Metabolites
Pathways and genes
Up/downregulation folds
CR
ART
H2O2
Glucose
Glycolysis
Acs2
−17.28
−2.63
−3.02
Adh6
−6.18
−1.54
−3.06
Ald3
−1.49
−1.83
2.13
Eno1
−1.75
−1.38
−2.26
Pdc6
−4.88
−2.16
−3.71
Pgm2
−1.68
−1.74
−2.02
Pyk2
−1.27
−1.72
−2.20
Tdh2
−4.32
−4.17
−12.62
Tdh3
−1.49
−2.47
−10.36
Citrate cycle
Aco1
−1.71
−1.43
−1.63
Cit2
1.04
1.11
1.06
Fum1
−2.55
−1.16
−1.20
Idh1
−3.26
1.08
1.06
Kgd1
−1.58
−1.26
−1.28
Kgd2
−1.51
−1.15
−1.29
Lat1
1.00
1.32
1.39
Lsc2
−1.63
1.03
−1.32
Mdh3
1.55
1.39
1.39
Pck1
−2.21
−1.19
−1.26
Pdb1
−1.60
−1.04
−1.14
Pyc2
−1.73
1.46
1.33
Sdh4
−1.58
−1.12
−1.17
Fatty acids
Biosynthesis (including unsaturation and elongation)
Acc1
−2.46
1.50
−1.21
Fas1
−2.54
−1.00
−1.53
Fas2
−2.29
1.19
−1.22
Fen1
−2.03
1.59
1.54
Ifa38
−2.02
−1.22
−1.30
Sur4
−4.07
−2.47
−3.54
Tsc13
−2.37
−2.15
−2.85
Ole1
−2.94
−1.03
−1.12
Degradation (β-oxidation)
Dit2
2.86
2.61
2.82
Faa2
3.31
1.38
−1.06
Faa4
2.13
1.20
−1.16
Pot1
4.56
1.60
1.56
Pox1
5.99
2.31
1.78
Amino acids
Cysteine and methionine biosynthesis
Aro8
−4.17
−1.46
−1.54
Cys3
−3.91
−2.54
−4.85
Hom2
−2.09
−1.19
1.04
Irc7
−14.85
−1.89
−2.98
Met6
−2.24
−1.68
−1.88
Sah1
−7.18
−1.29
−1.29
Sam1
−4.11
−1.94
−1.43
Sam4
−4.94
−2.27
−2.64
Spe2
−2.84
−1.38
−1.32
Spe4
−2.02
−1.31
−1.19
Utr4
−2.71
−1.02
−1.05
Nucleotides
Purine and pyrimidine degradation
Apa1
2.73
1.84
2.40
Cdc8
2.25
1.11
−1.19
Dal3
2.70
4.26
4.10
Dbp3
2.24
3.34
4.70
Dbp4
3.28
1.47
1.65
Gud1
2.37
1.90
2.00
Hnt2
2.66
2.82
3.20
Met14
3.28
1.46
1.74
Prs2
2.14
1.50
1.61
Rnr3
2.02
1.02
1.30
Rpa12
2.08
1.11
2.07
Rpa34
2.50
−1.20
1.78
Rpa43
2.83
1.29
2.46
Rpc31
2.24
1.10
1.08
Rpc53
2.75
1.41
2.06
Trr2
3.18
1.24
1.26
As a major kind of energy metabolites, glucose is regenerated through gluconeogenesis and degraded through glycolysis. Because gluconeogenesis and glycolysis are inter-reversible, it is reasonable that gluconeogenesis/glycolysis pathway genes are downregulated by CR due to glucose restriction. Regarding to fatty acids, another family of major energy metabolites, it is also understandable that fatty acid biosynthesis pathway genes are downregulated, whereas fatty acid degradation pathway genes upregulated in all treatment groups. In similar, biosynthesis pathway genes for two kinds of amino acids, cysteine and methionine, are downregulated, whereas degradation pathway genes for two kinds of nucleotide bases, purine and pyrimidine, upregulated. From above results, we can predict that the biosynthesis of both proteins and nucleic acids should be attenuated or even halted because of food shortage and nutrition insufficiency in CR, which can be mimicked by ART and H2O2.
6.2.2.4 Downregulation of Genes for Protein Biosynthesis and Upregulation of Genes for Protein Degradation by CR and Mimetics
To further reveal the effect of CR and mimetics on protein biosynthesis and degradation, we examined the expression levels of yeast ribosomal protein large subunit genes (Rpl) and ribosomal protein small subunit genes (Rps) after treatment by CR and mimetics. As the consequence, more Rpl/Rps and other related genes are downregulated by CR and mimetics. During treatment, a dramatic decreased protein concentration was noticed in all tested samples. These results indicated that the biosynthesis of ribosomal proteins is inhibited, and ART or H2O2 can simulate CR in the modulation of ribosome genes and hence their expression.
Accordingly, ribosome biogenesis genes that accelerate the ribosomal protein biosynthesis were found to be highly upregulated in all groups, implying a feedback response of ribosomes from various stress conditions. Not surprisingly, upregulation of ribosome biogenesis genes is more remarkable for the CR group because its ribosome genes are extremely downregulated. At the same time, we also analyzed the impact of different treatments on the controlled degradation of proteins through ubiquitylation. Consequently, some ubiquitylation genes (Ubi) are upregulated, but others downregulated. These results proved that selective protein degradation is enhanced after treatments, by which amino acids that are released from degraded proteins can be re-utilized. Furthermore, it was also found that most of autophagy genes (Atg) are upregulated by each treatment albeit a few of which are downregulated. It is clear that CR and mimetics can partially augment autophagy in order to help yeast coping with environmental stress (Fig. 6.1).


Fig. 6.1
Transcriptome-wide profiling of selective protein degradation-related autophagy pathway genes. ART artesunate; CR calorie restriction. The red color indicates upregulation, and the green color represents downregulation
6.2.2.5 Mitohormesis: Modulation of Transporter Gene Expression Profiles and Lipid Metabolic Patterns by CR and Mimetics
Genome-wide expression profiling was used in the present study to compare the effects of CR and mimetics on ATP-binding cassette transporter gene expression profiles and lipid metabolic patterns. The dynamic changes of SOD activity were monitored in pretreated and post-treated yeast cells. After treatments, cellular detoxification-related ATP-binding cassette transporter genes are downregulated or unchanged, whereas the peroxisomal ATP-binding cassette transporter genes Pxa1 and Pxa2 that enhance long-chain fatty acid transport as well as the plasma membrane ATP-binding cassette transporter genes Aus1 and Pdr11 that accelerate sterol uptake are considerably upregulated (Table 6.2).
Table 6.2
ABC transporter gene expression profiles in yeast treated by CR and mimetics
ABC transporter gene | CR | H2O2 | ART |
---|---|---|---|
Plasma membrane | |||
Pdr5 | −4.57 | −2.33 | −1.29 |
Snq2 | 1.65 | −1.08 | 1.17 |
Pdr12 | −3.19 | 2.49 | 2.96 |
Pdr15 | −3.16 | −2.42 | −1.44 |
Aus1 | 1.31 | 1.42 | 1.05 |
Pdr11 | 2.43 | 2.41 | 2.02 |
Yor1 | −1.12 | −1.36 | 1.02 |
Ste6 | −2.08 | −2.12 | −2.82 |
Vacuole membrane | |||
Bpt1 | 2.23 | 1.56 | 1.36 |
Ybt1 | 1.06 | 1.34 | −1.11 |
Ycf1 | 1.26 | 1.63 | 1.54 |
Mitochondrial membrane | |||
Atm1 | 2.2 | 1.15 | 1.14 |
Mdl1 | −1.37 | 1.36 | 1.26 |
Mdl2 | 1.18 | 1.46 | 1.28 |
Peroxisome membrane | |||
Pxa1 | 5.47 | −1.66 | −1.43 |
Pxa2 | 4.11 | 2.00 | 1.98 |
These results indicated that mitohormesis depends on ATP-binding cassette transporter-mediated lipid transport and subsequent lipid degradation and re-utilization (Wang and Zeng 2014).
6.2.2.6 Expression Fluctuations of Oxidative Phosphorylation and Antioxidation Responsible Genes: Dual-Phase Responses During CR and Mimetic Exposure
The metabolic flux of glucose flows from the cytosol to mitochondria. Because the cytosolic glycolysis pathway genes are globally downregulated by CR and mimetics, it could be predicted that the mitochondrial oxidative phosphorylation pathway genes should be accordingly downregulated by each treatment. Indeed, it was found that those genes encoding COX (complex IV), F1F0-ATP synthase (Complex V), and other associated signature proteins are downregulated in every group, although the expression levels of those genes are much lower in the CR group than in the mimetic groups.
The analytic data of transcriptomic microarray indicated that H2O2 degradation-responsible antioxidant genes, such as Cat1 encoding mitochondria-localized CAT and Gpx2 encoding cytosolic GSH-POX, are upregulated by CR and mimetics. In contrary, H2O2 generation-responsible antioxidant genes, such as Sod1 and Sod2 coding for cytosolic copper-zinc SOD (Cu/Zn-SOD) and mitochondrial manganese SOD (Mn-SOD), are downregulated by CR and mimetics, implying that the main type of ROS in this stage is H2O2 other than O2 −. Besides, it was also seen that other antioxidant enzyme-encoding genes are either upregulated (Trx3), or downregulated (Gto3 and Dot5), or unchanged in different treatment groups.
As experimental evidence supporting above suggestion of H2O2 as a main type of ROS, we investigated the expression modes and activity dynamics of mitochondrial signatures including antioxidant enzymes. In ME yeast cells incubated for 12 h, CR increases both Cu/Zn-SOD and Mn-SOD activities. In contrast, in PME yeast cells incubated for several days, CR decreases both Cu/Zn-SOD and Mn-SOD activities. So CR-based “dual-phase responses” can be typically distinguished by the “up-and-down” modes of antioxidant responses.
Additionally, an elevation of the expression level of peroxisome genes in all treatment groups seemed to support above deduction that H2O2 has become the major kinds of ROS, which can be thoroughly scavenged by the cooperation of CAT, GSH-POX, and peroxisomal enzymes. More importantly, upregulation of peroxisomal β-oxidation enzymes contributes to energy generation by the oxidation of fatty acids released by the degraded storage lipids and recycled cellular components during carbon starvation.
6.2.2.7 Differential Regulation of Protein Kinase Genes in Logarithmic and Postlogarithmic Stages
It is noted that a repression of the respiratory activity should lead to the decrease of ATP and NADH, i.e., the elevation of AMP/ATP and NAD+/NADH ratios, which can in turn activate AMPK and SIRT1 separately. In postlogarithmic yeast cells, only a slight upregulation of Snf1 (coding for a yeast homolog of AMPK) and Sir2 (coding for a yeast homolog of SIRT1) was observed in CR treatment, but no significant changes of those genes were noticed in mimetic treatment. Additionally, Sch9, encoding a yeast homolog of mammalian ribosomal S6 kinase 1 (S6K1), was also found to be weakly upregulated in the CR group but unchanged in mimetic groups.
It was shown that mitogen-activated protein kinase (MAPK) pathway genes (Kss1 and Fus3 are yeast homologs of MAPK) are mostly downregulated by CR and mimetics, indicating a common modulation pattern in the PE phase. As a critical gene controlling cell fate and determining lifespan, Tor is upregulated in either treatment group, in which Tor1 is considerably upregulated for many folds. Because TOR is activated via phosphorylation, we do not expect that TOR activity is correlated with its transcript abundance. Nevertheless, it seemed that the inhibition of TOR activity may induce Tor expression perhaps in a feedback manner.
We further quantified the induced expression levels of Snf1, Tor1, and Kss1 in logarithmic yeast among treatment groups. It is very clear that their expression patterns are distinct in the logarithmic stage from those in the postlogarithmic stage. For example, Tor1 exhibits downregulation in the logarithmic stage, but shows upregulation in the postlogarithmic stage. In the logarithmic yeast, the overall expression levels of Snf1 and Kss1 that are induced by CR, ART, or H2O2 are typically lower than those in calorie nonrestriction. These results further strengthened that protein kinase genes are regulated in a dual-phase mode.
6.2.2.8 Covalent ART-Heme Conjugation and Synchronous COX Induction
Until currently, no direct evidence informs how CR elicits ROS and induces antioxidant enzymes. Considering the previous results demonstrating that ART can alkylate the heme moiety and hence inactivate heme-harboring proteins, we proposed that ART may covalently conjugate COX and permanently inhibit its activity, which in turn induces COX and increases its activity. Indeed, we found that ART really increases yeast COX activity in different concentrations and incubated for a varying duration. This finding might be well interpreted by an induction of Cox after ART conjugation to COX.
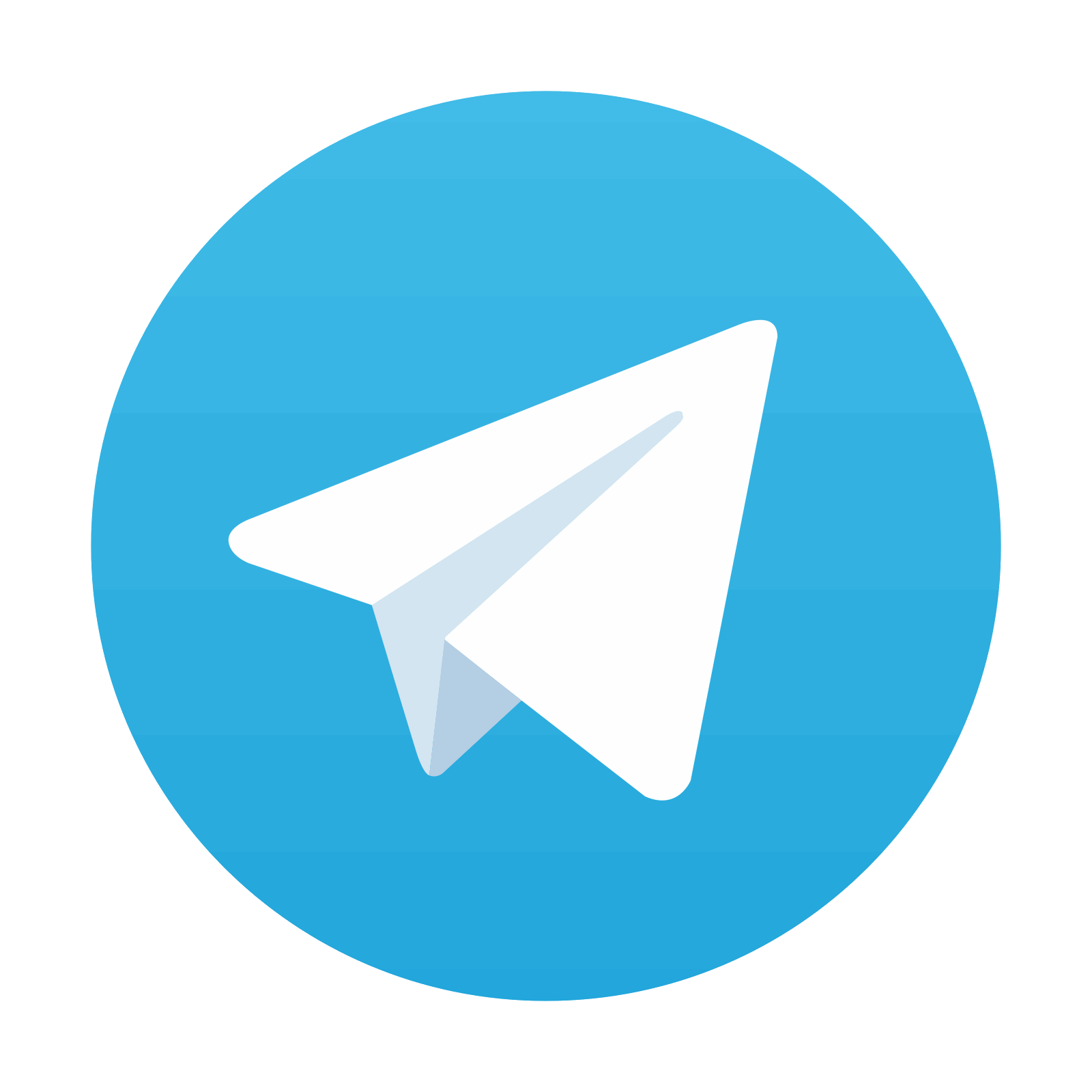
Stay updated, free articles. Join our Telegram channel

Full access? Get Clinical Tree
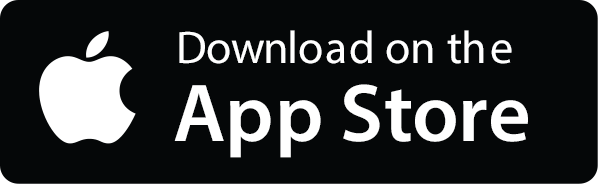
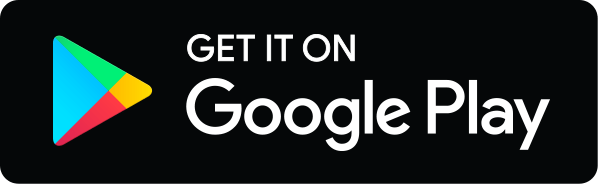