FIGURE 36-1 (A) PAC from the right superior pulmonary vein (RSPV) that triggered AF. Once AF was sustained, a CFAE map was performed as shown in B. The LA map was created during AF. The map displays CFAE areas with respect to the shortest cycle length of the fractionated electrogram. Red areas have the shortest cycle length, and in this patient the RSPV, septum, and antrum of the left inferior pulmonary vein (LIPV) had the shortest cycle length (<100 ms). (C) The electrograms recorded from the LIPV as shown in the inset (arrow); note the much shorter cycle length at this site compared to the electrograms recorded from the coronary sinus. The red dots are the RF application points. Ablations were performed in these areas and rendered AF termination (D) and noninducible tachyarrhythmias with a high dose isoproterenol infusion (20 μg/min E).
ABLATION THERAPY FOR ATRIAL FIBRILLATION
The watershed observation by Haissguerre et al that PVs are an important source of triggering foci for paroxysmal AF has drawn electrophysiologists’ attention to the PVs as important target sites for AF ablation1. Although the initial approach was to focally ablate the culprit PV that was identified as the triggering site initiating AF,1,2 this approach quickly was out of vogue because numerous limitations: (1) difficulty of mapping the triggering focus due to a paucity of spontaneous AF coupling and time consuming because inducing the triggering arrhythmia often required multiple provocation and was quite inconsistent; (2) a daunting task of mapping multiple triggering foci; (3) multiple cardioversions were often needed for those whose AF became persistent. The strategy then changed to attempt to isolate the electrical connections of all four veins from the LA muscle.3–5 Various techniques were utilized to achieve electrical isolation of PVs: segmental isolation introduced by Haissaguerre and his colleagues,3 PV isolation at the antrum at the atrium-venous junction,4 or electroanatomical mapping and circumferential PV ablation.5
However, a total isolation of all four PVs poses a risk of PV stenosis. Furthermore, this strategy assumes that PVs are virtually the primary source of triggers or the perpetuator of AF, and thus all AF patients were essentially treated the same way—guided by anatomical scheme rather than electrophysiological mapping. As a result, electrical isolation of PVs has a variable rate of success in treating patients with all types of AF.6,7
We have utilized a different approach in ablating AF by using electroanatomical mapping of a substrate that perpetuates AF through identification of areas that have CFAEs during AF.
CHARACTERISTICS OF ATRIAL ELECTROGRAMS DURING ATRIAL FIBRILLATION
When one maps both atria during AF, one finds that there are three types of atrial electrogram characteristics8–10 (Figure 36-2). Atrial electrograms during sustained AF have three distinct patterns: single potential, double potential, and complex fractionated potential (CFAE). There are significant differences in these atrial electrograms during AF with respect to their cycle lengths and patterns and their regional distribution. Interestingly, areas that harbor CFAE with a very short cycle length have proclivity to localize and cluster in specific areas of the atria and do not meander; in other words, these atrial electrograms exhibit surprisingly remarkable temporal and spatial stability. These CFAE areas represent the AF substrate sites and become important target sites for AF ablation. By ablating such areas that have persistent CFAEs recording, one eliminates AF and usually renders AF noninducible. With this observation, CFAE mapping has become a novel approach for guiding a successful ablation of AF substrate with excellent long-term outcomes.
FIGURE 36-2 Complex fractionated atrial electrograms (CFAEs) are composed of two deflections or more and/or have a perturbation of the baseline with continuous deflections from a prolonged activation complex with a very short cycle length. This figure shows an electroanatomical map of the LA in the LAO-oblique view, displaying the shortest cycle length of the recording sites in the span of 2.5 seconds ranging from 50 to 120 ms. The site from anterior aspect of the LA around the antrum of right superior pulmonary veins (arrow) had continuous low voltage fractionated atrial electrograms with the shortest cycle length of 54 ms (Map 1-2). In contrast, electrograms recorded from the coronary sinus (Ref 1-2) showing varying three types of electrograms in the same short time span; note that CFAE of this site are fleeting, sandwiching between a double potential electrogram and a single potential; note also that the cycle length of this recording site is much longer than that of the Map 1-2 recording site. CFAE recorded from Map 1-2 is an ideal target for ablation.
CFAEs are usually low voltage multiple potential signals between 0.05 and 0.15 mV. CFAEs are defined as follows: (1) atrial electrograms that have fractionated electrograms composed of two deflections or more, and/or have a perturbation of the baseline with continuous deflection of a prolonged activation complex (Figure 36-2). (2) Atrial electrograms with a very short cycle length (<120 ms) with or without multiple potential; however, when compared to the rest of the atria, this site has the shortest cycle length (Figure 36-3) that drives the rest of the atria. These CFAEs, as shown in Figure 36-3, are also important targets for ablations.
FIGURE 36-3 An example of CFAE that has very short cycle length. It is much shorter than those recorded from the rest of atria, despite having no multiple prolonged potentials.
ELECTROPHYSIOLOGIC MECHANISMS UNDERLYING CFAEs
The elegant study by Konings et al showed that the complex, multiple component fractionated electrical potentials observed during intraoperative mapping of human AF were found mostly in the areas of slow conduction and/or pivot points where the wavelets turn around the end of the arch of the functional block.8,9 Thus, such areas of fractionated electrical recordings during AF represent either continuous reentry of the fibrillatory waves into the same area or the overlap between different wavelets entering the same area at different times.
Kalifa et al12 identified a key relationship between areas of dominant frequency and areas of fractionation in sheep. The investigators were able to localize areas with regular, fast, spatio-temporally organized activity and map the regions around them. Waves propagating from these areas were found to break and change direction recurrently at a boundary zone and demonstrate fractionation of local electrograms. Their findings suggested that one of the possible electrophysiologic mechanisms for AF relating to the hypothesis that high-frequency reentry at the boundary zones is responsible for the fractionation.
The most prominent theory underlying the occurrence of CFAE involves the complex interplay of the intrinsic cardiac nervous system on atrial tissues. The cardiac ganglionic plexi (GP) are a collection of autonomic nervous tissues with afferent and efferent sympathetic and parasympathetic fibers.13,14 Six major GPs that may exert influence on the atria are the superior LA, the posterolateral LA, the posteromedial LA, the anterior descending LA, the posterior right atrium (RA), and the superior RA. In animal models, the stimulation of parasympathetic fibers within the GP has been shown to decrease atrial effective refractory periods and allow AF to perpetuate. Quan KJ et al13 showed that electrical stimulation of cardiac ganglia near the PV orifices significantly shortened the atrial refractoriness close to the site of the stimulation and that the effects diminished at >2 cm away from this site. This raises the possibility that neurotransmitter release ie, acetylcholine, at preganglionic, and/or postganglionic terminal may contribute to the genesis of CFAEs and may play role in the differences of CFAEs regional distribution in the atria during AF. Indeed, Scherlag et al14 have demonstrated quite convincingly that in the areas of the LA where the GPs are identified by high frequency stimulation, CFAEs were almost always recorded after the high frequency stimulation initiated AF. They suggest that a marked shortening of action potential duration and formation of early repolarization caused ectopic beats, initiating AF that was sustained by the marked shortening of the refractory period. Ongoing research has identified a close relationship between the location of CFAE and the GP in animal models.15–18 CFAE-targeted ablation may provide a surrogate for modification of the GP if this relationship can be confirmed in humans. Certainly, ablation in areas that have resulted in a vagal response has shown excellent results in the treatment of AF.
It is possible that all of the above electrophysiologic changes are the underlying causes of CFAE. However, regardless of the mechanism underlying CFAEs, it is very likely that CFAE areas represent substrate areas that perpetuate AF.
REGIONAL DISTRIBUTION OF CFAE
The regions that harbor CFAE are not symmetrically located within the atria, and the distribution of CFAE in the right and left atria is vastly different from one area to another but can be predictably sought in certain places during mapping.10,11 CFAE are surprisingly stationary and exhibit relative spatial and temporal stability; thus, one can perform point-to-point mapping of these CFAE areas and associate them into an electroanatomical map. The following key areas have demonstrated a predominance of CFAE within our cohort: the proximal coronary sinus; the superior vena cava-RA junction; the septal wall anterior to the right superior and inferior PVs; the anterior wall medial to the LA appendage; the area between the LA appendage and left superior PV; and the posterosuperior wall medial to the left superior PV (Figure 36-3
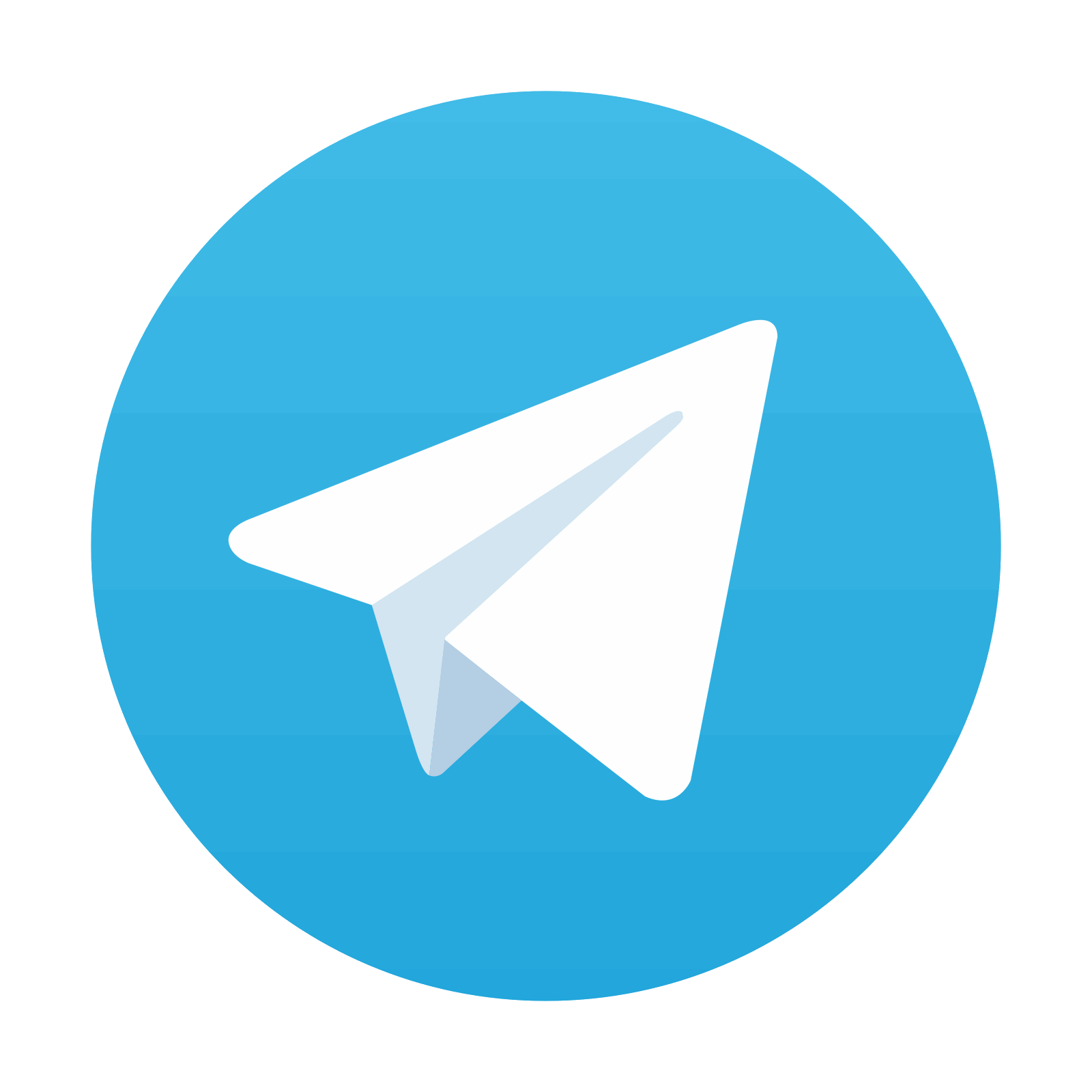
Stay updated, free articles. Join our Telegram channel

Full access? Get Clinical Tree
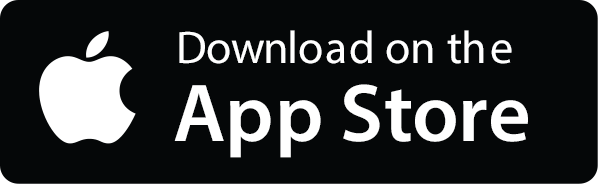
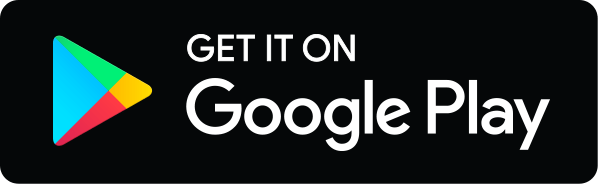