Group
A415
A476
Control 24 h
0.044 ± 0.001
0.042 ± 0.004
ART 24 h
0.145 ± 0.002*
0.110 ± 0.003**
ART 48 h
0.033 ± 0.001*
0.123 ± 0.002**
ART 96 h
0.049 ± 0.002*
0.131 ± 0.002**
3.2.2.2 ART’s Threshold Effect and Possible ART-Targeted Enzymes
In the present investigation, we evaluated the effect of different concentrations of ART on the in vitro propagation of HepG2 after incubation for 48 h. It was found that the survival rate of tumor cells is highly dependent upon ART’s concentrations, in which 50 μM ART leads to a higher survival rate accounting for nearly 80 %, whereas 100 and 200 μM ART cause the dramatic cell death, with a survival rate of 40 % for 100 μM ART and of 25 % for 200 μM ART. These results indicated that there exists a threshold effect of ART on the survival/death fate of tumor cells.
Considering that NOS is a hemoprotein and ART enables the alkylation of hemoproteins, we envisaged that ART in an optimal concentration might conjugate the heme-containing NOS to inhibit its activity, and also induce its expression in a feedback manner. Therefore, we should verify an elevation of NO levels after incubation of HepG2 with a sublethal dose of ART. For this purpose, we incubated HepG2 with 50 μM ART for measuring the NO level. As our expectation, a dramatic elevation of the NO level was observed up to 35 μM after incubation for 48 h. In contrast, NO was not detected in HepG2 treated by 100 or 200 μM ART. These results indicated that 50 μM ART can trigger a protective level of NO from tumor cells, but 100 and 200 μM represent the lethal doses of ART.
Because CAT that scavenges H2O2 is also a hemoprotein, we measured the H2O2 level after incubating HepG2 with 50, 100, or 200 μM ART for 48 h. However, neither low-dose nor high-dose ART results in a significant change of H2O2. The level of H2O2 in untreated and 50 μM ART-treated tumor cells is equal to 53 mM, and that in 100 or 200 μM ART-treated tumor cells is approximately 57 mM. These results suggested that CAT is unlikely implicated in tumor killing by ART, and H2O2-mediated apoptosis may not be a major mechanism by which ART exerts an antitumor effect.
3.2.2.3 Synergistic Effects Through the Combination of ART with Chemotherapeutics
Although high doses of ART (100–200 μM) are effective on tumor killing, it is apparently higher than ART’s IC50 (70 μM), thereby endowing a nonselective toxicity to normal cells. However, 50 μM ART not only fails to kill tumor cells, but also promotes tumor growth. To obtain a higher antitumor effect using a lower dose of ART, we combined 50 μM ART with 10 μM 5-fluorouracil (FLU) for in vitro antitumor evaluations in HepG2 cells. Consequently, ART exhibits a significant synergistic effect to FLU, in which tumor growth is inhibited by 25–40 %. In contrary, tumor cells treated by 10 μM FLU alone show an inhibition rate of only 8 %. These results suggested that a sublethal dose of ART could effectively kill tumor cells as it is combined with FLU.
It was observed that ART + FLU can dramatically decrease the NO level in HepG2, from 16 μM NO in 50 μM ART-treated tumor cells to 11 μM NO in 50 μM ART + 10 μM FLU-treated tumor cells. At the same time, no significant change in H2O2 levels was found in tumor cells incubated with ART alone or ART + FLU. Therefore, ART may not stimulate H2O2 for augmenting FLU’s antitumor activity.
3.2.3 Discussion
It has been reported that approximately 5–18 % of ART can bind to hemoproteins such as hemoglobin, but does not react with heme-free globins (Meshnick et al. 1991). Among versatile hemoproteins, ART’s targets for exerting antitumor activity are currently unidentified. Because NOS was previously proved a cytochrome P450-like hemoprotein (Chinje and Stratford 1991), we planed to examine whether the formation of ART-heme adducts might be correlated with the elevation of NO levels as well as whether they would accompany with the elevation or decline of survival rates in HepG2.
If this is the case, ART conjugation to NOS should lead to its inhibition and induction. Indeed, we detected a dramatic increase of A415 following the exposure of HepG2 to ART upon incubation for 24 h, suggesting the de novo biosynthesis of hemoproteins including NOS. As indicated earlier, NO is able to inhibit the progressions of cysteine reduction and Fenton reaction (Gusarov and Nudler 2005). Therefore, NO is likely exploited by bacteria to protect from oxidative damage, and NO confers bacteria resistance to antibiotics (Gusarov et al. 2009).
With equal importance, it has been filed that NO can regulate cancer growth, migration, invasion, survival, angiogenesis, and metastasis in a concentration-dependent manner (Ridnour et al. 2006; Jang and Kim 2002). Evidence supporting a beneficial role of NO to tumor survival comes from a finding that NO levels are higher in cancerous tissues compared to their normal counterparts (Wink et al. 1993). This finding was later deciphered as that NO is harnessed to protect against cellular damage and cytotoxicity from ROS and organic peroxides (Wink et al. 1995).
Our results indicated that a lower concentration (50 μM) of ART allows tumor cells survival with a higher rate, whereas higher concentrations (100–200 μM) of ART cause tumor cell death. Interestingly, 50 μM ART triggers NO burst, but 100–200 μM ART does not. Therefore, it can be deduced that ART-induced protective NO should benefit to tumor cell survival. This conclusion is supported by a previous observation that the cytotoxicity of ART in the mouse macrophage cell line RAW 264.7 is associated with the inhibition of NOS (Konkimalla et al. 2008). Additionally, we did not detect any significant changes of H2O2 levels after ART treatment although CAT is also a hemoprotein. This is likely because ART only inhibits the heme-harboring CAT, but does not inhibit the heme-independent GSH-POX, which can detoxify H2O2 and may maintain a homeostatic redox state.
In summary, a low dose of ART does not exert an antitumor role because it induces the production of a protective NO level. In contrast, a high dose of ART exhibits a potent antitumor effect by its direct cytotoxicity.
3.2.4 Conclusions
ART kills cancer cells with uncertain mechanisms. Here, we report that ART can exert its antitumor activity by alkylating hemoproteins such as NOS in tumor cells. The conjugation of ART with the heme moiety of hemoproteins was evident by monitoring the shift of absorbance from heme (A415) to ART-heme adducts (A476). Accordingly, it was observed that a transient elevation of A415 is accompanied with a synchronous burst of NO and a higher rate of survival following incubation of tumor cells with 50 μM ART. In contrast, ART at above 100 μM leads to a lower NO level along with a lower survival rate, strongly implying that NOS may represent an important target of ART for killing tumor cells. Although CAT as a hemoprotein also interacts with ART, no remarkable fluctuation of CAT activity was observed after the incubation of tumor cells with 50, 100, or 200 μM ART for 48 h, suggesting that ART kills tumor cells unlikely through the H2O2-mediated apoptosis mechanism. Furthermore, we observed that a combination of ART with FLU exhibits a synergistic effect in killing tumor cells, perhaps by abrogating the NO-conferred cytoprotective role. These results should pave an avenue toward the eventual application of ART in clinic antitumor treatment.
3.3 Pro-oxidant Agents Synergize ART in Killing Tumor Cells
3.3.1 Purposes and Significance
Although ART exhibits antitumor activity at some extent, it cannot be comparative to any conventional chemotherapeutics because of its relative lower cytotoxicity. Nevertheless, ART has a potential of killing tumor cells with resistance to common antitumor drugs. The suggested mechanisms of ART combating tumor cells include: (1) antiproliferation and antiangiogenesis; (2) apoptosis; (3) oxidative stress; (4) oncogenes and tumor suppressor genes; and (5) multidrug resistance (Efferth 2006).
Given ART exerting a tumor-killing role via its endoperoxide, any compounds that stabilize the unique structure should serve as synergists, whereas any compounds that destabilize the unique structure might function as antagonists. In other words, a milieu tending to oxidation should enhance ART’s antitumor activity, whereas a milieu beneficial to antioxidation should suppress ART’s antitumor activity. In fact, the IC50 of ART was observed to correlate with the expression levels of 170 genes involved in the oxidative stress response and metabolism in 60 cell lines (Efferth and Oesch 2004).
To test whether oxidative stress benefits ART to exert more potent antitumor effects, we combined ART with pro-oxidants to constitute a drug combination, known as “ART-sensitizing compounds” (ASC), for antitumor evaluations in vitro in the hepatoma cell line HepG2 and in vivo in tumor-bearing nude mice. In addition to ART per se, ASC include three pro-oxidants: the GSH exhauster diethyl maleate (DM); the GSH-POX inhibitor mercaptosuccinic acid (MA); and the CAT inhibitor aminotriazole (AT).
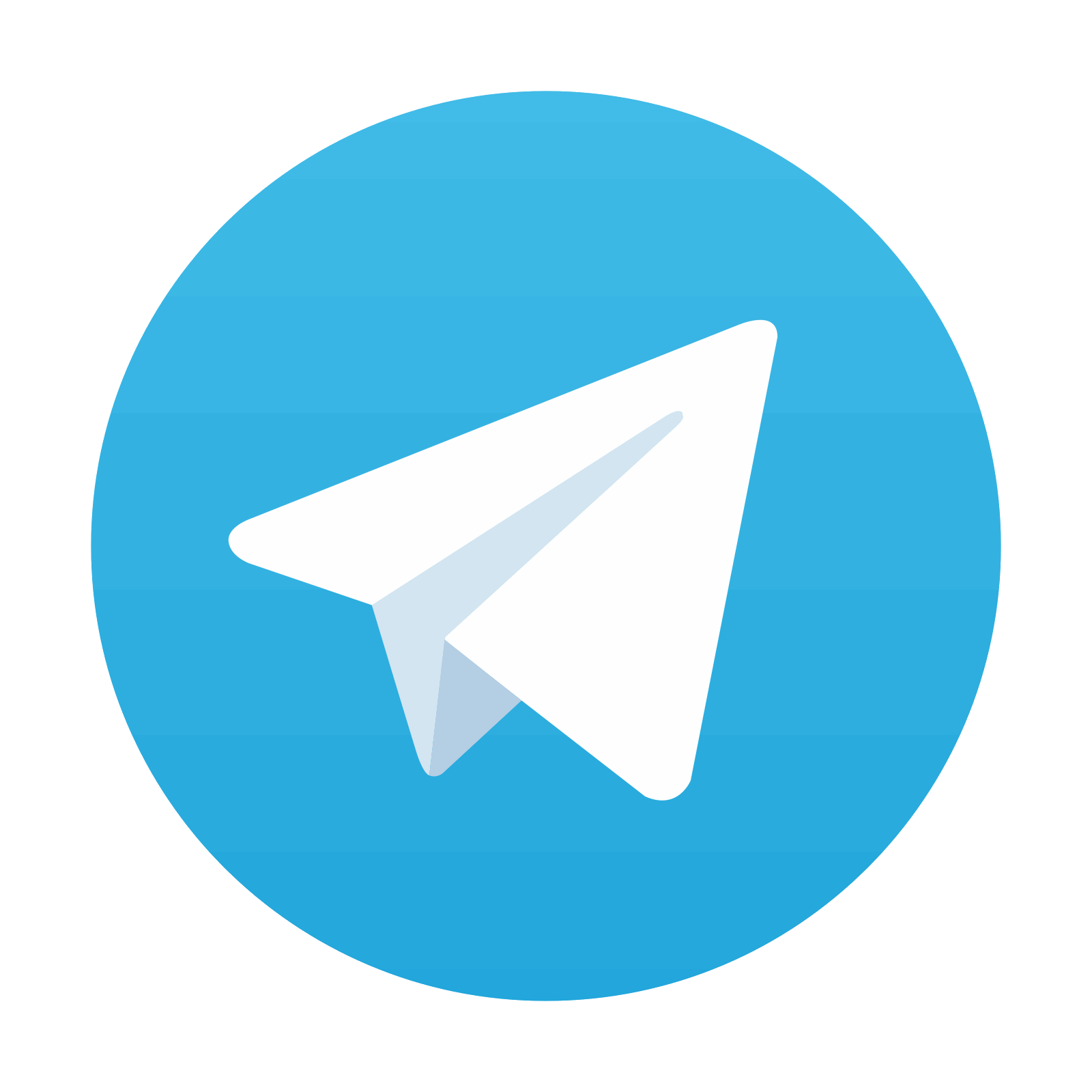
Stay updated, free articles. Join our Telegram channel

Full access? Get Clinical Tree
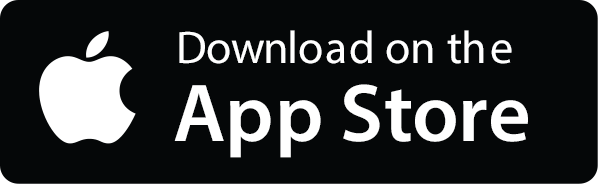
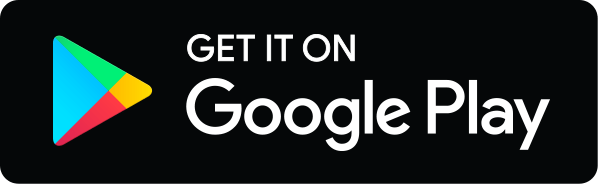