Fig. 4.1
Induction of NO production in B. licheniformis upon acclimatization to oxidative stress and suppression of oxidative stress-inducible NO. ART artemisinin; l–NMMA NG-monomethyl-l-arginine monoacetate; NO nitric oxide. Double asterisks (**) represent very significant difference from the control (P < 0.01); Double pound signs (##) represent very significant difference from the hypoxia + cold group (P < 0.01)
These results indicated that bacteria can synthesize NO for adaptation to oxidative stress circumstance, and ART can inhibit the synthesis of NO in bacteria, hence representing a novel inhibitor of all kinds of NOS including bNOS. Thus, we could expect that ART would sensitize those antibiotics that pose oxidative stress to bNOS-dependent bacteria.
4.2.2.2 Attenuation of NO-Conferred Antibiotic Tolerance by ART: In Vitro Study
We first tested whether bacterial NO burst under a hypoxic condition confers B. licheniformis tolerance to RIF. After cultured for 12 h with RIF (20, 40, 60, or 120 μg/ml), hypoxia-acclimatized bacteria proliferate at a higher rate, whereas nonacclimatized bacteria exhibit no proliferation. Similarly, hypoxia-acclimatized bacteria also show accelerated proliferation in the presence of cefotaxime (CEF) (15, 25, or 50 μg/ml). These results demonstrated that hypoxia-induced NO allows bacteria to thrive in exposure to RIF/CEF, suggesting that NO confers B. licheniformis tolerance to those antibiotics.
By attenuating the protective NO production in bacteria, ART was anticipated to reverse NO-mediated protection of bacteria from antibiotics. Indeed, a combination of ART (60 μg/ml) with RIF (20–120 μg/ml) leads to a lower rate of bacterial growth than RIF alone, implying that ART potentates RIF by abrogating bacterial NO production. We observed that enhanced NO burst, at a maximal level of 80 μM, occurs in bacteria in exposure to RIF (20–120 μg/ml), whereas a decreased NO level (20 μM) or even a negligible NO level was measured in bacteria cotreated by ART (60 μg/ml) with RIF (20–120 μg/ml).
As comparison, we also detected the NO level in bNOS-free E. coli, either with or without CEF, but no correlation of bacterial proliferation with NO production was established. The growth dynamics of E. coli under CEF (100 μg/ml) are similar with those under ART (60 μg/ml) + CEF (100 μg/ml). The maximal NO levels are only 4–8 μM under CEF (100 μg/ml) or ART (60 μg/ml) + CEF (100 μg/ml). From the extremely low level of NO in E. coli, we could infer that its own NO may not be sufficient to detoxify antibiotics.
4.2.2.3 Bacterial Production of H2O2 Due to CAT Inhibition by ART
As an antioxidant hemoprotein, CAT was expected to interact with ART and to exhibit a lower enzymatic activity. If this is true, we could deduce that there should be a similar mechanism by which ART binds to CAT and blocks the conversion of toxic H2O2 to nontoxic H2O or other detoxified compounds. To confirm this deduction, we measured the CAT activity of B. licheniformis after incubation with RIF (20–120 μg/ml) or RIF (20–120 μg/ml) + ART (60 μg/ml). The results showed that CAT activity is considerably reduced once ART was included in the culture (Fig. 4.2). For example, after 24 h, CAT activity in bacteria treated by RIF (20 μg/ml) is as threefold as that in bacteria treated by RIF (20 μg/ml) + ART (60 μg/ml).


Fig. 4.2
CAT activity in B. Licheniformis upon exposure to RIF or ART + RIF. ART artemisinin; Rif rifampicin. A single asterisk (*) represents significant difference in ART + RIF from RIF (P < 0.05); Double asterisks (**) represent very significant difference in ART + RIF from RIF (P < 0.01)
It means that enhanced H2O2 production following CAT inhibition by ART should facilitate the bactericidal effect of antibiotics. Therefore, ART can abrogate the protective NO, on the one hand, and also promote the harmful H2O2, on the other hand, thereby exhibiting a dual synergistic effect as the inhibitors of both bNOS and CAT.
4.2.2.4 Identification of ART-Bacterial Heme Conjugates
Based on our assumption, ART would bind to bNOS, CAT, and other hemoproteins, hence prohibiting the interconversion between Fe3+ and Fe2+. We monitored the dynamic fluctuations of heme and ART-heme conjugates. As results, A 415 that reflects the absorbance of heme and A 476 that represents the absorbance of ART-heme conjugates were observed to have higher readings (A 415 = 0.3; A 476 = 0.2) after adding ART for three hours. As comparison, the lower readings (A 415 = 0.18; A 476 = 0.06) were determined in bacterial cultures without ART supplementation. After elevations by three-hour incubation, both A 415 and A 476 decline to readings equal to the control by incubation for 6–9 h.
The elevation of A 415 was assumed to the de novo biogenesis of hemoproteins, while the elevation of A 476 could be a hint indicating the formation of ART-heme conjugates. The increases of hemoproteins including bNOS and CAT need the overexpression of corresponding genes, because they should be inducible after the inactivation of those enzymes due to heme alkylation by ART. The double decline of A 415 and A 476 is likely attributed to bacterial death upon ART’s bactericidal effects.
4.2.3 Discussion
The nonpathogenic B. subtilis and pathogenic B. anthracis as well as other G+ bacteria generate NO by their own bNOS that contains a prosthetic heme moiety for the reduction of a catalytic center from [Fe3+] to [Fe2+] (Gusarov et al. 2008). Our present research showed that B. licheniformis can accumulate nitrate and nitrite, the oxidized products of NO, in the media under oxidative stress conditions including hypoxia and hypoxia + cold. This finding is supported by a previous report describing that NO is not consumed and can accumulate in the microenvironment of human tissue at lower O2 concentrations (Taylor and Moncada 2010). Furthermore, we also found that bacterial NO production can be repressed by ART or l-NMMA, suggesting that bNOS is involved in the oxidative stress-induced NO production.
Although E. coli strains generating NO are independent on bNOS, they have an enzyme complex encompassing nitrite reductase, flavohemoglobin, and NO-sensing regulator responsible for NO production (Corker and Poole 2003). Indeed, we observed an elevation of NO levels in E. coli overnight cultures. The NO level, either in the presence or absence of ART or ART + CEF, was detectable, but relatively low (below 12 μM), much lower than that in B. licheniformis (80–120 μM), suggesting that such a low NO level in E. coli is insufficient to protect bacteria from antibiotic attacking. ART may be directly cytotoxic to bacteria because ART can be converted to carbon-centered free radicals in vivo. The inhibition of a bacterial multidrug efflux pump system, of course, should represent an alternative mechanism of ART sensitizing antibiotics in E. coli (Li et al. 2011).
Given that heme alkylation by ART has been verified by identifying the ART-heme adducts in malaria-infected mice (Robert et al. 2005), and considering that an ART-heme interaction has been also confirmed in tumor cells (Zhang and Gerhard 2009), we proposed that ART should also interact with bacterial hemoproteins. In addition to bNOS, CAT is also a hemoprotein in Bacilus bacteria (Bol and Yasbin 1994). In the present study, we did detect the presence of ART-heme conjugates in B. licheniformis after incubation with ART for three hours. Later on, however, ART-heme conjugates cannot be detected, probably because of the degradation of conjugates following bacterial death. This situation is similar with our observation in tumor cells (Zeng and Zhang 2011).
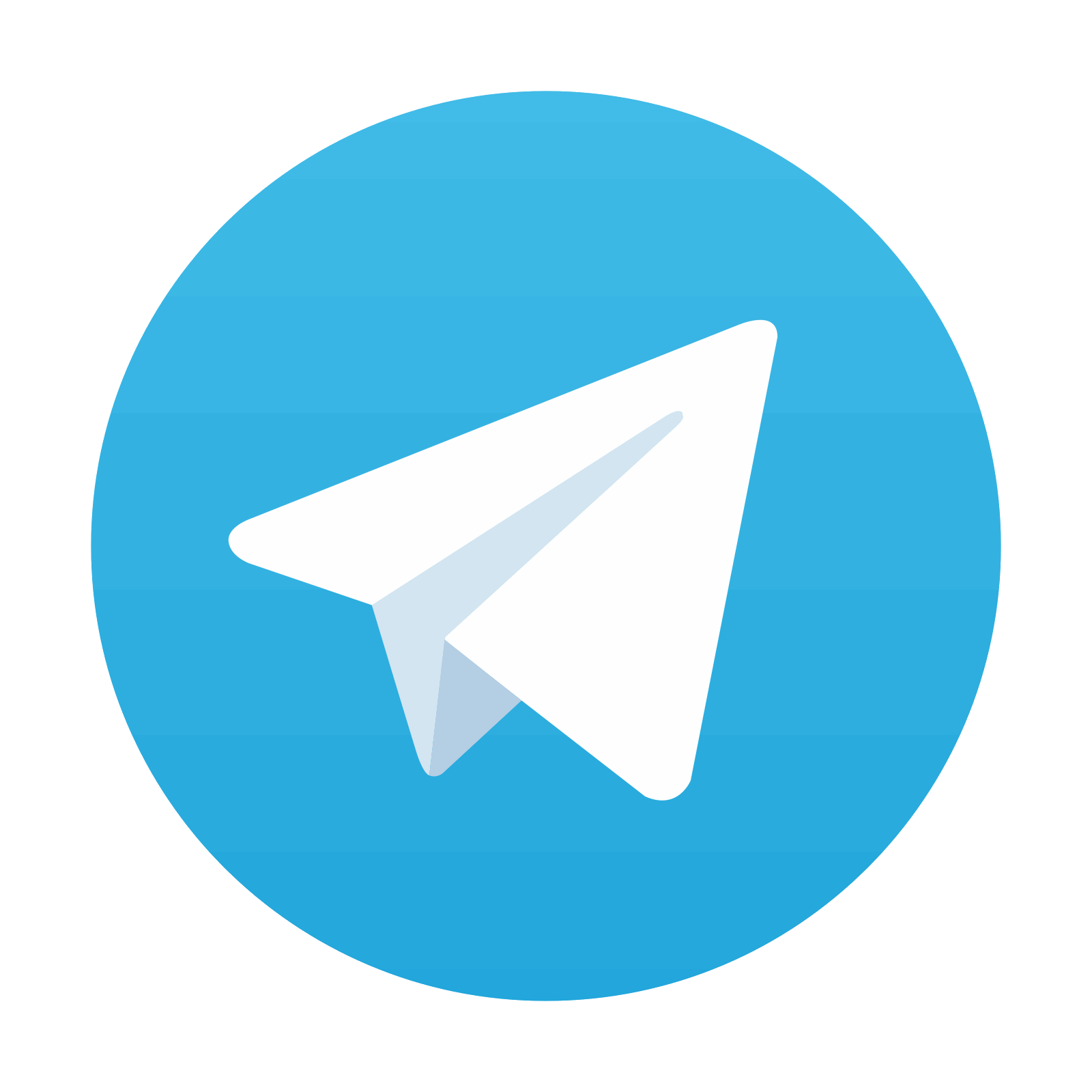
Stay updated, free articles. Join our Telegram channel

Full access? Get Clinical Tree
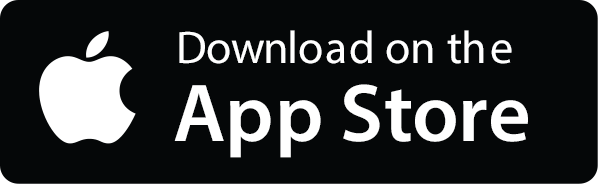
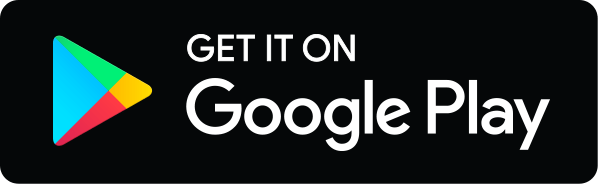