Fig. 19.1
Therapeutic vaccines for drug addiction elicit an adaptive immune response against drugs of abuse. The composition of the vaccine is critical for recognition by antigen-presenting cells (APC) and activation of antigen-specific B and T cells. (a) Drug targets, shown oxycodone and hydrocodone structures. (b) A conjugate immunogen is composed of (i) a hapten derivatized from the structure of the targeted drug of abuse, which represents the B cell epitope that binds hapten-specific B cells that will proliferate and mature into antibody-secreting and memory B cells; (ii) a larger immunogenic structure, called carrier, which activates carrier-specific CD4+ helper T cells by providing T cell epitopes (carrier proteins are digested by APCs, either macrophages or B cells, and presented to CD4+ T cells as smaller peptides bound to MHCII); and (iii) the conjugate is packaged in an adjuvant to increase its immunogenicity and provide an injectable formulation. The figure shows an oxycodone-based hapten containing a tetraglycine linker at the C6 position (6OXY(Gly)4) conjugated to the keyhole limpet hemocyanin (KLH) carrier protein
This is not a limitation of addiction vaccines per se, but it appears to generalize to other conjugate immunogens against small molecules. Synthetic glycan-based haptens are conjugated to carrier proteins to elicit antibodies that bind carbohydrates expressed on cancer cells, which then elicit antibody-dependent cell toxicity or complement-dependent cytotoxicity (Astronomo and Burton 2010). Similar to nicotine and cocaine vaccines, in a clinical trial of the sialyl Tn hapten conjugated to KLH, an increase in overall survival against metastatic breast cancer was observed only in vaccinated subjects developing high levels of Tn-specific serum antibodies (Ibrahim et al. 2013).
The mechanism(s) underlying individual variability in the magnitude of hapten-specific serum antibody responses is not well understood because, in comparison to protein-based infectious diseases vaccines, less is known about B cell responses specific for drugs of abuse or other clinically relevant small molecules (e.g., Tn antigen). To design better vaccines, it is important to understand the mechanisms underlying vaccine efficacy against the targeted drug and the observed variability across immunized subjects. Currently, it is not fully understood whether the large portion of nonresponders may be due to vaccine design, disease heterogeneity, or specific interactions between the vaccine’s components and the host immune system. The following sections will discuss concepts and proposed models that are based on current knowledge of B cells specific for known model antigens. As a cautionary note, a broad and general perspective on B cell biology that is relevant to the development of addiction vaccines will be provided.
19.2 Concepts of B Cell Biology
19.2.1 Cellular Biology and B Cell Phenotypes
Bone marrow produces immature lymphocyte precursors of B cells, whereas T cells are produced in the thymus (McHeyzer-Williams and McHeyzer-Williams 2005). Prior to immunization, the host immune system contains millions of naïve B cells circulating through the spleen and lymph nodes via the bloodstream. Each naïve B cell expresses a transmembrane form of antibody (immunoglobulin or Ig) called the B cell receptor (BCR) that is unique to each cell (Pape et al. 2011; Taylor et al. 2012a). The presence of a functional BCR defines a B cell identity. To enable adaptive immune responses against infections, vaccination, or the variety of antigens that individuals may encounter during a lifetime, the naïve (i.e., pre-immunization or in unimmunized subjects) B cell pool requires a large BCR repertoire. In mammals, BCR diversity is established through rearrangement of immunoglobulin heavy and light chain V, D, and J gene segments, a process known as V(D)J recombination (Tonegawa 1983). In addition to V(D)J recombination, other mechanisms contribute to the highly diverse BCR repertoire, such as addition or subtraction of nucleotides at gene segment junctions. Both B and T cells are main players in humoral adaptive immune responses to infections and vaccines. Yet B cells are the only immune cell type capable of generating antibodies against antigens, including drugs of abuse.
19.2.2 B Cell Ontogeny
In mammals, B cells are derived from two lineages, B-1 and B-2, and their commitment to either is based on complex processes (Scholz et al. 2014). It is not clear the extent to which B cells derive from a common precursor cell type and evolve into separate lineages driven by antigen selection, or alternatively originate from distinct precursors. The B-1 cell lineage has been well characterized in mice, whereas its relevance to the human immune system is less understood. In contrast, the B-2 lineage has been studied in depth in both mice and humans. In mice, prenatal production of the B-1 lymphocytes occurs in the liver, while the B-2 lineage emerges from the bone marrow in adults, and its is responsible for the generation of B cell pools found in the lymph nodes and spleen (Scholz et al. 2014). The characteristics of B-2 cell development and rearrangement of the immunoglobulin variable regions in mice provide a close preclinical model of human B cell and BCR processes.
19.2.3 Overview of T Cell-Dependent B Cell Generation of Antibodies Against Antigens
After immunization with the antigen of interest, T cell-dependent B cell activation largely occurs within secondary lymphoid organs in the germinal center (GC) (McHeyzer-Williams and McHeyzer-Williams 2005). The GC-dependent T cell-dependent B cell activation is a critical aspect of the adaptive immune response to generate mature switched immunoglobulin memory B cells and antibody-secreting plasma B cells, which are B cell types essential for the generation of long-term high-affinity antibody responses against antigens and responding to booster vaccine injections (McHeyzer-Williams and McHeyzer-Williams 2005).
Shortly after immunization (i.e., 3–7 days), within secondary lymphoid organs, proliferating antigen-specific B cells move toward the B/T cell border, a specific anatomical area of B and T cell interaction, and migrate deeper into follicles to form GC (McHeyzer-Williams and McHeyzer-Williams 2005). Germinal centers are dynamic hot spots where T cell-dependent B cell activation, somatic hypermutation, and affinity maturation processes occur (Victora and Nussenzweig 2012). The formation of GC involves coordinated interaction between activated antigen-specific B and T cells in the presence of a professional antigen-presenting cell (APC) displaying the major histocompatibility complex II (MHCII) receptor. In the GC, antigen-specific B cells interact with a subset of CD4+ T cells, known as T follicular helper cells (TfH), which specialize in sustaining B cell maturation (McHeyzer-Williams et al. 2012; Crotty 2011). CD4+ T cells promote GC B cell activation by a variety of signaling mechanisms that include either direct interaction through membrane receptors (e.g., CD40) or secretion of interleukins (e.g., IL-4) and other soluble molecules (Victora and Nussenzweig 2012).
Within the GC, antigen-specific B cells go through affinity maturation to become either switched immunoglobulin (swIg) memory B cells carrying specialized immunoglobulins (e.g., IgG, IgA, or IgE) or long-lived antibody-secreting plasma B cells (Taylor et al. 2012a). Both GC-dependent B cell maturation and TfH activation are dependent upon a complex transcriptional program involving balanced activity of a variety of downstream signals (McHeyzer-Williams et al. 2012; Crotty 2011). These pathways include the signal transducers and activators of transcription (STAT), the transcription factors B cell 6 lymphoma protein (bcl-6) and B lymphocyte-induced maturation protein 1 (blimp-1) (McHeyzer-Williams et al. 2012; Crotty 2011). Affinity maturation is a complex process that involves somatic hypermutation, class switch recombination, and clonal selection (Victora and Nussenzweig 2012). Somatic hypermutation, which is characterized by high mutations rates in B cells, involves the key effector activation-induced deaminase (AID) which introduces mutations in the variable regions of the V(D)J gene segments, to modify the binding affinity of the BCR (Maul and Gearhart 2010). Somatic hypermutation is critical to increase the diversity of the BCR within the B cell repertoire and generate immunoglobulins with high affinity for the target antigen. Similarly, class switch recombination is instrumental in generating B cells that switched their immunoglobulin from the low-affinity IgM, or IgD, to the more specialized IgG, IgA, and IgE. After the occurrence of somatic hypermutation and class switch recombination events, B cells will compete for the antigen and other resources through clonal selection. Only the antigen-specific B cell clones that have the highest affinity for the antigen will survive (McHeyzer-Williams and McHeyzer-Williams 2005; Victora and Nussenzweig 2012; McHeyzer-Williams et al. 2012).
19.3 B Cells Specific for Drugs of Abuse Haptens and Addiction Vaccines
In the field of addiction vaccines, limited information is available in regard to B and T cell responses to immunization. Most studies have focused on vaccine development and screening of the most effective vaccines for their ability to elicit antibodies, their effect on drug distribution to the serum and to the brain compartments, and their efficacy in preventing various drug-induced behaviors. As detailed in this book, these studies have focused on the role of hapten and conjugation chemistry, use of novel adjuvants and carriers, and new delivery platforms including nanoparticles or liposomes or synthetic materials. This wealth of literature provides an in-depth understanding of how vaccine components contribute to elicit the antibody response required for vaccine efficacy against drugs of abuse but provides only clues about the adaptive immune response underlying vaccine efficacy. Vaccine development will benefit from complementary studies aimed at understanding the molecular and cellular mechanisms underlying effective and long-lasting immunization against drugs of abuse.
In regard to adaptive immune responses to addiction vaccines, many questions remain unanswered. For instance, it is not clear whether the number of the B cells specific for a drug-based hapten or the number of carrier-specific T cells present prior to immunization determines the quantity and quality of the antibody response to vaccination. Recent studies have developed strategies to study B cells specific for drug-based haptens and showed that a greater population size of polyclonal hapten-specific B cells correlates to increased vaccine ability to induce drug-specific antibodies and their efficacy in preventing oxycodone distribution to the brain and its behavioral effects in mice. These novel analytical tools and data support screening new vaccine formulations for their ability to activate specific subsets of naïve B and T cells or to target critical aspects of the immune response.
19.3.1 T Cell-Dependent B Cell Responses to Haptens and Conjugate Vaccines
Addiction vaccines consist of drug-derived haptens chemically bound to larger foreign immunogenic structures that stimulate T cell-dependent B cell activation to generate antibodies against drugs of abuse (Fig. 19.1). The physical and chemical structure of the conjugate immunogen (i.e., the vaccine) is crucial for the coordinated activation of B and T cells, in the presence of APC. As shown in Fig. 19.1, a conjugate vaccine is composed of (1) a hapten derived from the structure of the targeted drug of abuse, which represents the B cell epitope that binds hapten-specific B cells that, in turn, will proliferate and mature into memory and antibody-secreting plasma B cells; (2) a larger immunogenic structure, called a carrier, which activates carrier-specific CD4+ helper T cells by providing T cell epitopes in the form of smaller peptide sequences presented by MHCII receptors on APC; and (3) an adjuvant that enhances immunogenicity of the conjugate through various mechanisms.
After immunization, haptens can directly bind to the BCR and activate hapten-specific B cells. However, the generation of antibodies against the drug-based hapten and free drug requires T cell-dependent B cell activation involving professional APC that display surface MHCII receptors, including macrophages, dendritic cells, and B cells. After immunization, macrophages and dendritic cells uptake the conjugate in a nonselective manner or carry the whole immunogen to the draining lymph node for processing. Alternatively, the antigen passively drains to the lymph nodes through the lymphatic system. After internalization, APC digest the carrier protein to shorter peptides, which are displayed on their MHCII receptors. Peptide presentation on MHCII receptors is necessary for engagement of CD4+ T helper cells. MHCII presentation by either B cells or other APC will engage T cell help through recognition by T cell receptors (TCR) specific for the peptide sequence derived from the carrier protein. MHCII-dependent activation of naïve T cells specific for peptides derived from carrier proteins promotes their differentiation into mature effector T cells including antigen-specific CD4+ T helper (TH) cells. CD4+ TH cells can be further subdivided in TH1 or TH2 cells, which activate either the cellular (e.g., antigen-specific CD8+ T cells or NK cells) or the humoral (i.e., B cells and antibodies) branch of the adaptive immune response, respectively. This is of particular importance for understanding the mechanisms underlying vaccine efficacy since most adjuvants are characterized by their ability to activate the TH1, TH2, or TH1/TH2 (for details on adjuvants, see Chap. 16). For example, alum is a known TH2 adjuvant while complete Freund’s adjuvant (CFA) activates a mixed TH1/TH2 response. Activated antigen-specific CD4+ TH cells support hapten-specific B cell clonal expansion and maturation by direct interaction through membrane receptors, such as CD40:CD40L, or by soluble molecules such as interleukins (e.g., IL-4, IL-6, and IL-21) and other inducible costimulatory signals (e.g., ICOS:ICOSL). Supported by TfH cells, activated B cells will then go through expansion, maturation, and differentiation in various B cell phenotypes including swIg memory B cells displaying high-affinity BCR or antibody-secreting B cells capable of secreting high-affinity switched immunoglobulins, such as IgG, specific for drugs of abuse. The next paragraphs will cover in detail the initiation and progression of hapten-specific B cell responses to conjugate vaccines for drug addiction.
19.3.2 Proposed Mechanisms of Hapten-Specific B Cell Responses to Immunization
Among the polyclonal naïve B cell population, very few B cells display a BCR with high affinity for the hapten of interest. After immunization with drug hapten-protein conjugates, successful activation and maturation of B cells that recognize the vaccine will generate high-affinity antibodies against the target drug. In secondary lymphoid organs, such as the spleen and lymph nodes, hapten-specific naïve B cells expressing surface immunoglobulins, which recognize and bind the vaccine, undergo activation and proliferation with the help of CD4+ T cells. Based on studies of protein-based antigens, we know that these processes generate (1) short-lived antibody-secreting B cells, (2) GC-independent differentiation in low-affinity IgM+ memory B cells, and (3) GC B cells (Fig. 19.2) (Taylor et al. 2012a). Germinal center B cells then mature into either long-lived antibody-secreting B cells (ASC or plasma cells) that maintain serum immunoglobulin levels or long-lived memory B cells which will switch their Ig constant region from IgM to IgG, IgA, or IgE while acquiring somatic mutations in their variable region (i.e., swIg B cells) (McHeyzer-Williams and McHeyzer-Williams 2005; Maul and Gearhart 2010; Tarlinton 2008). Long-lived antibody-secreting and memory B cells will then transit and reside to the bone marrow (i.e., homing). After subsequent boost immunizations, the IgM+ or swIg memory B cell subsets respond to the antigen challenge and generate more antibody-secreting B cells, which boost the amount of antigen-specific serum antibodies (Taylor et al. 2012a). Depending upon the timing of the secondary antigen challenge, memory B cells may form more IgM+ or swIg memory B cells and also GC B cells (Taylor et al. 2012a). These models are likely to apply to hapten-specific B cells elicited by addiction vaccine; however, their validity still remains to be tested.
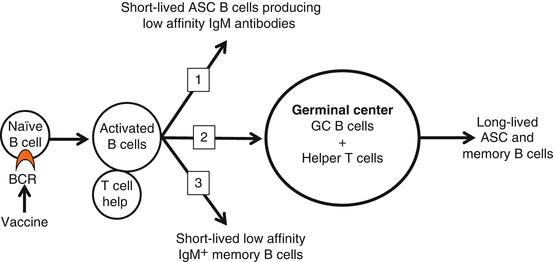
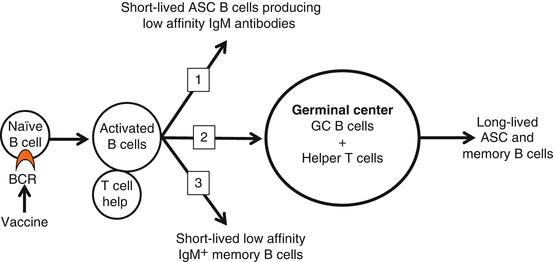
Fig. 19.2
T cell-dependent B cell activation. After immunization with conjugate immunogens, successful activation of naïve hapten-specific B cells is required for the generation of antibody-secreting cells (ASC) and the production of antibodies against the target drug. In the spleen and lymph nodes, naïve B cells expressing a BCR specific for the hapten will undergo activation and clonal expansion with the help of CD4+ T cells. This initial critical event will lead to the generation of (1) short-lived ASC B cells, (2) GC B cells and (3) low-affinity IgM+ memory B cells. In the GC, hapten-specific B cells mature into either long-lived ASC or swIg memory B cells
In subjects immunized against infectious diseases, exposure to the infectious agent (e.g., the hepatitis B virus) will activate antigen-specific memory B cells and induce an antigen-specific antibody response that will contain the infection. In contrast, in subjects immunized with vaccines for drug addiction, subsequent exposure to drugs of abuse, lacking T cell epitopes, is not sufficient to boost memory B cells and induce differentiation in antibody-secreting B cells. In fact, to maintain drug-specific antibody responses is necessary to administer boost immunizations with hapten-carrier conjugate immunogens. Current animal and human studies of addiction vaccines have employed immunization protocols that involved multiple repeated immunizations to rapidly achieve the required high antibody titers required to counteract plasma concentrations of drugs. Clinical trials of vaccines for nicotine and cocaine involved immunization regimens of 3–5 monthly doses followed by booster injections every 2 or 6 months to preserve serum anti-drug antibody levels (Cornuz et al. 2008; Hatsukami et al. 2011; Martell et al. 2009). Similar immunization regimens, involving repeated immunizations every few weeks with hapten-protein conjugates, have also been used to generate antibody responses against tumor-associated carbohydrate and peptide small antigens and peptide derived from Alzheimer’s pathological proteins, which are also known as weak immunogens. The rationale for this approach has been that to curb addiction, an aggressive immunization protocol may elicit a fast and robust anti-drug antibody response that would rapidly block the reinforcing effects of drugs from the initiation of treatment.
Future evaluation of new addiction vaccines may include screening of different vaccination schedules (e.g., longer time intervals between immunizations) with the goal of achieving long-term antibody and memory responses. Future studies may also test the challenging hypothesis of generating high-affinity swIg memory B cells that would recognize unconjugated drugs of abuse, so that exposure to drug would boost a protective drug-specific antibody response. Tracking the hapten-specific B cell population will provide data to support analysis of antibody quantity and quality. For instance, B cell analysis may be performed prior to immunization when antibodies are not yet present or shortly after immunization when antibody levels are below the detection threshold of current assays. Alternatively, B cells may be measured in subjects long after the antibody response waned to determine whether vaccine formulations or immunization protocols elicited long-lived memory B cells.
19.4 A Novel Method to Detect Antigen-Specific B Cells
In contrast to other components of the immune system (e.g., T cells), analysis of antigen-specific B cells has been limited by the lack of methods to detect very scarce B cells displaying BCR specific for a given antigen (i.e., antigen-specific B cells). Most studies relied on the use of transgenic mice carrying mutations in their BCR to track antigen-specific B cells. A recent technology allows detection and analysis of very low numbers of antigen-specific B cells present in the whole B cell repertoire (Pape et al. 2011). This method has high resolution permitting detection of antigen-specific B cells in a naïve or unimmunized mouse. In the original report, the authors tracked B cells specific for the fluorescent protein phycoerythrin (PE) in naïve and immunized mice (Pape et al. 2011). To isolate PE-specific B cells, the whole B cell repertoire from pooled lymph nodes and spleen was first incubated with PE, and then B cells bound to PE were isolated by means of anti-PE magnetic beads, using separation columns in a magnetic field (Pape et al. 2011). After this enrichment step, PE-specific B cells were identified by means of flow cytometry and further characterized for their surface markers. This analysis allows the counting of individual antigen-specific B cells and determines whether they are IgMhigh memory B cells, memory B cells carrying isotype-switched immunoglobulin such as IgG or IgA, GC B cells displaying the GL7 surface marker, or antibody-secreting plasma B cells displaying high levels of immunoglobulin. The use of fluorescent antigen-based magnetic enrichment paired to flow cytometry showed that within the ~2 × 108 nucleated cells from the spleen and lymph nodes in a naïve mouse, only ~20,000 B cells were specific for PE (Pape et al. 2011), ~7000 for allophycocyanin, ~4000 for chicken ovalbumin (OVA), and even fewer for the enzyme glucose-6-phosphate isomerase (GPI). This method to detect antigen-specific B cells in unimmunized subjects has now been applied to study B cell memory formation, autoimmunity, and development of addiction vaccines (Pape et al. 2011; Taylor et al. 2012b, c, 2014).
19.4.1 Analysis of Hapten-Specific B Cells
The fluorescent antigen-based magnetic enrichment strategy paired to flow cytometry analysis has been recently adapted to study hapten-specific B cells before and after immunization with vaccines against drugs of abuse. As described in detail in Sects. 19.4.2 and 19.4.3, this strategy consists of two consecutive steps: magnetic enrichment of hapten-specific B cells and multiparameter flow cytometry analysis of hapten-specific B cell subsets. This analysis can be easily performed in wild-type and genetically modified mice (Pravetoni et al. 2014; Taylor et al. 2014). Although strain differences must be taken in account for proper experimental design, this method is not strain- or species-specific because it depends exclusively on the ability of B cells to bind the hapten or antigen of interest and on the availability of flow cytometry reagents. For instance, it is not currently feasible to perform this analysis in rats because critical reagents to identify rat B cell surface markers are missing. However, this method has been currently applied to analyze human peripheral blood mononuclear cells (PBMC, Pravetoni, unpublished). The most complete set of antibodies for characterization of lymphocytes are directed toward mouse, human, and nonhuman primate markers.
19.4.2 Method I: Magnetic Enrichment of Hapten-Specific B Cells
First, peripheral lymph nodes, spleen, or blood samples are processed into a single-cell suspension that includes a fraction of the whole B cell repertoire. Then, B cells are incubated with the hapten of interest (Figure 19.3 shows an oxycodone-based hapten, 6OXY) conjugated to the red-phycoerythrin (PE) fluorescent protein and with a decoy reagent consisting of an additional fluorochrome, Alexa Fluor (AF) 647, conjugated directly to PE. Using anti-PE magnetic microbeads and magnetic columns, all B cells that recognized the hapten and PE are positively isolated (i.e., enrichment as shown in Fig. 19.3). Using flow cytometry, 6OXY-specific B cells are separated from B cells that recognize PE because the PE-AF conjugate fluoresces at a wavelength different than PE alone (λ, Fig. 19.3). Once 6OXY-specific B cells are identified (Fig. 19.3), they are further characterized by the use of antibodies specific for B cell markers conjugated to additional fluorochromes.
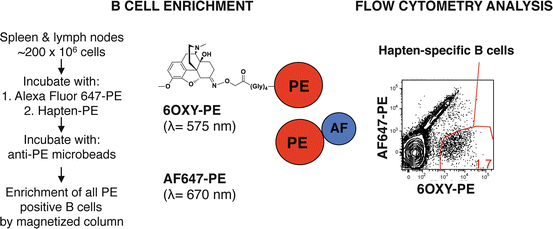
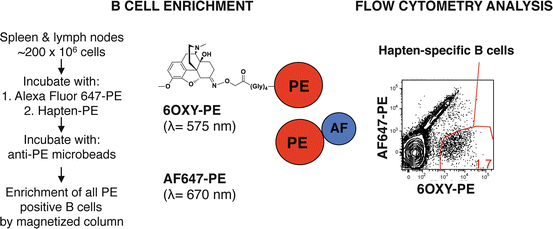
Fig. 19.3
Enrichment strategy to analyze hapten-specific B cells. The whole B cell repertoire, from individual mouse peripheral lymph nodes and spleen, is first incubated with a decoy reagent consisting of PE-AF647 followed by incubation with the hapten-PE (shown 6OXY-PE). All B cells binding the AF647-PE or the hapten-PE detection decoy reagent are positively isolated using anti-PE antibody-conjugated magnetic beads and magnetic columns. Using flow cytometry, it is possible to identify hapten-specific B cells from PE-specific B cells because the AF647-PE conjugate fluorescences at a different wavelength than PE
19.4.3 Method II: Multiparameter Flow Cytometry Analysis of Hapten-Specific B Cells
In order to perform analysis of antigen-specific B cells, a commercially available flow cytometer that permits multiparameter acquisition is needed. For instance, the procedures discussed in this chapter used a four-laser flow cytometer that allows analysis of up to 16 colors. After collection of raw data by flow cytometry, B cells are analyzed by standard commercial software (e.g., FlowJo, FlowJo LLC, Ashland, OR) using a specific analysis generally described as “gating strategy,” because it involves the application of flow cytometry gates to define specific B cell population characterized by specific sets of non-B and B cell markers as described below and shown in Fig. 19.4.
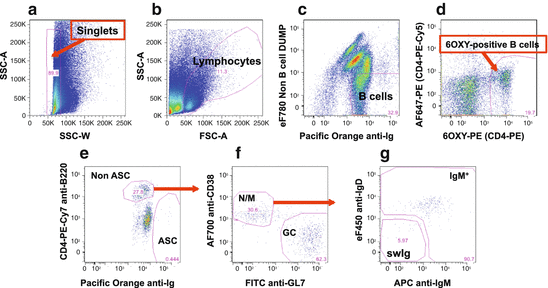
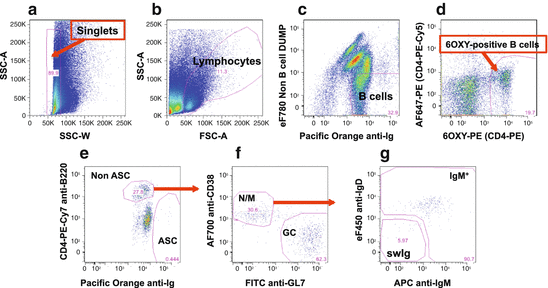
Fig. 19.4
B cell gating strategy of 6OXY-specific B cells in a representative dot plot from a mouse immunized with the 6OXY-KLH immunogen. (a) Singlet gating to remove any cells that have aggregated. (b) Total lymphocytes. (c) Total B cells expressing immunoglobulin (Ighigh) but not the following non-B cell markers: CD90.2 (T cells), Gr-1 (neutrophils), CD11.c (dendritic cells), and F4/80 (macrophages). (d) To differentiate between 6OXY-specific B cells from PE-binding B cells, B cells were further identified using the AF647-PE decoy. (e) Hapten-specific B cells were classified as either B220high non-ASC B cells or Ighigh ASC B cells. (f) Hapten-specific B220high non-ASC B cells were further identified as GL7high germinal center (GC) or CD38high naïve/memory (N/M). (g) Naïve/memory B cells were then identified as IgMhigh or IgMneg/IgDneg swIg B cells
First using a standard gating procedure (forward-scattered light, or FSC, vs side-scattered light, or SSC), single cells are separated from cell aggregates (see Fig. 19.4, panel a). Within the single-cell gate, lymphocytes are identified from residual non-lymphocytes or dead cells (Fig. 19.4, panel b). Once lymphocytes are identified, they are further identified as either B cells expressing intracellular immunoglobulin or non-B cells expressing surface markers for T cells (CD90.2), neutrophils (Gr-1), dendritic cells (CD11.c), and macrophages (F4/80) as shown in Fig. 19.4, panel c. At this stage, B cells that bound the 6OXY hapten or the PE carrier are differentiated based on the fluorescence of the decoy reagent AF647-PE, which fluorescences at a wavelength of 670 nm compared to the PE alone at 575 nm.
Within the population of B cells that bound the 6OXY hapten, actual 6OXY-specific B cells are identified as either non-antibody-secreting cells (non-ASC) expressing high levels of B220high or antibody-secreting cells (ASC) expressing high levels of intracellular immunoglobulin (Ighigh). The use of multiple gating and B cell markers ensure that analysis of B cells is accurate and does not include “contaminant or residual cells”. For instance, B220mid Igmid FC-binding cells may appear soon often immunization (Fig. 19.4, panel e), but they are not B cells (Bell and Gray 2003) and are not included in further analysis. Once B220high non-ASC 6OXY-specific B cells are identified, these cells are classified as CD38neg GL7high GC B cells or GL7neg CD38high naïve and memory B cells. Then, the CD38high B cell population is characterized as B cells expressing either high levels of IgM (IgMhigh B cells) or high-affinity swIg B cells (Pape et al. 2011; Taylor et al. 2014). Limited markers are available to differentiate between GL7neg CD38high naïve and memory B cells; therefore, it is critical to include in the experimental design both naïve and immunized mice so that naïve and memory B cells can be compared before and after immunization.
In general, when assessing the contribution of hapten-specific B cells to vaccine responses, it is important to include several controls in the experimental design. For instance, analysis of B cells should be performed in naïve mice or mice exposed to the vehicle or the adjuvant alone to determine background or baseline numbers of hapten-specific B cells. Analysis of vaccine-induced hapten-specific B cells should be performed in mice immunized with either the unconjugated carrier or the hapten-carrier conjugate to ensure that B cell responses are specific to the hapten. To further assess the specificity of this procedure, B cells are preincubated in the presence of the free drug, hapten, or conjugate to inhibit binding of the hapten-PE reagent (Taylor et al. 2014). Alternative negative controls may include magnetic enrichment of B cells in the presence of PE alone or in the absence of anti-PE microbeads.
19.5 Hapten-Specific B Cells Are Markers of Vaccine Efficacy Against Prescription Opioids
As described in Chapter 1, the prescription opioids oxycodone, hydrocodone, and oxymorphone are among the most commonly abused drugs in the USA (National Survey on Drug Use and Health: National Findings 2010; Oxymorphone abuse: a growing threat nationwide 2011; Epidemic: responding to America’s prescription drug abuse crisis 2011). The Center for Disease Control and Prevention estimated that ~2.1 million of people abused prescription opioids in 2012 and that prescription opioids accounted for ~17,000 overdose deaths (Wide-ranging OnLine Data for Epidemiologic Research (WONDER) 2014).
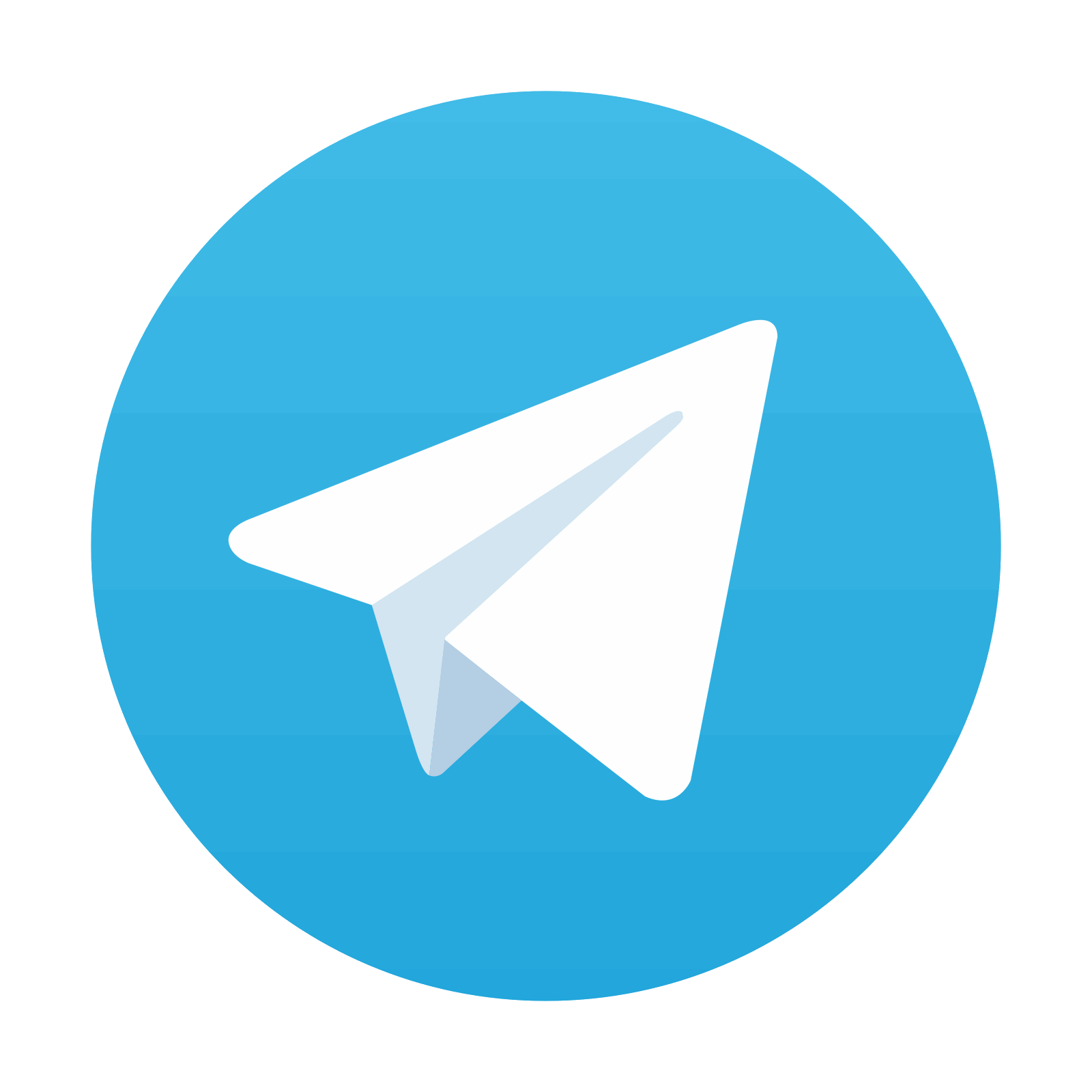
Stay updated, free articles. Join our Telegram channel

Full access? Get Clinical Tree
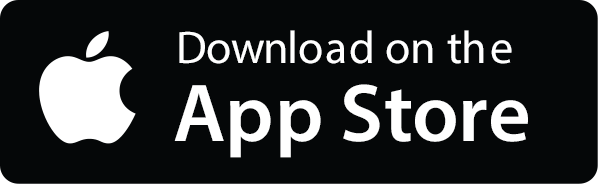
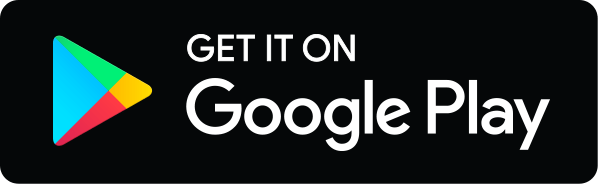