© The Author(s) 2016
Chris WillmottBiological Determinism, Free Will and Moral ResponsibilitySpringerBriefs in Ethics10.1007/978-3-319-30391-8_55. Are We Ready for an Expanded Use of Neuroscientific Evidence in the Courtroom?
(1)
Department of Molecular and Cell Biology, University of Leicester, Leicester, LE1 9HN, UK
Abstract
Evidence in the form of behavioural genetics and brain imaging has started to reach the courtroom. In this concluding chapter, the underlying validity of these methods will be examined. After review it will be determined that electroencephalography, positron emission tomography and functional magnetic resonance imaging are all appropriate techniques for examining the working brain. Despite being scientifically valid, however, it does not follow automatically that the uses for which such evidence has been offered in criminal cases were necessarily justified. Based on current experience, judiciaries would be wise to wait for more robust validation of neurobiological evidence before expansion of its use. This does not mean, however, that data derived via these techniques will not be integral to criminal proceedings in the future. Before taking that step, more closely matched reference populations need to be established, and the interaction of environmental stimuli alongside genetics needs to be better understood.
Keywords
Brain overclaim syndromeChristmas tree effectDeterminismEcological validityfMRIG2iMoral responsibilityReverse inferenceDevelopments in understanding of the genetics and neuroscience underlying behaviour have been amongst the most fruitful areas of recent scientific endeavour. The potential philosophical and legal implications of these discoveries are self-evident; if science were to show that our behaviour is entirely determined by biological factors this would undermine traditional notions of free will and, in turn, the concept of moral responsibility and culpability that underpins the legal system. As Roskies notes, “If moral responsibility is found to be incoherent, then our social, moral and legal systems will be eviscerated and the result will be chaos.” (Roskies 2006: p. 421).
Philosophically, it is only hard determinism1 (Sect. 1.3) which would rule out any notion of moral responsibility, since compatibilism retains scope for personal accountability. Evidence that severed the connection between someone’s actions, their moral responsibility and/or the appropriate punishment would have seismic effects on the organisation of democratic societies. Bear in mind, as well, that it might not even be necessary for the science to actually substantiate this attack on responsibility in order for the chaos described by Roskies to ensue. If there is sufficient popular perception that our behaviour is in some way hard-wired, and therefore outwith our conscious control, then trust in the legal process might be undermined regardless. The hypothesis that developments in neuroscience challenge traditional views regarding the existence of free will has certainly reached some quarters of the popular consciousness (e.g. Economist 2006; Chivers 2010; Gutting 2011; Stafford 2015).
Drawing together our reflections on the philosophical, legal and scientific dimensions of moral responsibility, we are led towards two bipartite questions. Firstly, is the genetic and brain analysis data regarding behaviour scientifically robust and, if it is, does the science support a deterministic worldview? Secondly, should the science be used in the courtroom and, if so, in what ways? Each of these issues will be considered in turn.
5.1 Is the Genetic and Brain Imaging Evidence on Behaviour Scientifically Robust and, if so, Does the Science Support a Deterministic Worldview?
If it was established that the science of behaviour could be accurately described via the study of neuroscience and genetics, it would not necessarily follow that this evidence ought immediately to play a role in the legal system. However, if the science was shown to be fundamentally flawed it would argue strongly against its use in the context of criminal justice.
The kinds of research being conducted to investigate the biological basis of behaviour have been discussed at length in Chap. 3. Here we examine specifically issues regarding the validity and reliability of this data considering, in turn, the brain imaging and the genetic approaches.
5.1.1 Neuroscience
Over the past century a variety of approaches to brain imaging have been developed (Table 3.1). In terms of potential applicability in the criminal court, it is the scientific validity of Electroencephalography (EEG), Positron Emission Tomography (PET) and functional Magnetic Resonance Imaging (fMRI) which need to be examined most closely.
EEG: After more than 80 years of use and refinement, the value of EEG as a method for examining cognitive processes is now well established (Lopes da Silva 2013). Recent developments are even bolstering the spatial as well as the temporal dimensions of EEG (Michel and Murray 2012). It remains, of course, possible to abuse a scientifically-robust technique by misapplication of the data generated and some controversy persists regarding the legal application of EEG-generated evidence as an assessment of incriminating memories (Sect. 4.4.1). The method itself is, nevertheless, considered reliable.
PET: As with EEG, PET is also considered to be a scientifically-validated method, with the highest sensitivity of any technique for measuring specific ligand-receptor interactions in situ (Zimmer and Luxen 2012). The use of glucose labelled with radioactive fluorine to study brain metabolism has been extensively researched and the underlying science is well understood.
The involvement of radioactivity is both a strength and a weakness of PET. The use of purposely-labelled molecules gives more straightforward signals than other methods such as fMRI (as we will see in a moment). However, the health risks associated with ionising radiation also limits their usage.
fMRI: Of these three techniques, therefore, it is the scientific validity of fMRI which requires the closest inspection. We live in an era where neuroscientific spin is being applied to an increasing number of fields. Aside from Neurolaw, there is growing interest in areas such as Neuromarketing (e.g. Ariely and Berns 2010), Neuropolitics (e.g. Henry and Plemmons 2012) and Neuroeconomics (e.g. Glimcher and Rustichini 2004).
Increased use of fMRI is at the core of this spread into different disciplines, which Geoffrey Aguirre, Neurologist at the Perelman School of Medicine has termed “imaging colonisation” (Aguirre 2014). As is often the case when an innovation carefully nurtured in one community is adopted by another, without the requisite history, there are concerns that new adopters may use the technology inappropriately and overstate the results. In keeping with the nomenclature, this has led to coining of the terms “Neuroskepticism” (e.g. Marks 2010; Rachul and Zarzeczny 2012) and even “Neurobollocks” (e.g. Poole 2012).
In a thorough and balanced review, University of Pennsylvania neuroscientist Farah (2014) has examined whether or not the fundamental criticisms of the science of fMRI (as opposed to their application in criminal cases) are warranted. A first area of concern emerges from the indirect manner in which the image data is generated. As noted in Sect. 3.1.1, fMRI actually reports on the magnetic properties of haemoglobin, which is influenced by the levels of oxygen within the blood, as a proxy for neuronal activity. Processing of information by the brain needs energy and the flow of blood bringing oxygen to the site serves a second-hand report of the action. Clearly this is not ideal, we would like to be able to “see” the nerve cells directly rather than measuring blood-oxygen level dependent (BOLD) variation. The “neurovascular coupling” between the two processes is, however, substantiated by a large number of empirical studies (Aguirre 2014). It is also important to note that dependency on secondary signals to obtain information about scientific process is not an issue unique to fMRI. As Farah points out with wilful alliteration, Chemists, Cosmologists and Climate Scientists all make inferences from secondary data. This is not, therefore, a fatal concern.
A second accusation levied at fMRI relates to the alluring simplicity of the images produced. The photograph-like appearance of brain scans presented in journal articles and, potentially, in court cases belies the enormous amount of statistical work and data processing that has been employed to reach that point. Some people get twitchy at the mere mention of statistics. As Farah notes, “while the incorrect use of statistics in indeed misleading, there is nothing inherently misleading about using statistics… When carried out properly, statistical analyses deepen our understanding of the data and the larger reality from which they are sampled. This is the whole point of using statistical methods in any field, from cognitive neuroscience to demography.” (Farah 2014: p. S25). The take-home message therefore is not that the use of statistics is dubious per se, but to remind us that an MRI machine being used for functional analysis of someone’s brain is not actually capturing a multi-coloured photo mid-thought.
To get a feel for the scale of number-crunching involved, consider the following. As part of the fMRI scanning process, the brain is divided into sections known as volumetric pixels, or voxels for short. A typical study might involve data collection from 50,000 to 100,000 voxels in any one pass (Aguirre 2014). Re-scans then occur every 1–3 s, possibly for as long as an hour in total. That’s a lot of data points!
With so many readings at such a large number of voxels, it is inevitable that random fluctuations will occur. Even if this occurs once in every thousand voxels, that would mean that something in the order of 50–100 voxels in our typical study might be randomly activated and potentially misconstrued as exhibiting a genuine response. A threshold therefore needs to be set in order to try and guard against changes that were mere chance (false positives), whilst trying to avoid missing real phenomena by setting the threshold too high (false negatives).
The danger of the former problem was brought into stark relief by an infamous experiment where researchers placed a dead Arctic Salmon in their MRI scanner. They put the salmon through the same experimental protocol that they used for their human subjects, and monitored brain activity as the fish was exposed to a series of photographs depicting human emotions (Bennett et al. 2010). They were surprised to observe apparent signals from the brain of the dead fish in response to some of the photos. Evidently these could not be detecting genuine behaviour, they must have been random changes. Carrying out simple statistical refinements enabled them to confirm these “findings” were artefacts. Nevertheless, what had started out as a joke in fact turned into an important lesson about conducting the appropriate safeguards.
As expertise in fMRI has grown, few scientists would submit a manuscript with such glaring lack of baseline control, and even fewer journals would consider publishing them. There are, however, other statistical errors that have been more persistent in the literature. Vul et al. (2009) conducted a systematic review of 53 published papers linking regions of the brain to behavioural traits. They identified that 28 fell foul of what they term the “non-independence error”. In essence these studies were guilty of a degree of circularity in their reasoning. A first pass of the data was used to select interesting voxels for further study. These are likely to be areas showing different levels of activity when the research subject participates receives two contrasting stimuli. The researchers then conducted a secondary analysis of the same data pertaining to those selected regions in order to quantify the difference in activation. They were therefore measuring correlation in areas where they have already decided there is correlation. As Vul and colleagues point out, this does not mean to say the identified relationships are not valid (though some may be false), but the significance of the correlation is likely to have been overstated.
The apparent strength of association between certain tasks and particular regions of the brain can also be inappropriately emphasised through the choice of false-colour for modelling the activity in a final fMRI image. Vibrant colours are usually chosen to illustrate areas where a change in blood flow has been noted. Often the documented changes are relatively slight, but use of bright colour in conjunction with generous calibration of change-in-colour related to change-in-BOLD signal might make this appear more significant than is truly warranted. This has led to worries about the so-called “Christmas Tree Effect”, which we will discuss in further detail in Sect. 5.2.
An aspect of some fMRI use where concerns are justified involves the process of “reverse inference”. This is most prominent in the potential use of fMRI as a means of lie-detection. In reverse inference an observation is made about the activity of the brain, from which an assumption is made that the observer can know the thought processes that have led to that change (as opposed to “forward inference” in which the participant is given a known stimulus and the impact on brain response is then noted).
There is general consensus that under controlled laboratory conditions, some areas of the brain are more active when the subject is instructed to lie than when they are telling the truth (Farah et al. 2014). The correlation becomes more dubious, however, when we see activity in those sections associated with lying and assume therefore that the subject is being untruthful. It may be that other processes can lead to similar patterns of response within the brain. This is a field of research that is ripe for greater validation, after which it would still be better practice to interpret reverse inference data as offering a percentage probability of a client’s truthfulness rather than a stark affirmation that they are, or are not, lying (Farah 2014).
Finally, there is a concern that apparent changes in the brain may, in fact, be influenced by other physiological processes such as breathing, heartbeat or other movement (no-one can stay perfectly still for the full duration of an fMRI scan). There is thus a theoretical danger of misinterpretation. Since, however, any well-conducted scientific study is going to look for multiple observations of the same phenomenon, it ought to be possible to disregard any one-off movement. A more significant problem might arise in so called “cognitive information testing” (Sect. 4.4.1) in which a suspect is asked questions relating to a crime scene. A guilty, but canny, defendant might be able to “game” the test by employing countermeasures such as thinking about something entirely different or making subtle movements of fingers or toes.
On balance, however, informed scholars are happy that fMRI sits comfortable alongside EEG and PET as a legitimate tool for brain study. “Contrary to the claims of some critics, neuroimaging is not modern phrenology” (p. S9), concludes Aguirre (2014), adding “It is now possible to conduct an fMRI study with great confidence in the statistical validity of the results and with a clear–eyed understanding of the assumptions on which any claims are based” (p. S16).
Of course, confirmation of a correlation between defined regions of the brain and particular behaviours is not de facto an indication of causation, less still of compulsion. The other brain studies which have been interpreted as challenging the notion of free will are the Benjamin Libet-style investigations, in which an electrical signal, the readiness potential, appears to precede conscious awareness of a decision to move. The interpretation of such experiments has already been discussed at considerable length in Sect. 3.3.4.
It is worth reiterating here that although the readiness potential itself seems to be a real phenomenon there are diverse views regarding its significance. I am sufficiently persuaded by the critiques of Adina Roskies, Alfred Mele and others that the inevitable constraints of the experiments mean that they are not necessarily offering the insights into the timing and control of volitional actions implied by the determinists. The failure to adequately distinguish between measurement of when to act and whether to act, the potential difference between conscious intent and consciousness of conscious intent, and the inability to probe decisions made after a long period of deliberation all leave open the reality of free will, and moral responsibility.
5.1.2 Genetics
Unlike ongoing controversies about interpretation of the readiness potential, it is now beyond dispute that genetic factors play important roles in behaviour. Traditional twin and adoption studies (Sect. 3.2) which hinted at a genetic component to behaviour are being supplemented by fresh insights from molecular biology. Experiments involving animal models, corroborated by research on humans, have confirmed that there is a genetic dimension to conduct. The focus therefore has shifted from if genes have an effect on behaviour to identification of how genes are involved in behaviour and elucidation of their specific function(s).
The notion that genes alone are responsible for behaviour has, however, been systematically undermined by the most recent discoveries in the field. An intuitive sense that experiential stimuli and other “environmental” factors must have an influence on behaviour is now being supported at the molecular level through discoveries in the emerging fields of epigenetics and control via small non-coding RNA molecules (Sect. 3.2). As Oxford Professor Denis Noble has observed, “Without genes we would be nothing. But it is equally true to say that with only genes we would also be nothing” (Noble 2006: p. 44).
The need for genetic and environmental interactions (G × E) may go a long way to explaining why genome-wide association studies (GWAS) have not been as effective as anticipated at linking specific genes to particular conditions, such as diseases or behavioural patterns (Maher 2008). GWAS research involves the side-by-side comparison of the genomes of a large number of people in the hope that a spotlight will be shone on the gene or genes responsible for their shared condition. If a combination of environmental stimuli and genes are required to cause the development of a characteristic, then examination of the genes in isolation may not elicit the intended outcome. Whatever the underlying reasons for the failure of GWAS approaches to deliver the expected correlations, it is clear that a “bottom-up”, causal reductionist, model in which genes blindly determine our responses, is now untenable.
We must also maintain some restraint regarding extrapolation of genetic evidence derived from animal research. In recent years, the ability to conduct comparisons of the genomes of humans and other species has facilitated the deliberate modification of interesting genes in simpler, model organisms in order to tease out information regarding the potential roles of proteins encoded by these “candidate” genes (Fry and Willmott 2011, 2012). This reductionist approach, has borne fruit, but due caution is required in interpreting findings, particularly in the area of behaviour. If a fruitfly or a mouse responds in a mechanistic manner as a consequence of a particular genetic mutation, it does not follow implicitly that humans are driven in the same way, even if they are shown to have the equivalent mutation.
As has already been noted (Sect. 3.1.2), it is the extensive development of the frontal lobe, the seat of executive functions, which marks out the human brain as different to those of other species (Miller and Cohen 2001). Crucially, a sizeable portion of this additional capacity is given over to self-inhibition, the deliberate decision to countermand inappropriate responses, and therefore not to act in certain ways (Brass and Haggard 2007; Kühn and Brass 2009). There is plenty of scope for “top-down” regulation; as Chivers (2010) notes “there’s a whole brain network associated with holding back things you shouldn’t do”. Once again correlation is not causation, is not compulsion.
So, whilst a role for genes in behaviour is now established, and some brain imaging is endorsing the connections between neuroarchitecture and certain behaviours, any suggestions that moral responsibility has been killed-off have been greatly exaggerated. What bearing does this have on legal process?
5.2 Should the Science Be Used in Court and, if so, in What Ways?
As noted in Sect. 2.4, there is a widely held view that the current UK legislation regarding insanity, diminished responsibility and automatism in criminal cases is in need of major overhaul.2 Legal experts are certainly keen to plan for a greater application of appropriate science in their proceedings. David Ormerod, heading up the Law Commission’s review on fitness to plead, has argued that “Modern criminal law should be informed by modern science and by modern psychiatric thinking” (Ormerod 2010).
Transferring this worthy intention into legal practice is not, however, a straightforward task. Interaction of scientific evidence and legal process can lead to curious outcomes; witness the peculiar interpretations of “disease of the mind” discussed in Sect. 2.1.2. At a fundamental level, the law is ultimately looking for a binary solution—the defendant is guilty or the defendant is innocent—whereas science is more likely to present the defendant as being at a particular position on a spectrum (Martell 2009).
Both genetic and brain imaging data have now been cited in criminal cases in various jurisdictions around the world (see Sects. 4.3 and 4.4). However, their usage has been introduced in an uncoordinated manner, and serious concerns have been raise about the appropriateness of the applications to which such evidence has been put.
Although data of this kind may, in the future, become sufficiently reliable to warrant regular courtroom usage, the consensus amongst experts seems to be that the current use of neuroscientific data in determination of criminal responsibility is somewhat premature. Stephen Morse, Professor of Psychology and Law at the University of Pennsylvania, has been at the vanguard of this criticism. Somewhat tongue-in-cheek, Morse has spoken of a new pathological condition Brain Overclaim Syndrome, for which the principal symptom is a tendency “to make claims about the implications of neuroscience for criminal responsibility that cannot be conceptually or empirically sustained” (Morse 2006: p. 397).
Morse and other critics point to a number of substantive difficulties regarding the applicability of such evidence. By way of illustration, no pun intended, let’s take the use of brain images such as those obtained using Positron Emission Tomography (PET) or functional Magnetic Resonance Imaging (fMRI).
If a court introduces neuroimage data into their proceedings it will be because they are seeking to obtain valid and relevant information regarding the role brain function might have played in the culpability of a given individual who, it is believed, has carried out a particular act at a specified time. However, interpretation of PET or fMRI scans cannot be made in isolation; there is a requirement to compare the suspect’s brain against reference values. The latter are derived by averaging measurements taken from multiple different people. As a consequence there is an issue known as the “Group to individual” problem, or the G2i inference. This is awkward on both a philosophical and a pragmatic level. Philosophically, the G2i problem involves taking averaged data, whose purpose was to convey “this is generally true” and comparing it against the behaviour of one individual in one context. This has the potential to ride roughshod over all sorts of nuances in their particular case. Pragmatically, there may be defendant-specific differences in, say, brain shape or cellular neuroarchitecture that raise doubts about the legitimacy of comparison to an averaged brain.
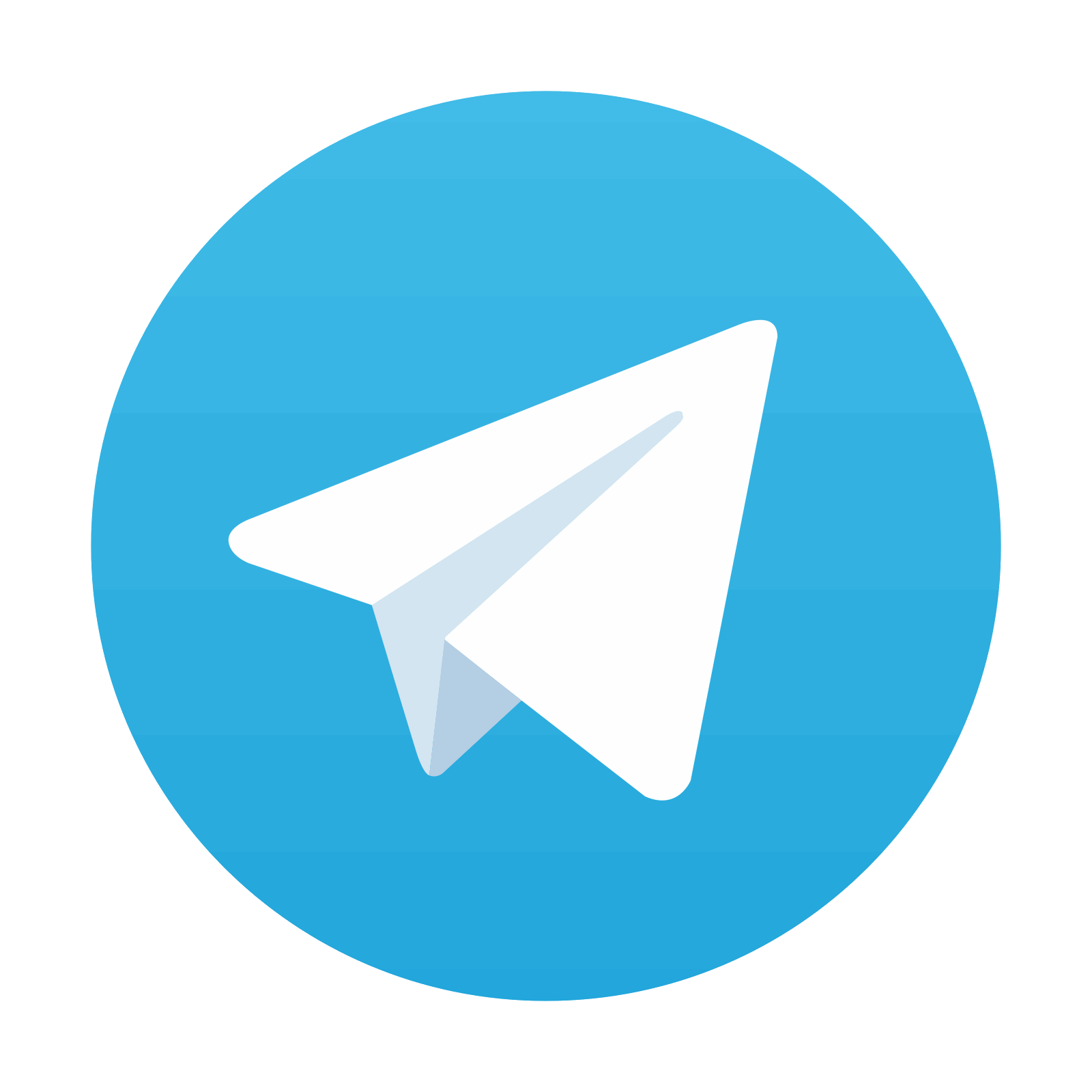
Stay updated, free articles. Join our Telegram channel

Full access? Get Clinical Tree
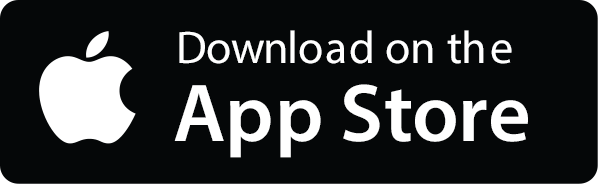
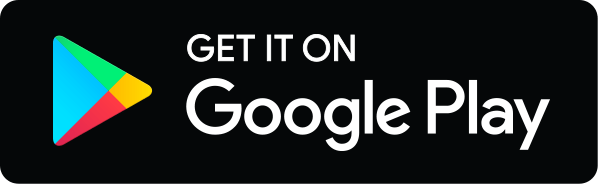