fig. 4.2B).
In addition to serving as growth zones, the anular epiphyses and their cartilaginous remnants provide some protection to the vertebral bodies and permit some diffusion of fluid between the IV disc and the blood vessels (capillaries) in the vertebral body (see Fig. 4.26). The superior and inferior epiphyses usually unite with the centrum, the primary ossification center for the central mass of the vertebral body (Fig. 4.2B), early in adult life (at approximately age 25) (see “Ossification of Vertebrae,” p. 453).
The vertebral arch is posterior to the vertebral body and consists of two (right and left) pedicles and laminae (Fig. 4.2A & C). The pedicles are short, stout cylindrical processes that project posteriorly from the vertebral body to meet two broad, flat plates of bone, called laminae, which unite in the midline. The vertebral arch and the posterior surface of the vertebral body form the walls of the vertebral foramen (Fig. 4.2A & B). The succession of vertebral foramina in the articulated vertebral column forms the vertebral canal (spinal canal). The canal contains the spinal cord and the roots of the spinal nerves, along with the membranes (meninges), fat, and vessels that surround and serve them (Figs. 4.1D and 4.3). (See the blue box “Laminectomy,” p. 457.)
The vertebral notches are indentations observed in lateral views of the vertebrae superior and inferior to each pedicle between the superior and inferior articular processes posteriorly and the corresponding projections of the body anteriorly (Fig. 4.2C). The superior and inferior vertebral notches of adjacent vertebrae and the IV discs connecting them form intervertebral foramina (Fig. 4.2D) through which the spinal nerves emerge from the vertebral column (see Fig. 4.27). Also, the spinal (posterior root) ganglia are located in these foramina.
FIGURE 4.27. Innervation of periosteum and ligaments of vertebral column and of meninges. Except for the zygapophysial joints and external elements of the vertebral arch, the fibroskeletal structures of the vertebral column (and the meninges) are supplied by the (recurrent) meningeal nerves. Although usually omitted from diagrams and illustrations of spinal nerves, these fine nerves are the first branches to arise from all 31 pairs of spinal nerves and are the nerves that initially convey localized pain sensation from the back produced by acute herniation of an IV disc or from sprains, contusions, fractures, or tumors of the vertebral column itself. (Based on Frick H, Kummer B, Putz R. Wolf-Heidegger’s atlas of human anatomy, 4th ed. Basel: Karger AG, 1990:476.)
Seven processes arise from the vertebral arch of a typical vertebra (Fig. 4.2A–C):
• One median spinous process projects posteriorly (and usually inferiorly, typically overlapping the vertebra below) from the vertebral arch at the junction of the laminae.
• Two transverse processes project posterolaterally from the junctions of the pedicles and laminae.
• Four articular processes (G. zygapophyses)—two superior and two inferior—also arise from the junctions of the pedicles and laminae, each bearing an articular surface (facet).
The spinous and transverse processes provide attachment for deep back muscles and serve as levers, facilitating the muscles that fix or change the position of the vertebrae.
The articular processes are in apposition with corresponding processes of vertebrae adjacent (superior and inferior) to them, forming zygapophysial (facet) joints (Figs. 4.1B and 4.2D). Through their participation in these joints, these processes determine the types of movement permitted and restricted between the adjacent vertebrae of each region.
The articular processes also assist in keeping adjacent vertebrae aligned, particularly preventing one vertebra from slipping anteriorly on the vertebra below. Generally, the articular processes bear weight only temporarily, as when one rises from the flexed position, and unilaterally when the cervical vertebrae are laterally flexed to their limit. However, the inferior articular processes of the L5 vertebra bear weight even in the erect posture.
Regional Characteristics of Vertebrae
Each of the 33 vertebrae is unique; however, most of the vertebrae demonstrate characteristic features identifying them as belonging to one of the five regions of the vertebral column (e.g., vertebrae having foramina in their transverse processes are cervical vertebrae) (Fig. 4.4). In addition, certain individual vertebrae have distinguishing features; the C7 vertebra, for example, has the longest spinous process. It forms a prominence under the skin at the back of the neck, especially when the neck is flexed (see Fig. 4.8A).
FIGURE 4.4. Comparison of presacral vertebrae. As the vertebral column descends, bodies increase in size in relationship to increased weight-bearing. The size of the vertebral canal changes in relationship to the diameter of the spinal cord.
FIGURE 4.8. Surface anatomy of cervical and thoracic vertebrae. Except for the spinous process of the C7 vertebra (vertebra prominens), the visibility of the spinous processes depends on the abundance of subcutaneous tissue and the position of the back, neck, and upper limbs (especially protraction/retraction of scapulae). However, the spinous and thoracic transverse processes can usually be palpated in the mid- and paravertebral lines.
In each region, the articular facets are oriented on the articular processes of the vertebrae in a characteristic direction that determines the type of movement permitted between the adjacent vertebrae and, in aggregate, for the region. For example, the articular facets of thoracic vertebrae are nearly vertical, and together define an arc centered in the IV disc; this arrangement permits rotation and lateral flexion of the vertebral column in this region (Fig. 4.7). Regional variations in the size and shape of the vertebral canal accommodate the varying thickness of the spinal cord (Fig. 4.1D).
FIGURE 4.7. Thoracic vertebrae. Isolated (A) and articulated (B) typical thoracic vertebrae. (See also Fig. 4.4B.) In radiographs of the thoracic vertebrae, the articulating ribs obscure lateral features in anteroposterior views (C) and the vertebral arch components in lateral views (D). The uniformity of the vertebral bodies and radiographic “disc spaces” (caused by the radiolucency of the IV discs) are apparent.
CERVICAL VERTEBRAE
Cervical vertebrae form the skeleton of the neck (Fig. 4.1). The smallest of the 24 movable vertebrae, the cervical vertebrae are located between the cranium and the thoracic vertebrae. Their smaller size reflects the fact that they bear less weight than do the larger inferior vertebrae. Although the cervical IV discs are thinner than those of inferior regions, they are relatively thick compared to the size of the vertebral bodies they connect. The relative thickness of the IV discs, the nearly horizontal orientation of the articular facets, and the small amount of surrounding body mass give the cervical region the greatest range and variety of movement of all the vertebral regions.
The distinctive features of cervical vertebrae are illustrated in Figures 4.4A and 4.5 and described in Table 4.1. The most distinctive feature of each cervical vertebra is the oval foramen transversarium (transverse foramen) in the transverse process. The vertebral arteries and their accompanying veins pass through the transverse foramina, except those in C7, which transmit only small accessory veins. Thus the foramina are smaller in C7 than those in other cervical vertebrae, and occasionally they are absent.
FIGURE 4.5. Cervical vertebrae. A. C1, C2, and C3 vertebrae are atypical. B. The superior and inferior surfaces of the bodies of the cervical vertebrae are reciprocally convex and concave. Combined with the oblique orientation of the articular facets, this facilitates flexion and extension as well as lateral flexion. C. The anterior arch of the atlas lies anterior to the continuous curved line formed by the anterior surfaces of the C2–C7 vertebral bodies. (Courtesy of Dr. J. Heslin, Toronto, Ontario, Canada.)
TABLE 4.1. CERVICAL VERTEBRAEa
aThe C1, C2, and C7 vertebrae are atypical.
The transverse processes of cervical vertebrae end laterally in two projections: an anterior tubercle and a posterior tubercle. The tubercles provide attachment for a laterally placed group of cervical muscles (levator scapulae and scalenes). The anterior rami of the cervical spinal nerves course initially on the transverse processes in grooves for spinal nerves between the tubercles (Fig. 4.5A & B). The anterior tubercles of vertebra C6 are called carotid tubercles (Fig. 4.5A) because the common carotid arteries may be compressed here, in the groove between the tubercle and body, to control bleeding from these vessels. Bleeding may continue because of the carotid’s multiple anastomoses of distal branches with adjacent and contralateral branches, but at a slower rate.
Vertebrae C3–C7 are typical cervical vertebrae (Figs. 4.4A and 4.5A; Table 4.1). They have large vertebral foramina to accommodate the cervical enlargement of the spinal cord as a consequence of this region’s role in the innervation of the upper limbs. The superior borders of the transversely elongated bodies of the cervical vertebrae are elevated posteriorly and especially laterally, but they are depressed anteriorly, resembling somewhat a sculpted seat.
The inferior border of the body of the superiorly placed vertebra is reciprocally shaped. The adjacent cervical vertebrae articulate in a way that permits free flexion and extension and some lateral flexion but restricted rotation. The planar, nearly horizontal articular facets of the articular processes are also favorable for these movements. The elevated superolateral margin is the uncus of the body (uncinate process) (Fig. 4.4A).
The spinous processes of the C3–C6 vertebrae are short and usually bifid in white people, especially males, but usually not as commonly in people of African descent or in females (Duray et al., 1999). C7 is a prominent vertebra that is characterized by a long spinous process. Because of this prominent process, C7 is called the vertebra prominens. Run your finger along the midline of the posterior aspect of your neck until you feel the prominent C7 spinous process. It is the most prominent spinous process in 70% of people (Fig. 4.8A).
The two superior-most cervical vertebrae are atypical. Vertebra C1, also called the atlas, is unique in that it has neither a body nor a spinous process (Figs. 4.5A and 4.6B). This ring-shaped bone has paired lateral masses that serve the place of a body by bearing the weight of the globe-like cranium in a manner similar to the way that Atlas of Greek mythology bore the weight of the world on his shoulders (Fig. 4.6E). The transverse processes of the atlas arise from the lateral masses, causing them to be more laterally placed than those of the inferior vertebrae. This feature makes the atlas the widest of the cervical vertebrae, thus providing increased leverage for attached muscles.
FIGURE 4.6. Cranial base and C1 and C2 vertebrae. A. The occipital condyles articulate with the superior articular facets of the atlas (vertebra C1). B. The atlas, on which the cranium rests, has neither a spinous process nor a body. It consists of two lateral masses connected by anterior and posterior arches. C and D. The tooth-like dens characterizes the axis (vertebra C2) and provides a pivot around which the atlas turns and carries the cranium. It articulates anteriorly with the anterior arch of the atlas (“Facet for dens of axis,” in part B), and posteriorly with the transverse ligament of the atlas (see part B). E. Radiograph and articulated atlas and axis showing the dens projecting superiorly from the body of the axis between the lateral masses of the atlas. Since the atlas and axis lie posterior to the mandible (Fig. 4.5C), anteroposterior radiographs must be taken through the open mouth as indicated in the orientation figure.
The kidney-shaped, concave superior articular surfaces of the lateral masses articulate with two large cranial protuberances, the occipital condyles, at the sides of the foramen magnum (Fig. 4.6A). Anterior and posterior arches, each of which bears a tubercle in the center of its external aspect, extend between the lateral masses, forming a complete ring (Fig. 4.6B). The posterior arch, which corresponds to the lamina of a typical vertebra, has a wide groove for the vertebral artery on its superior surface. The C1 nerve also runs in this groove.
Vertebra C2, also called the axis, is the strongest of the cervical vertebrae (Figs. 4.5A and 4.6C). C1, carrying the cranium, rotates on C2 (e.g., when a person turns the head to indicate “no”). The axis has two large, flat bearing surfaces, the superior articular facets, on which the atlas rotates. The distinguishing feature of C2 is the blunt tooth-like dens (odontoid process), which projects superiorly from its body. Both the dens (G. tooth) and the spinal cord inside its coverings (meninges) are encircled by the atlas. The dens lies anterior to the spinal cord and serves as the pivot about which the rotation of the head occurs.
The dens is held in position against the posterior aspect of the anterior arch of the atlas by the transverse ligament of the atlas (Fig. 4.6B). This ligament extends from one lateral mass of the atlas to the other, passing between the dens and spinal cord, forming the posterior wall of the “socket” that receives the dens. Thus it prevents posterior (horizontal) displacement of the dens and anterior displacement of the atlas. Either displacement would compromise the portion of the vertebral foramen of C1 that gives passage to the spinal cord. C2 has a large bifid spinous process (Fig. 4.6C & D) that can be felt deep in the nuchal groove, the superficial vertical groove at the back of the neck.
THORACIC VERTEBRAE
The thoracic vertebrae are in the upper back and provide attachment for the ribs (Fig. 4.1). Thus the primary characteristic features of thoracic vertebrae are the costal facets for articulation with ribs. The costal facets and other characteristic features of thoracic vertebrae are illustrated in Figures 4.4B and 4.7 and listed in Table 4.2.
TABLE 4.2. THORACIC VERTEBRAE
The middle four thoracic vertebrae (T5–T8) demonstrate all the features typical of thoracic vertebrae. The articular processes extend vertically with paired, nearly coronally oriented articular facets that define an arc centered in the IV disc. This arc permits rotation and some lateral flexion of the vertebral column in this region. In fact, the greatest degree of rotation is permitted here (Fig. 4.7A). Attachment of the rib cage, combined with the vertical orientation of articular facets and overlapping spinous processes, limits flexion and extension as well as lateral flexion.
The T1–T4 vertebrae share some features of cervical vertebrae. T1 is atypical of thoracic vertebrae in that it has a long, almost horizontal spinous process that may be nearly as prominent as that of the vertebra prominens. T1 also has a complete costal facet on the superior edge of its body for the 1st rib and a demifacet on its inferior edge that contributes to the articular surface for the 2nd rib.
The T9–T12 vertebrae have some features of lumbar vertebrae (e.g., tubercles similar to the accessory processes). Mammillary processes (small tubercles) also occur on vertebra T12. However, most of the transition in characteristics of vertebrae from the thoracic to the lumbar region occurs over the length of a single vertebra: vertebra T12. Generally, its superior half is thoracic in character, having costal facets and articular processes that permit primarily rotatory movement, whereas its inferior half is lumbar in character, devoid of costal facets and having articular processes that permit only flexion and extension. Consequently, vertebra T12 is subject to transitional stresses that cause it to be the most commonly fractured vertebra.
SURFACE ANATOMY OF CERVICAL AND THORACIC VERTEBRAE
Several of the spinous processes can usually be observed, especially when the back is flexed and the scapulae are protracted (Fig. 4.8A); most of them can be palpated—even in an obese patient—because fat does not normally accumulate in the midline.
The tip of the C7 spinous process is the most evident superficially. Often, when the patient stands erect, it is the only spinous process visible (Fig. 4.8B); hence the name vertebra prominens. The spinous process of C2 can be felt deeply in the midline, inferior to the external occipital protuberance, a median projection located at the junction of the head and neck. C1 has no spinous process, and its small posterior tubercle is neither visible nor palpable.
The short bifid spinous processes of the C3–C5 vertebrae may be felt in the nuchal groove between the neck muscles, but they are not easy to palpate because the cervical lordosis, which is concave posteriorly, places them deep to the surface from which they are separated by the nuchal ligament. However, because it is considerably longer, the bifid spinous process of C6 vertebra is easily felt superior to the visible tip of the C7 process (vertebra prominens) when the neck is flexed.
When the neck and back are flexed, the spinous processes of the upper thoracic vertebra may also be seen. If the individual is especially lean, a continuous ridge appears linking their tips—the supraspinous ligament (Fig. 4.8C).
Although C7 is most commonly the most superior spinal process that is visible and readily palpable, the spinous process of T1 sometimes is more prominent. The spinous processes of the other thoracic vertebrae may be obvious in thin people, and in others can be identified by superior to inferior palpation beginning at the C7 spinous process. The tips of the thoracic spinous processes do not indicate the level of the corresponding vertebral bodies because they overlap (lie at the level of) the vertebra below (Figs. 4.1D and 4.7B & C).
When the back is not being flexed or the scapulae are not protracted, the tips of the thoracic spinous processes lie deep to a median longitudinal furrow (Fig. 4.8B & C). The tips of the spinous processes are normally in line with each other, even if the collective line wanders slightly from the midline. A sudden shift in the alignment of adjacent spinous processes may be the result of a unilateral dislocation of a zygapophysial joint; however, slight irregular misalignments may also result from a fracture of the spinous process. The short 12th rib, the lateral end of which can be palpated in the posterior axillary line, can be used to confirm identity of the T12 spinous process.
The transverse processes of C1 may be felt laterally by deep palpation between the mastoid processes (prominences of the temporal bones posterior to the ears) and the angles of the jaws. The carotid tubercle, the anterior tubercle of the transverse process of C6 vertebra, may be large enough to be palpable; the carotid artery lies anterior to it. In most people, the transverse processes of thoracic vertebrae can be palpated on each side of the spinous processes in the thoracic region. In lean individuals, the ribs can be palpated from the tubercle to the angle, at least in the lower back (inferior to the scapula) (see Figs. 1.1 and 1.2A).
LUMBAR VERTEBRAE
Lumbar vertebrae are in the lower back between the thorax and sacrum (Fig. 4.1). Characteristic features of lumbar vertebrae are illustrated in Figures 4.4C and 4.9 and described in Table 4.3. Because the weight they support increases toward the inferior end of the vertebral column, lumbar vertebrae have massive bodies, accounting for much of the thickness of the lower trunk in the median plane. Their articular processes extend vertically, with articular facets sagittally oriented initially (beginning abruptly with the T12–L1 joints), but becoming more coronally oriented as the column descends.
FIGURE 4.9. Lumbar vertebrae. Isolated (A–C) and articulated (D–F) typical lumbar vertebrae. In a lateral radiograph (E) the wedge shape of the lumbar vertebrae and especially the lumbar IV discs is evident. In anteroposterior radiographs (F) the vertebral canal is visible as a columnar shadow (between arrowheads); in lateral radiographs, the vertebral canal is primarily evident in the radiolucency of the IV foramina. (Courtesy of Dr. J. Heslin, Toronto, Ontario, Canada.)
TABLE 4.3. LUMBAR VERTEBRAE
The L5–S1 facets are distinctly coronal in orientation. In the more sagittally oriented superior joints, the laterally facing facets of the inferior articular processes of the vertebra above are “gripped” by the medially facing facets of the superior processes of the vertebra below, in a manner that facilitates flexion and extension and allows lateral flexion, but prohibits rotation (Fig. 4.9A, B, D, & E).
The transverse processes project somewhat posterosuperiorly as well as laterally. On the posterior surface of the base of each transverse process is a small accessory process, which provides an attachment for the intertransversarii muscles. On the posterior surface of the superior articular processes are small tubercles, the mammillary processes, which give attachment to both the multifidus and intertransversarii muscles of the back.
Vertebra L5, distinguished by its massive body and transverse processes, is the largest of all movable vertebrae. It carries the weight of the whole upper body. The L5 body is markedly taller anteriorly; therefore, it is largely responsible for the lumbosacral angle between the long axis of the lumbar region of the vertebral column and that of the sacrum (Fig. 4.1D). Body weight is transmitted from L5 vertebra to the base of the sacrum, formed by the superior surface of S1 vertebra (Fig. 4.10A).
FIGURE 4.10. Sacrum and coccyx. A. Base and pelvic surface of sacrum and coccyx. B. Dorsal surface of sacrum and coccyx. C. Lateral and anterior orientation drawings of the sacrum in its anatomical position demonstrate the essentially frontal plane and level at which the sacrum has been sectioned to reveal the sacral canal containing the cauda equina. Spinal ganglia lie within the IV foramina, as they do at superior vertebral levels. However, the sacral posterior and anterior rami of the spinal nerves exit via posterior and anterior (pelvic) sacral foramina, respectively. The lateral orientation drawing demonstrates the auricular surface that joins the ilium to form the synovial part of the sacro-iliac joint. In the anatomical position, the S1–S3 vertebrae lie in an essentially transverse plane, forming a roof for the posterior pelvic cavity. D. In anteroposterior radiographs the oblique plane of the auricular surfaces creates two lines indicating each sacro-iliac joint. The lateral line is the anterior aspect of the joint, the medial line is the posterior aspect.
SACRUM
The wedged-shaped sacrum (L. sacred bone) is usually composed of five fused sacral vertebrae in adults (Fig. 4.10). It is located between the hip bones and forms the roof and posterosuperior wall of the posterior half of the pelvic cavity. The triangular shape of the sacrum results from the rapid decrease in the size of the inferior lateral masses of the sacral vertebrae during development. The inferior half of the sacrum is not weight-bearing; therefore, its bulk is diminished considerably. The sacrum provides strength and stability to the pelvis and transmits the weight of the body to the pelvic girdle, the bony ring formed by the hip bones and sacrum, to which the lower limbs are attached.
The sacral canal is the continuation of the vertebral canal in the sacrum (Fig. 4.10B & C). It contains the bundle of spinal nerve roots arising inferior to the L1 vertebra, known as the cauda equina (L. horse tail), that descend past the termination of the spinal cord. On the pelvic and posterior surfaces of the sacrum between its vertebral components are typically four pairs of sacral foramina for the exit of the posterior and anterior rami of the spinal nerves (Fig. 4.10A–D). The anterior (pelvic) sacral foramina are larger than the posterior (dorsal) ones.
The base of the sacrum is formed by the superior surface of the S1 vertebra (Fig. 4.10A). Its superior articular processes articulate with the inferior articular processes of the L5 vertebra. The anterior projecting edge of the body of the S1 vertebra is the sacral promontory (L. mountain ridge), an important obstetrical landmark (see Chapter 3). The apex of the sacrum, its tapering inferior end, has an oval facet for articulation with the coccyx.
The sacrum supports the vertebral column and forms the posterior part of the bony pelvis. The sacrum is tilted so that it articulates with the L5 vertebra at the lumbosacral angle (Fig. 4.1D), which varies from 130° to 160°. The sacrum is often wider in proportion to length in the female than in the male, but the body of the S1 vertebra is usually larger in males.
The pelvic surface of the sacrum is smooth and concave (Fig. 4.10A). Four transverse lines on this surface of sacra from adults indicate where fusion of the sacral vertebrae occurred. During childhood, the individual sacral vertebrae are connected by hyaline cartilage and separated by IV discs. Fusion of the sacral vertebrae starts after age 20; however, most of the IV discs remain unossified up to or beyond middle life.
The dorsal surface of the sacrum is rough, convex, and marked by five prominent longitudinal ridges (Fig. 4.10B). The central ridge, the median sacral crest, represents the fused rudimentary spinous processes of the superior three or four sacral vertebra; S5 has no spinous process. The intermediate sacral crests represent the fused articular processes, and the lateral sacral crests are the tips of the transverse processes of the fused sacral vertebrae.
The clinically important features of the dorsal surface of the sacrum are the inverted U-shaped sacral hiatus and the sacral cornua (L. horns). The sacral hiatus results from the absence of the laminae and spinous process of S5 and sometimes S4. The sacral hiatus leads into the sacral canal. Its depth varies, depending on how much of the spinous process and laminae of S4 are present. The sacral cornua, representing the inferior articular processes of S5 vertebra, project inferiorly on each side of the sacral hiatus and are a helpful guide to its location.
The superior part of the lateral surface of the sacrum looks somewhat like an auricle (L. external ear); because of its shape, this area is called the auricular surface (Fig. 4.10B & C). It is the site of the synovial part of the sacro-iliac joint between the sacrum and ilium. During life, the auricular surface is covered with hyaline cartilage.
COCCYX
The coccyx (tail bone) is a small triangular bone that is usually formed by fusion of the four rudimentary coccygeal vertebrae, although in some people, there may be one less or one more (Fig. 4.10A–D). Coccygeal vertebra 1 (Co1) may remain separate from the fused group. The coccyx is the remnant of the skeleton of the embryonic tail-like caudal eminence, which is present in human embryos from the end of the 4th week until the beginning of the 8th week (Moore, Persaud and Torchia, 2012). The pelvic surface of the coccyx is concave and relatively smooth, and the posterior surface has rudimentary articular processes. Co1 is the largest and broadest of all the coccygeal vertebrae. Its short transverse processes are connected to the sacrum, and its rudimentary articular processes form coccygeal cornua, which articulate with the sacral cornua. The last three coccygeal vertebrae often fuse during middle life, forming a beak-like coccyx; this accounts for its name (G. coccyx, cuckoo). With increasing age, Co1 often fuses with the sacrum, and the remaining coccygeal vertebrae usually fuse to form a single bone.
The coccyx does not participate with the other vertebrae in support of the body weight when standing; however, when sitting it may flex anteriorly somewhat, indicating that it is receiving some weight. The coccyx provides attachments for parts of the gluteus maximus and coccygeus muscles and the anococcygeal ligament, the median fibrous band of the pubococcygeus muscles (see Chapter 3).
SURFACE ANATOMY OF LUMBAR VERTEBRAE, SACRUM, AND COCCYX
The spinous processes of lumbar vertebrae are large and easy to observe when the trunk is flexed (Fig. 4.11A). They can also be palpated in the posterior median furrow (Fig. 4.11B & C). The L2 spinous process provides an estimate of the position of the inferior end of the spinal cord. A horizontal line joining the highest points of the iliac crests passes through the tip of the L4 spinous process and the L4–L5 IV disc. This is a useful landmark when performing a lumbar puncture to obtain a sample of cerebrospinal fluid (CSF) (see the blue box “Lumbar Spinal Puncture,” p. 505).
FIGURE 4.11. Surface anatomy of lumbar vertebrae, sacrum, and coccyx.
The S2 spinous process lies at the middle of a line drawn between the posterior superior iliac spines, indicated by the skin dimples (Fig. 4.11B). The dimples are formed by the attachment of skin and deep fascia to these spines. This level indicates the inferior extent of the subarachnoid space (lumbar cistern). The median sacral crest can be felt inferior to the L5 spinous process. The sacral triangle is formed by the lines joining the two posterior superior iliac spines and the superior part of the intergluteal (natal) cleft between the buttocks. The sacral triangle outlining the sacrum is a common area of pain resulting from low back sprains. The sacral hiatus can be palpated at the inferior end of the sacrum located in the superior part of the intergluteal cleft.
The transverse processes of thoracic and lumbar vertebrae are covered with thick muscles and may or may not be palpable. The coccyx can be palpated in the intergluteal cleft, inferior to the apex of the sacral triangle. The apex of the coccyx can be palpated approximately 2.5 cm posterosuperior to the anus. Clinically, the coccyx is examined with a gloved finger in the anal canal.
Ossification of Vertebrae
Vertebrae begin to develop during the embryonic period as mesenchymal condensations around the notochord. Later, these mesenchymal bone models chondrify and cartilaginous vertebrae form. Typically, vertebrae begin to ossify toward the end of the embryonic period (8th week), with three primary ossification centers developing in each cartilaginous vertebra: an endochondral centrum, which will eventually constitute most of the body of the vertebra, and two perichondral centers, one in each half of the neural arch (Fig. 4.12A–J & M).
FIGURE 4.12. Ossification of vertebrae. A. Vertebra C1 (atlas) lacks a centrum. B and C. Vertebra C2 (axis) has two centra, one of which forms most of the dens. D–F. The development of “typical” cervical vertebrae is shown, including (D) the primary ossification centers within the hyaline cartilage, (E) a CT scan of the vertebra shown in part D (SC, spinal cord), and (F) the primary and secondary ossification centers. G–I. The development of thoracic vertebrae is shown, including (G) the three primary ossification centers in a cartilaginous vertebra of a 7-week-old embryo (observe the joints present at this stage), (H) the primary and secondary ossification centers (with ribs developed from costal elements), and (I) the bony parts of a thoracic vertebra after skeletonization (cartilage removed). J–L. The development of the lumbar vertebrae is shown, including (J) the primary and secondary ossification centers, (K) the anular epiphyses separated from the body, and (L) the anular epiphyses in place. M and N. The development of the sacrum is shown. Note that the ossification and fusion of sacral vertebrae may not be completed until age 35.
Ossification continues throughout the fetal period. At birth, typical vertebra and the superiormost sacral vertebrae consist of three bony parts united by hyaline cartilage. The inferior sacral vertebrae and all the coccygeal vertebrae are still entirely cartilaginous; they ossify during infancy. The halves of the neural arches articulate at neurocentral joints, which are primary cartilaginous joints (Fig. 4.12G). The halves of the neural/vertebral arch begin to fuse with each other posterior to the vertebral canal during the 1st year, beginning in the lumbar region and then in the thoracic and cervical regions. The neural arches begin fusing with the centra in the upper cervical region around the end of the 3rd year, but usually the process is not completed in the lower lumbar region until after the 6th year (Moore et al., 2012).
Five secondary ossification centers develop during puberty in each typical vertebra: one at the tip of the spinous process; one at the tip of each transverse process; and two anular epiphyses (ring epiphyses), one on the superior and one on the inferior edges of each vertebral body (i.e., around the margins of the superior and inferior surfaces of the vertebral body) (Fig. 4.12F & I–L).
The hyaline anular epiphyses, to which the IV discs attach, are sometimes referred to as epiphysial growth plates and form the zone from which the vertebral body grows in height. When growth ceases early in the adult period, the epiphyses usually unite with the vertebral body. This union results in the characteristic smooth raised margin, the epiphysial rim, around the edges of the superior and inferior surfaces of the body of the adult vertebra (Figs. 4.2B and 4.3). All secondary ossification centers have usually united with the vertebrae by age 25; however, the ages at which specific unions occur vary.
Exceptions to the typical pattern of ossification occur in vertebrae C1, C2, and C7 (Fig. 4.12A–C) and in the sacrum (Fig. 4.12M & N) and coccyx. In addition, at all levels, primordial “ribs” (costal elements) appear in association with the secondary ossification centers of the transverse processes (transverse elements). The costal elements normally develop into ribs only in the thoracic region; they become part of the transverse process or its equivalent at other levels.
In the cervical region, the costal element normally remains diminutive as part of the transverse process. Foramina transversarii develop as gaps between the two lateral ossification centers, medial to a linking costotransverse bar, which forms the lateral boundary of the foramina (Fig. 4.12A–F). Also, as a result of the cervical transverse processes being formed from the two developmental elements, the transverse processes of cervical vertebrae end laterally in an anterior tubercle (from the costal element) and a posterior tubercle (from the transverse element). The atypical morphology of vertebrae C1 and C2 is also established during development. The centrum of C1 becomes fused to that of C2 and loses its peripheral connection to the remainder of C1, thus forming the dens (Fig. 4.12C). Since these first two centra are fused and are now part of C2, no IV disc is formed between C1 and C2 to connect them. The part of the body that remains with C1 is represented by the anterior arch and tubercle of C1.
In the thoracic region, the costal elements separate from the developing vertebrae and elongate into ribs, and the transverse elements alone form the transverse processes (Fig. 4.12I).
All but the base of the transverse processes of the lumbar vertebrae develop from the costal element (Fig. 4.12J); this projecting bar of the mature bone is therefore called the costal process. The transverse elements of the lumbar vertebrae form the mammillary processes.
The ala and auricular surfaces of the sacrum are formed by the fusion of the transverse and costal elements.
Variations in Vertebrae
Most people have 33 vertebrae, but developmental errors may result in 32 or 34 vertebrae (Fig. 4.13). Estimates of the frequency of abnormal numbers of vertebrae superior to the sacrum (the normal number is 24) range between 5% and 12%. Variations in vertebrae are affected by race, gender, and developmental factors (genetic and environmental). An increased number of vertebrae occurs more often in males, and a reduced number occurs more frequently in females. Some races show more variation in the number of vertebrae. Variations in the number of vertebrae may be clinically important. An increased length of the presacral region of the vertebral column increases the strain on the inferior part of the lumbar region of the column owing to the increased leverage. However, most numerical variations are detected incidentally during diagnostic medical imaging studies being performed for other reasons and during dissections and autopsies of persons with no history of back problems.
FIGURE 4.13. Variations in vertebrae and their relationship to ribs. A. A “cranial shift” is demonstrated, in which there are 13 ribs, including a cervical rib articulating with vertebra C7, and a diminished 12th rib articulating with vertebra T12. Vertebra L5 is shown partially incorporated into the sacrum, but such “sacralization” can also be complete. The lowest sacral segment (S5) is partially segmented. B. The common arrangement of the vertebrae and the position of the 1st and 12th ribs are shown. C. A “caudal shift” is shown, in which the 12th rib is increased in size, and there is a small lumbar rib. The transverse process of vertebra L4 is increased in size, whereas that of vertebra L5 is greatly reduced. The first sacral segment is shown partially separated from the rest of the sacrum, but such “lumbarization” can also be complete. The 1st coccygeal segment is incorporated into the sacrum—that is, it is “sacralized.”
Caution is necessary, however, when describing an injury (e.g., when reporting the site of a vertebral fracture). When counting the vertebrae, begin at the base of the neck. The number of cervical vertebrae (seven) is remarkably constant (and not just in humans, but among vertebrates—even giraffes and snakes have seven cervical vertebrae!). When considering a numerical variation, the thoracic and lumbar regions must be considered together because people having more than five lumbar vertebrae often have a compensatory decrease in the number of thoracic vertebrae (O’Rahilly, 1986).
Variations in vertebrae also involve the relationship between the vertebrae and ribs, and the number of vertebrae that fuse to form the sacrum (Fig. 4.13). The relationship of presacral vertebrae to ribs and/or sacrum may occur higher (cranial shift) or lower (caudal shift) than normal. Note, however, that a C7 vertebra articulating with a rudimentary cervical rib(s) is still considered a cervical vertebra. The same is true for lumbar vertebrae and lumbar ribs. Likewise, an L5 vertebra fused to the sacrum is referred to as a “sacralized 5th lumbar vertebra” (see the blue box “Abnormal Fusion of Vertebrae,” p. 462).
VERTEBRAE
Vertebral Body Osteoporosis
Vertebral body osteoporosis is a common metabolic bone disease that is often detected during routine radiographic studies. Osteoporosis results from a net demineralization of the bones caused by a disruption of the normal balance of calcium deposition and resorption. As a result, the quality of bone is reduced and atrophy of skeletal tissue occurs. Although osteoporosis affects the entire skeleton, the most affected areas are the neck of the femur, the bodies of vertebrae, the metacarpals (bones of the hand), and the radius. These bones become weakened and brittle, and are subject to fracture.
Radiographs taken during early to moderate osteoporosis demonstrate demineralization, which is evident as diminished radiodensity of the trabecular (spongy) bone of the vertebral bodies, causing the thinned cortical bone to appear relatively prominent (Fig. B4.1B). Osteoporosis especially affects the horizontal trabeculae of the trabecular bone of the vertebral body (see Fig. 4.3). Consequently, vertical striping may become apparent, reflecting the loss of the horizontal supporting trabeculae and thickening of the vertical struts (Fig. B4.1A). Radiographs in later stages may reveal vertebral collapse (compression fractures) and increased thoracic kyphosis (Figs. 4.1C and B4.17B). Vertebral body osteoporosis occurs in all vertebrae but is most common in thoracic vertebrae and is an especially common finding in postmenopausal females.
FIGURE B4.1. Effects of osteoporosis on vertebral column. A. Early to moderate osteoporosis, characterized by vertical striation in the vertebral bodies. B. Later, the striated pattern is lost as the continued loss of trabecular bone produces uniform radiolucency (less white, more “transparent”). In contrast, the cortical bone, while thinned, appears relatively prominent. C. Late osteoporosis in the thoracic region of the vertebral column demonstrates excessive thoracic kyphosis, a result of the collapse of the vertebral bodies, which have become wedge-shaped (W), planar (P), and biconcave (B).
FIGURE B4.17. Abnormal curvatures of vertebral column.
Laminectomy
The surgical excision of one or more spinous processes and the adjacent supporting vertebral laminae in a particular region of the vertebral column is called a laminectomy (1 in Fig. B4.2A). The term is also commonly used to denote removal of most of the vertebral arch by transecting the pedicles (2 in Fig. B4.2A).
FIGURE B4.2. A. Sites at which laminectomies are performed. B. Posterior view, postlaminectomy.
Laminectomies are performed surgically (or anatomically in the dissection laboratory) to gain access to the vertebral canal, providing posterior exposure of the spinal cord (if performed above the L2 level) and/or the roots of specific spinal nerves. Surgical laminectomy is often performed to relieve pressure on the spinal cord or nerve roots caused by a tumor, herniated IV disc, or bony hypertrophy (excess growth).
Dislocation of Cervical Vertebrae
Because of their more horizontally oriented articular facets, the cervical vertebrae are less tightly interlocked than other vertebrae. The cervical vertebrae, “stacked like coins,” can be dislocated in neck injuries with less force than is required to fracture them (Fig. B4.3A–F). Because of the large vertebral canal in the cervical region, slight dislocation can occur here without damaging the spinal cord (Fig. B4.3B). Severe dislocations, or dislocations combined with fractures (fracture–dislocations) injure the spinal cord. If the dislocation does not result in “facet jumping” with locking of the displaced articular processes (Fig. B4.3F & G), the cervical vertebrae may self-reduce (slip back into place) so that a radiograph may not indicate that the cord has been injured. An MRI, however, may reveal the resulting soft tissue damage.
FIGURE B4.3 A–E.
FIGURE B4.3. Dislocations of cervical vertebrae. Four stages of injury are shown: (A) stage I, flexion sprain; (B) stage II, anterior subluxation with 25% anterior translation; (C) stage III, 50% translation; and (D) stage IV, complete dislocation. E. This lateral view radiograph shows a stage III dislocation with 50% translation. F. This MRI study of a stage IV dislocation with cord injury reveals that the body of C7 is fractured (open white arrowhead). The ligamentum flavum is disrupted (curved black arrow), and the spinous process is avulsed (straight black arrow). G. This transverse CT scan (same individual shown in F) reveals the reversed position of the articular processes of the C6 and C7 vertebrae owing to “facet jumping.”
Fracture and Dislocation of Atlas
The atlas (vertebra C1) is a bony ring, with two wedge-shaped lateral masses, connected by relatively thin anterior and posterior arches and a transverse ligament (Fig. 4.4A). Because the taller side of the lateral mass is directed laterally, vertical forces (as would result from striking the bottom of a pool in a diving accident) compressing the lateral masses between the occipital condyles and the axis drive them apart, fracturing one or both of the anterior or posterior arches (Fig. B4.4B).
FIGURE B4.4 A–B. Jefferson fracture of atlas.
If the force is sufficient, rupture of the transverse ligament that links them will also occur (Fig. B4.4C). The resulting Jefferson or burst fracture (Fig. B4.4C–E) in itself does not necessarily result in spinal cord injury, because the dimensions of the bony ring actually increase. Spinal cord injury is more likely, however, if the transverse ligament has also been ruptured (see the blue box “Rupture of Transverse Ligament of Atlas” on p. 477) indicated radiographically by widely spread lateral masses.
FIGURE B4.4 C–E. Jefferson fracture of atlas.
Fracture and Dislocation of Axis
Fractures of the vertebral arch of the axis (vertebra C2) are one of the most common injuries of the cervical vertebrae (up to 40%) (Yochum and Rowe, 2004). Usually the fracture occurs in the bony column formed by the superior and inferior articular processes of the axis, the pars interarticularis (Fig. 4.5A). A fracture in this location, called a traumatic spondylolysis of C2 (Fig. B4.5A, B, & D), usually occurs as a result of hyperextension of the head on the neck, rather than the combined hyperextension of the head and neck, which may result in whiplash injury.
Such hyperextension of the head was used to execute criminals by hanging, in which the knot was placed under the chin before the body suddenly dropped its length through the gallows floor (Fig. B4.5C); thus, this fracture has been called a hangman’s fracture.
In more severe injuries, the body of the C2 vertebra is displaced anteriorly with respect to the body of the C3 vertebra. With or without such subluxation (incomplete dislocation) of the axis, injury of the spinal cord and/or of the brainstem is likely, sometimes resulting in quadriplegia (paralysis of all four limbs) or death.
FIGURE B4.5 A–C. Fracture and dislocation of axis. Posterosuperior (A) and lateral (B) views of a hangman’s fracture of the C2 vertebra are shown (arrows). C. The position of the knot produces hyperextension during hanging (arrows). D. Right lateral view radiograph demonstrating a hangman’s fracture (arrow) of C2.
Fractures of the dens are also common axis injuries (40–50%), which may result from a horizontal blow to the head or as a complication of osteopenia (pathological loss of bone mass) (see the blue box “Fracture of Dens,” p. 476).
Lumbar Spinal Stenosis
Lumbar spinal stenosis describes a stenotic (narrow) vertebral foramen in one or more lumbar vertebrae (Fig. B4.6B). This condition may be a hereditary anomaly that can make a person more vulnerable to age-related degenerative changes such as IV disc bulging. Lumbar spinal nerves increase in size as the vertebral column descends, but paradoxically, the IV foramina decrease in size. Narrowing is usually maximal at the level of the IV discs. However, stenosis of a lumbar vertebral foramen alone may cause compression of one or more spinal nerve roots occupying the inferior vertebral canal (Fig. 4.1). Surgical treatment of lumbar stenosis may consist of decompressive laminectomy (see the blue box “Laminectomy,” p. 457). When IV disc protrusion occurs in a patient with spinal stenosis (Fig. B4.6B), it further compromises a vertebral canal that is already limited, as does arthritic proliferation and ligamentous degeneration.
FIGURE B4.6. Lumbar spinal stenosis. Normal (A) and stenotic (B) vertebral foramina are compared. The sagittal (C) and transverse (D) lumbar MRIs demonstrate a high-grade stenosis caused by hypertrophic articular processes and ligamenta flava and moderate peripheral bulging of the L4–L5 IV disc.
Cervical Ribs
A cervical rib is a relatively common anomaly. In 1–2% of people, the developmental costal element of C7, which normally becomes a small part of the transverse process that lies anterior to the foramen transversarium (Fig. 4.5A), becomes abnormally enlarged. This structure may vary in size from a small protuberance to a complete rib that occurs bilaterally about 60% of the time.
The supernumerary (extra) rib or a fibrous connection extending from its tip to the first thoracic rib may elevate and place pressure on structures that emerge from the superior thoracic aperture, notably the subclavian artery or inferior trunk of the brachial plexus, and may cause thoracic outlet syndrome.
Caudal Epidural Anesthesia
In living persons, the sacral hiatus is closed by the membranous sacrococcygeal ligament, which is pierced by the filum terminale (a connective tissue strand extending from the tip of the spinal cord to the coccyx). Deep (superior) to the ligament, the epidural space of the sacral canal is filled with fatty connective tissue (Fig. B4.7A). In caudal epidural anesthesia or caudal analgesia, a local anesthetic agent is injected into the fat of the sacral canal that surrounds the proximal portions of the sacral nerves. This can be accomplished by several routes, including the sacral hiatus (Fig. B4.7B & C). Because the sacral hiatus is located between the sacral cornua and inferior to the S4 spinous process or median sacral crest, these palpable bony landmarks are important for locating the hiatus (Fig. B4.7A). The anesthetic solution spreads superiorly and extradurally, where it acts on the S2–Co1 spinal nerves of the cauda equina. The height to which the anesthetic ascends is controlled by the amount injected and the position of the patient. Sensation is lost inferior to the epidural block. Anesthetic agents can also be injected through the posterior sacral foramina into the sacral canal around the spinal nerve roots (transsacral epidural anesthesia) (Fig. B4.7B). Epidural anesthesia during childbirth is discussed in Chapter 3.
FIGURE B4.7.
Injury of Coccyx
An abrupt fall onto the buttocks may cause a painful subperiosteal bruising or fracture of the coccyx, or a fracture—dislocation of the sacrococcygeal joint. Displacement is common, and surgical removal of the fractured bone may be required to relieve pain. An especially difficult childbirth occasionally injures the mother’s coccyx. A troublesome syndrome, coccygodynia (or coccydynia), often follows coccygeal trauma; pain relief is commonly difficult.
Abnormal Fusion of Vertebrae
In approximately 5% of people, L5 is partly or completely incorporated into the sacrum—conditions known as hemisacralization and sacralization of the L5 vertebra (Fig. B4.8A), respectively. In others, S1 is more or less separated from the sacrum and is partly or completely fused with L5 vertebra, which is called lumbarization of the S1 vertebra (Fig. B4.8B). When L5 is sacralized, the L5–S1 level is strong and the L4–L5 level degenerates, often producing painful symptoms.
FIGURE B4.8.
Effect of Aging on Vertebrae
Between birth and age 5, the body of a typical lumbar vertebra increases in height threefold (from 5–6 mm to 15–18 mm), and between ages 5 and 13, it increases another 45–50%. Longitudinal growth continues throughout adolescence, but the rate decreases and is completed between ages 18 and 25.
During middle and older age, there is an overall decrease in bone density and strength, particularly centrally within the vertebral body. Consequently, the articular surfaces gradually bow inward so that both the superior and inferior surfaces of the vertebrae become increasingly concave (Fig. B4.9A), and the IV discs become increasingly convex. The bone loss and consequent change in shape of the vertebral bodies may account in part for the slight loss in height that occurs with aging. The development of these concavities may cause an apparent narrowing of the intervertebral “space” on radiographs based on the distance between the margins of the vertebral bodies; however, this should not be interpreted as a loss of IV disc thickness.
FIGURE B4.9. Effects of aging on vertebrae.
Aging of the IV discs combined with the changing shape of the vertebrae results in an increase in compressive forces at the periphery of the vertebral bodies, where the discs attach. In response, osteophytes (bony spurs) commonly develop around the margins of the vertebral body (along the attachments of the fibers of the outer part of the disc), especially anteriorly and posteriorly (Fig. B4.9B). Similarly, as altered mechanics place greater stresses on the zygapophysial joints, osteophytes develop along the attachments of the joint capsules and accessory ligaments, especially those of the superior articular process, whereas extensions of the articular cartilage develop around the articular facets of the inferior processes.
This bony or cartilaginous growth during advanced age has traditionally been viewed as a disease process (spondylosis in the case of the vertebral bodies, osteoarthrosis in the case of the zygapophysial joints), but it may be more realistic to view it as an expected morphological change with age, representing normal anatomy for a particular age range.
Correlation of these findings with pain is often difficult. Some people with these manifestations present with pain, others demonstrate the same age-related changes but have no pain, and still others exhibit little morphological change but complain of the same types of pain as those with evident change. In view of this and the typical occurrence of these findings, some clinicians have suggested that such age-related changes should not be considered pathological, but as the normal anatomy of aging (Bogduk, 2012).
Anomalies of Vertebrae
Sometimes the epiphysis of a transverse process fails to fuse. Therefore, caution must be exercised so that a persistent epiphysis is not mistaken for a vertebral fracture in a radiograph or computed tomographic (CT) scan.
A common birth defect of the vertebral column is spina bifida occulta, in which the neural arches of L5 and/or S1 fail to develop normally and fuse posterior to the vertebral canal. This bony defect, present in up to 24% of the population (Greer, 2009), usually occurs in the vertebral arch of L5 and/or S1. The defect is concealed by the overlying skin, but its location is often indicated by a tuft of hair. Most people with spina bifida occulta have no back problems. When examining a newborn, adjacent vertebrae should be palpated in sequence to be certain the vertebral arches are intact and continuous from the cervical to the sacral regions.
In severe types of spina bifida, spina bifida cystica, one or more vertebral arches may fail to develop completely. Spina bifida cystica is associated with herniation of the meninges (meningocele, a spina bifida associated with a meningeal cyst) and/or the spinal cord (meningomyelocele) (Fig. B4.10). Neurological symptoms are usually present in severe cases of meningomyelocele (e.g., paralysis of the limbs and disturbances in bladder and bowel control). Severe forms of spina bifida result from neural tube defects, such as the defective closure of the neural tube during the 4th week of embryonic development (Moore, et al., 2012).
FIGURE B4.10. Infant with spina bifida cystica.
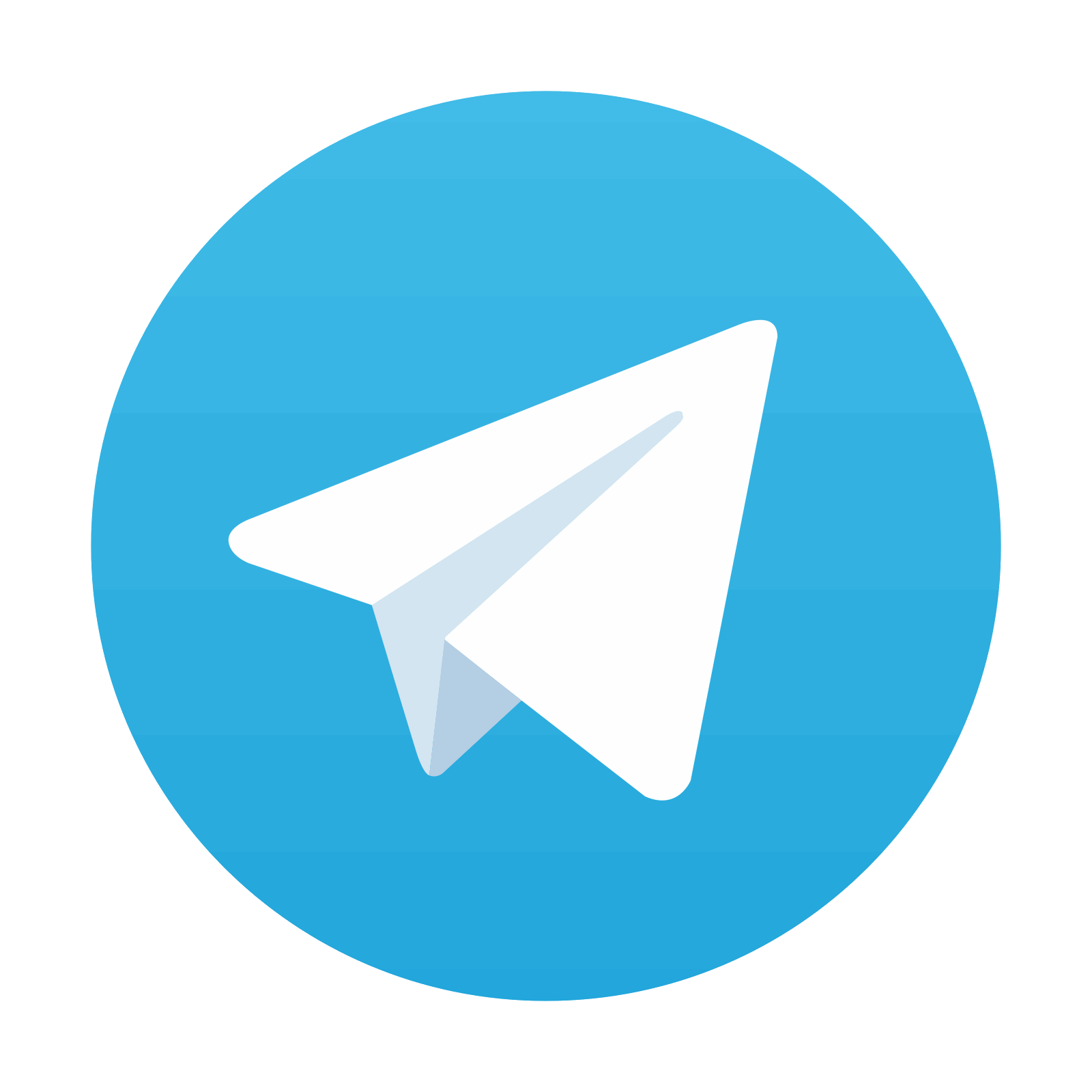
Stay updated, free articles. Join our Telegram channel

Full access? Get Clinical Tree
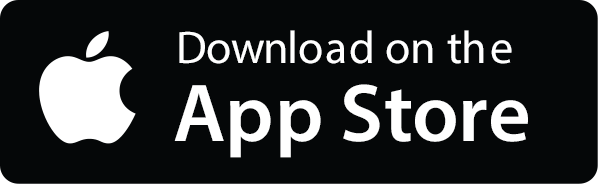
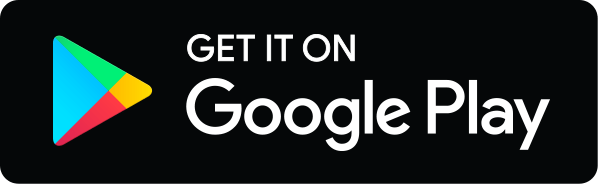