Aseptic Processing and Facilities for the Control of Microbial Contamination
James E. Akers Jr
From the earliest days of parenteral administration of health care products, there have been active ingredients that could not withstand a sterilization process, or in the parlance of the pharmaceutical industry, could not be terminally sterilized. Historically, these products were sufficiently heat labile, and moist heat, the principal method for end-product sterilization, could not be used.1 Advancements in sterilization technology, such as the discovery that radiation could be used to sterilize some heat labile products safely, increased the use of terminal processing. Currently, the majority of sterile parenteral preparations are still not able to withstand traditional sterilization in their final container. Surveys done in the 1990s reported that more than 80% of all sterile preparations were aseptically manufactured without terminal sterilization.2 Since those surveys were conducted, there has been an increasing prevalence of biological products that are incompatible with terminal sterilization technologies.
It is a widely held view in the health care industry that no manufacturing process is as technically challenging to accomplish and maintain as the aseptic processing production of sterile drug products. Aseptic manufacturing of sterile products without terminal sterilization requires the complex melding of several skills and technologies.3 These technologies typically include sterilization of all product contact packaging components and product contact manufacturing equipment. The most challenging technological requirement is an environment that can be designed and operated in a manner that renders it as free of contaminating microorganisms as possible. The challenges and risks of aseptic processing have been well described and have resulted in complex regulatory compliance requirements and standards.4,5,6,7,8
The recent emergence of new types of health care products derived from biotechnology has imposed an even more difficult process control challenge than that of traditional aseptic processing. In research and development as well as production, some biotechnology processes, such as genetic therapy, require not only that the product is sterile but also that potentially hazardous materials such as virus vectors are absent. Aseptic process controls with simultaneous containment of process generated hazards requires exacting process and facility design as well as operational controls.
Facilities used solely for the control of product microbiological quality do not require the same level of critical activities as those used for aseptic processing. In these types of operations, the systematic control of microbial contamination need not approach perfection, but instead, it must be good enough to protect adequately the process or product contained within the clean environment (see chapter 60). Whereas these facilities may be less technically demanding than those built for aseptic processing, they nevertheless require careful consideration regarding design and operation.

The term aseptic processing has been widely used in the health care industry to describe the manufacturing of sterile products without the benefit of terminal sterilization. In the science of microbiology, the word aseptic means the absence of microbial contaminant capable of causing sepsis. Literally, the most concise description of aseptic is “without infection.” Thus, aseptic has a meaning that is quite distinct from that of sterility, where aseptic implies the elimination of all organisms having the ability to reproduce. In the health care field, the essential meaning of sterility is that any microorganisms in or on a material must be nonviable.
It is not surprising that there has been a heated debate for decades regarding the labeling of products that cannot be terminally sterilized. Some industry scientists have held that aseptically manufactured products could not accurately be labeled sterile.1 In spite of the firmly
held opinions on both sides of this issue, the word sterile continues to be used to describe both products manufactured aseptically and using terminal sterilization. The regulatory application of the word sterile is not, it must be emphasized, a mere semantic distinction. Aseptic processing does not kill microorganisms, rather it functions to separate microorganisms from the product and prevent them from entering the product from the surrounding environment. Some microorganisms such as viruses are not always filterable and therefore may not be separable from the product stream. A further complication is that aseptic processing relies on environmental controls to prevent microorganisms from entering product containers, and attaining definitive proof of sterility in this aspect of manufacturing is dubious. So dubious in fact that it may be considered technically impossible both analytically and statistically.
held opinions on both sides of this issue, the word sterile continues to be used to describe both products manufactured aseptically and using terminal sterilization. The regulatory application of the word sterile is not, it must be emphasized, a mere semantic distinction. Aseptic processing does not kill microorganisms, rather it functions to separate microorganisms from the product and prevent them from entering the product from the surrounding environment. Some microorganisms such as viruses are not always filterable and therefore may not be separable from the product stream. A further complication is that aseptic processing relies on environmental controls to prevent microorganisms from entering product containers, and attaining definitive proof of sterility in this aspect of manufacturing is dubious. So dubious in fact that it may be considered technically impossible both analytically and statistically.
Terminally sterilized products are considered different regarding patient risk than those manufactured aseptically. This difference is attributed to the capability of terminal sterilization to inactivate microorganisms with an exceptionally high rate of success. Nevertheless, regulatory requirements make it necessary for industry to apply the label sterile to both terminally sterilized and aseptically processed products. At the same time, government regulators, industry experts, and standard setters acknowledge that the two methods for the manufacture of products labeled sterile are not entirely equivalent.
The microbiological analytical tools currently available lack the ability to distinguish between a product free of any viable organism and one that contains no potential infectivity. Even if assessment tools of the requisite limit of detection and sensitivity did exist, it would not be possible to assert with absolute certainty that an aseptic product was always sterile. There are organisms that are known pathogens, but others are opportunistic pathogens that can cause disease rarely and only in a subset of patients. Given the fact that many product recipients are to one extent or another unwell, or even immunocompromised, it is difficult to ascribe complete harmlessness to any organism. The pharmacological and chemical characteristics of products further complicate risk assessment. Many products are either inherently antimicrobial or contain antimicrobials in the form of preservatives (see chapters 39 and 40). Furthermore, there is no method given in the current international regulations or guidelines applicable to aseptic manufacturing for assessing the effect the product or its preservative system has on reducing the risk of microbial contamination. Although we do not formally consider the antimicrobial factors possessed by a product in any comprehensive way, the antimicrobial effect might reduce the risk of patient infection.
The only means currently used for directly evaluating the capability of an aseptic process is the process simulation or media fill test. This test is the filling and subsequent incubation of actual product containers with microbiological media under realistic simulated production conditions. The object of the media fill or process simulation test is to assess the ability of the process to manufacture uncontaminated (aseptic) product. The media fill test has been required in industrial aseptic manufacturing for about 40 years.9 Because the media fill test is used universally in industry, there exists an understanding of the facility and process conditions resulting in low rates of contamination.
Microbiological assessment of the facility environment, known as environmental monitoring (EM) is given critical importance by many practitioners and many regulatory authorities (see chapter 60). The principle technical limitation of EM is that it is only an indirect measurement of microbiological controls. The EM provides a means of assessing facility hygiene in general terms rather than a specific assessment of sterility assurance. The EM is not a suitable method for accurately determining patient risk.10 The value of EM is that it allows us to understand the factors in facility design and operation that consistently result in low microbiological and particulate contamination.
The antimicrobial effect produced by any preservative system present or by the nature of the product itself is by convention not considered in determining a patient safety factor for an aseptically filled product. The inherent antimicrobial factor(s) possessed by the formulation can abate hazards associated with microbial contamination. This risk abatement is excluded from the determination of contamination rate tabulated from media fill testing. It is possible in every case to estimate the antimicrobial effect of a formulation. This is a critical element of what is broadly known as product characterization that should not be excluded from patient risk analysis. The exclusion of antimicrobial risk abatement factors that are part of many product formulations when considering sterility assurance is unfortunate. We do not possess a means for objective quantitative assessment of the attribute of “sterility” in aseptic processing, and the substitution of nonquantitative subjective or even intuitive factors should be discouraged.11
The aseptic manufacture of products requires not only the ability to use a variety of contamination control technologies in concert with one another but also a high level of engineering, supervisory, and risk-assessment skills. Our forebearers in the health care industry were both wise and intellectually honest when they used the word aseptic to describe parenteral products that could not withstand terminal sterilization. It is challenging, even with 21st century technology, to manufacture products in a clean, aseptic environment. But we have learned from industrial aseptic processing experience that it is possible in the current state of practice to manufacture products that provide superb patient safety.12,13
Although the safety of products can be extremely high, aseptic processing manufacturing methods, at the current state of the art, can lack precision and sensitive
safety assessment tools. The sterility test has been required for product-release testing for sterile products for decades (see chapter 64); however, this test lacks the sensitivity to prove reliably that a product is pathogen or microbe free.14 Process simulation tests or media fills are important tools for proving process capability, and these tests have quite rightly taken on increasing importance over the years. It is important to know that the broad-spectrum microbiological medium typically used for these studies is not able to recover or grow all types of microbes that could be present in a product. Although the media fill is the most comprehensive evaluative tool available in aseptic processing, it is both statistically and microbiologically limited. Finally, the EM program, although it is useful for assessing background contamination in the manufacturing environment, does not directly assess the product. Additionally, we must recognize that any microbial test that relies on humans to conduct the test can and most likely will yield false-positive results.
safety assessment tools. The sterility test has been required for product-release testing for sterile products for decades (see chapter 64); however, this test lacks the sensitivity to prove reliably that a product is pathogen or microbe free.14 Process simulation tests or media fills are important tools for proving process capability, and these tests have quite rightly taken on increasing importance over the years. It is important to know that the broad-spectrum microbiological medium typically used for these studies is not able to recover or grow all types of microbes that could be present in a product. Although the media fill is the most comprehensive evaluative tool available in aseptic processing, it is both statistically and microbiologically limited. Finally, the EM program, although it is useful for assessing background contamination in the manufacturing environment, does not directly assess the product. Additionally, we must recognize that any microbial test that relies on humans to conduct the test can and most likely will yield false-positive results.
The best approach to the aseptic manufacture of health care products is a strong proactive one focused on contamination control and prevention rather than on measurement. Once the limitations of measurement-based strategies are acknowledged, the importance of proper design and operation of the aseptic environment and the process becomes clear. It can be stated accurately that the most important element in aseptic product safety is a process and environmental design that eliminates the potential for hazards. Fortunately, a great deal is known about the factors that introduce hazards into an aseptic process. It has been widely acknowledged since at least the late 1970s that the major causes of microbiological contamination in an aseptic processing clean room are the human beings working in those areas. In fact, human operators represent nearly all the contamination risk in aseptic manufacturing and are the only potential vector for pathogenic organisms in a clean room. Therefore, an obvious and generally quite successful approach to contamination control in the clean room is simply the elimination of personnel.15 Fortuitously, advanced automated equipment has provided the health care industry with an outstanding means of contamination control.16
It is also well accepted that contamination hazards are reduced by reducing the length of time sterile objects are exposed to the environment during aseptic manufacture.4,17 Therefore, equipment that can operate at high throughput smoothly and without human intervention is far safer than equipment that requires numerous interventions (or is manual) and operates at a low speed. For these reasons, equipment selection is an important factor in reducing hazards, which relate entirely to controlling contamination. High-speed, reliable, automated equipment that requires little human intervention for assembly, running adjustments, in-process maintenance, component charging, or quality-control sampling is far safer than equipment that is less able to run without operator intervention.18 Thus, equipment selection is an important factor in controlling contamination.
Another vital factor in reducing hazards associated with microbial contamination in aseptic manufacturing environments is the separation of hazards from the critical zone19 of the process that area within the aseptic environment where product, containers, and closures are brought together and assembled aseptically. It follows, given that operators are the most significant risk factor, anything that can be done to separate the operators from the critical zone will be an important control measure. In the 1970s, this separation typically was achieved primarily by the placement of flexible polyvinyl chloride (PVC) sheets. These sheets or curtains were an easily defeated barrier but nevertheless provided at least a psychological reinforcement of need for operators to keep their distance from the critical zone. A barrier in this case refers to any device that limits opportunity for human-derived or production-associated contamination to affect the quality of a product. Unfortunately, most process equipment available during that era required consistent and, in some cases, extensive human intervention. By the 1980s, equipment speed and reliability had increased to the point that less permeable fixed barriers could be used. Equipment manufacturers began to offer equipment that was enclosed in typically clear, solid polycarbonate panels. Equipment with these enclosures is still widely used in human scale clean rooms. Although these solid enclosures are less permeable than PVC sheets, the panels typically are mounted on hinges, which allows operator access for adjustment, maintenance, or sampling. As sophistication and automation of the equipment increased, light barriers or interlocks were added to stop moving parts when the panels were opened. In the mid-1980s, isolation technology was first used in the health care industry.20 Isolators can be defined as self-contained clean facilities and offer optimal control of human contamination because human operators work outside the clean zone. Isolators had been widely used in other industries since the 1960s, but they were used more for the containment of poisonous or otherwise hazardous materials than for the control of microbial contamination. Scientists and engineers recognized that a technology that was well suited to keeping contamination in, if properly employed, could be equally good at keeping microbial contamination out.
The devices the pharmaceutical industry calls isolators have been very widely adopted in the production of aseptic foods and beverages, where they are known as aseptic chambers. In the aseptic food and beverage industry, aseptic chambers have become universal in their application. These aseptic chambers often operate at throughput rates in the range of 600 to 1300 units per minute, so not only is the environment effectively controlled but the length of time that each unit produced is exposed to the environment is also extremely short.21 Isolators and the entire field
of isolation technology (aseptic chambers) have evolved considerably since their introduction to aseptic manufacturing industries and now represent the pinnacle of aseptic processing safety. Isolators, automation, robotics, and sensor technology will continue to advance, assuring a future in which human involvement in aseptic processing is limited to process supervision and review of processing data. The less complete barrier production operations sometimes called open restricted access barrier systems (RABS), which accommodate the possibility of human intervention, will in this author’s view become extinct along with all other manned clean rooms. The most likely trajectory for aseptic processing is the implementation of complete automation, where the nature of the enclosure will need to allow access during maintenance and changeover only, and never during routine aseptic operations. There is a species of separative technology used on the pharmaceutical and biopharmaceutical industries that is very similar to the aseptic chamber or isolator in performance. These are the so-called closed RABS systems, which are kept closed in operation and do not accommodate any direct human interventions during aseptic manufacturing. These closed systems were built to circumvent the decontamination processes generally required by regulatory agencies for isolator systems or aseptic barriers. In this author’s view, both closed and open RABs will face extinction because automation technology will render them unnecessary. Evidence of this can be found in the aseptic food and beverage industry where a RABS alternative has not evolved and human scale or manned clean rooms are already extinct.21
of isolation technology (aseptic chambers) have evolved considerably since their introduction to aseptic manufacturing industries and now represent the pinnacle of aseptic processing safety. Isolators, automation, robotics, and sensor technology will continue to advance, assuring a future in which human involvement in aseptic processing is limited to process supervision and review of processing data. The less complete barrier production operations sometimes called open restricted access barrier systems (RABS), which accommodate the possibility of human intervention, will in this author’s view become extinct along with all other manned clean rooms. The most likely trajectory for aseptic processing is the implementation of complete automation, where the nature of the enclosure will need to allow access during maintenance and changeover only, and never during routine aseptic operations. There is a species of separative technology used on the pharmaceutical and biopharmaceutical industries that is very similar to the aseptic chamber or isolator in performance. These are the so-called closed RABS systems, which are kept closed in operation and do not accommodate any direct human interventions during aseptic manufacturing. These closed systems were built to circumvent the decontamination processes generally required by regulatory agencies for isolator systems or aseptic barriers. In this author’s view, both closed and open RABs will face extinction because automation technology will render them unnecessary. Evidence of this can be found in the aseptic food and beverage industry where a RABS alternative has not evolved and human scale or manned clean rooms are already extinct.21

Facility Design Attributes
The first step in a facility planning exercise should be a detailed analysis of microbial hazards as they apply to the production process. A formal, documented method of hazard analysis is the most effective method for identifying and assessing hazards and can be extremely useful in determining what level of controls should be applied for each step in the manufacturing process. Hazard analysis critical control point (HACCP) planning is widely used to assess risks to a process, and environmental- or facility-related hazards should be included as components of a thorough HACCP program. The HACCP planning is a general regulatory requirement in food manufacturing and is being applied more broadly in health care manufacturing processes as well. An HACCP team for an aseptic process may overlap in membership with a facility planning team. The HACCP methods and program organization are beyond the scope of this chapter; however, the reader is urged to research these methods independently.22
There are other risk management systems that may be applied that suggest other statistical or computation means for conducting risk assessment. These include systems that consider intervention risk or use elements of a failure mode effect analysis approach. In the author’s view, risk analysis systems reliant on EM data alone as a metric are inherently suspect. This is because EM results in well controlled aseptic processing manufacturing environments typically show the absence of viable microorganisms. It is necessary to consider that the absence of growth, or zero (0), in microbiological plate cultivation methods has no clear meaning beyond the obvious conclusion that nothing grew (see chapter 64). It is not uncommon for a finding of no growth to be interpreted as a “sterile” outcome. This is analytically incorrect as it assumes that any organism that might be present would grow in the medium/media and growth conditions used in testing. It is well known that the efficiency of this type of testing for detection and quantitation is limited.23
Facilities that require human scale clean rooms for aseptic processing are often expensive to build and to operate. At the same time, manned clean room facilities for less than fully aseptic levels of control contamination can be relatively simple and contain only minimal environmental control features. In facilities where separative technologies are employed, such as with isolation technology, a simpler surrounding clean room environment can suffice. Facilities also may need to be designed to protect the worker from hazards associated with pathogenic organisms or chemical contaminants used for research, development, or manufacturing. Depending on the nature of the process, these facilities also may be required to protect the manufacturing process and the product from contamination with other microorganisms. Facilities that must provide both chemical and/or biological containment for the operator or environmental protection as well as microbial control for process hazard reduction, impose unique design constraints. Those that require a blend of hazardous material containment and microbiological control are among the costliest to install, test, and operate. But if isolators can be used, a combination of aseptic safety and containment of hazards can be accomplished optimally and at with minimal cost implications.
Facilities can be designed and built to accomplish varying degrees of control or containment, depending on the process and a variety of regulatory requirements. Thorough, rigorous planning is absolutely necessary to ensure that the best cost-to-performance ratio is achieved.24 Among the critical issues to be addressed in the design of a facility are
Process layout
Personnel access and personnel load
Location of the manufacturing process within a multipurpose building
Relationship, if any, with other work areas within the building
Relationships and the potential effect on other operations located on the same property or group of buildings
Current regulatory requirements including
Product regulatory requirements (eg, US Food and Drug Administration)
Environmental/civil engineering issues, including sewage discharge, safety, emergency planning
Occupational health and safety and others as applicable (eg, radiation safety, live organism control)
Likely changes in regulations or the need to comply with international regulations (It is vital to consider in any new facility the potential for increasing stringency in regulatory requirements as they evolve over time.)
Flexibility of design if the facility is converted to other uses or if other processes are likely to be added in the future
Emergency management
Facility Classification
Over the years, ventilation supply air quality classifications have evolved for facilities designed to control contamination. Probably the first official classification scheme to be used on a broad industrial scale was the US Federal Standard 209 entitled Airborne Particulate Cleanliness Classes in Cleanrooms and Clean Zones.25 This standard described the general design and particulate air quality performance requirements for facilities commonly called clean rooms. It emerged in the early 1970s with the iteration designated FS209B and remained the gold standard of classification until the global adoption of International Organization for Standardization (ISO) 14644 in 1999.26,27 The final iteration of FS209 (designated version E), even though was retired with the adaptation of ISO 14644, can still be read and referenced to the original clean room classification standard at the present time.
Technically, FS209E was a legal requirement only for organizations doing work for the United States government. Thus, FS209E was officially allied to products, manufactured in classified clean rooms or clean zones, that were purchased by the United States. In broad practice, FS209E became by far the most important general reference in the classification of clean rooms in its time. Many of the clean room operational parameters delineated initially in FS209 have become general design and control specifications that have been captured in one way or another in a variety of industrial and regulatory guidelines. The British, French, German, and Japanese governments, as well as others, also introduced clean room performance standards. The European Union (EU, then European Economic Community) incorporated a classification scheme in their good manufacturing practices document first issued in 1989. Although EU Annex 1 has been revised several times since its clean room grades were introduced and is currently under revision again, the fundamental requirements assigned to each grade have not changed.8 Tables 58.1 and 58.2 show internationally referenced room classification schemes commonly used in aseptic processing. Although these standards differ in units of measure and in some cases particulate size specified for classification, experience has proven that these clean room standards generally resulted in demonstrably equivalent levels of facility performance.
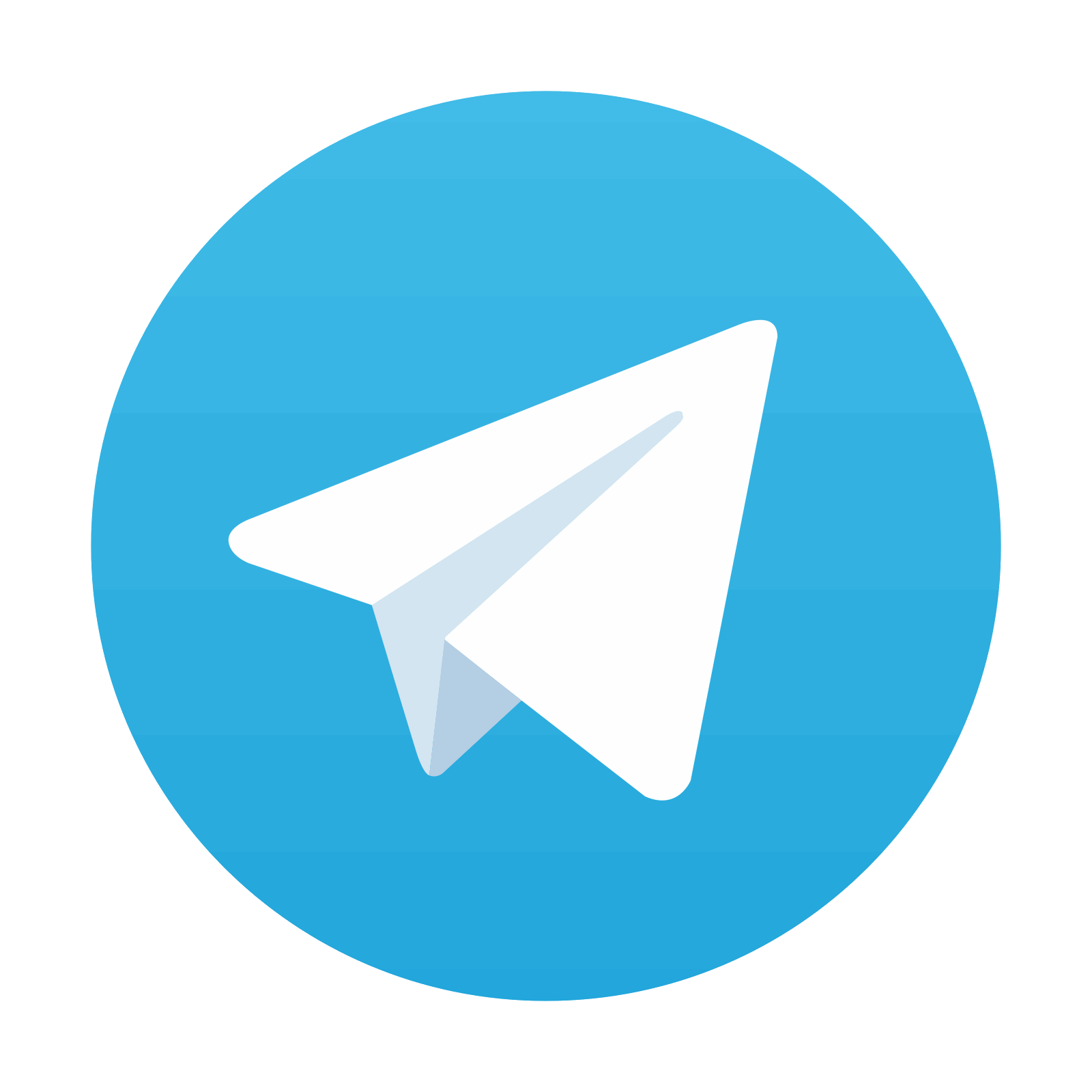
Stay updated, free articles. Join our Telegram channel

Full access? Get Clinical Tree
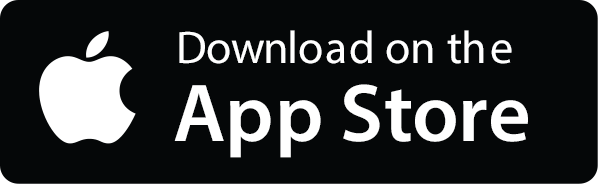
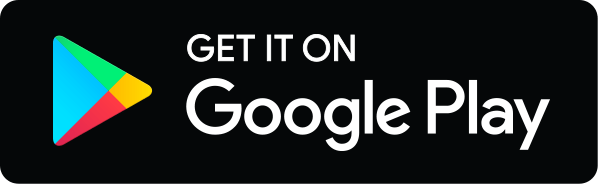