44
Antiviral Agents and Treatment of HIV Infection
This chapter is a composite of Chapters 58 and 59 in Goodman and Gilman’s The Pharmacological Basis of Therapeutics, 12th Edition. The material presented here will be more useful after having a basic understanding of the material presented in these chapters. This chapter does not contain detailed information about all antiviral or antiretroviral agents. For the details of agents not discussed here, see Chapters 58 and 59 in the 12th Edition of Goodman and Gilman’s The Pharmacological Basis of Therapeutics. In addition to the material presented here, the 12th Edition contains:
• Table 58-2 which provides the nomenclature of antiviral agents
• Figures 58-2, 58-4, 58-6, 59-2, 59-4, and 59-6 which show the chemical structures of each of the drugs discussed in this chapter
• At the beginning of Chapter 59 in Goodman and Gilman’s The Pharmacological Basis of Therapeutics, 12th Edition, there is an excellent overview of human immunodeficiency disease (HIV) infection and its treatment, the pathogenesis of HIV-related disease, and the principles of HIV chemotherapy
• Table 59-1 which is a list of antiretroviral agents approved in the United States
• Tables 59-2, 59-3, and 59-4 which provide detailed information on the pharmacokinetics of antiretroviral agents
LEARNING OBJECTIVES
Understand the treatment of herpes virus infections and the use of antiherpes drugs.
Know the treatment strategies for chronic hepatitis B and C infections.
Understand the mechanisms of action and resistance, and the therapeutic use of the anti-influenza agents.
Know the principles of HIV chemotherapy.
Know the mechanisms of action and resistance, the untoward effects, and the therapeutic uses of the drugs used to treat HIV infections.
DRUGS INCLUDED IN THIS CHAPTER
MECHANISMS OF ACTION AND RESISTANCE OF ANTIVIRAL DRUGS
FIGURE 44-1 Replicative cycles of DNA (A) and RNA (B) viruses. The replicative cycles of herpesvirus (A) and influenza (B) are examples of DNA-encoded and RNA-encoded viruses, respectively. Sites of action of antiviral agents also are shown. cDNA, complementary DNA; cRNA, complementary RNA; DNAp, DNA polymerase; mRNA, messenger RNA; RNAp, RNA polymerase; vRNA, viral RNA. The symbol (“T-line”) indicates a block to virus growth. A. Replicative cycles of herpes simplex virus, a DNA virus, and the probable sites of action of antiviral agents. Herpesvirus replication is a regulated multistep process. After infection, a small number of immediate-early genes are transcribed; these genes encode proteins that regulate their own synthesis and are responsible for synthesis of early genes involved in genome replication, such as thymidine kinases, DNA polymerases, etc. After DNA replication, the bulk of the herpesvirus genes (called late genes) are expressed and encode proteins that either are incorporated into or aid in the assembly of progeny virions. B. Replicative cycles of influenza, an RNA virus, and the loci for effects of antiviral agents. The mammalian cell shown is an airway epithelial cell. The M2 protein of influenza virus allows an influx of hydrogen ions into the virion interior, which in turn promotes dissociation of the RNP (ribonuclear protein) segments and release into the cytoplasm (uncoating). Influenza virus mRNA synthesis requires a primer cleared from cellular mRNA and used by the viral RNAp complex. The neuraminidase inhibitors zanamivir and oseltamivir specifically inhibit release of progeny virus. Small capitals indicate virus proteins.
FIGURE 44-2 Mechanism of action of acyclovir in cells infected by herpes simplex virus. A herpes simplex virion is shown attaching to a susceptible host cell, fusing its envelope with the cell membrane, and releasing naked capsids that deliver viral DNA into the nucleus, where it initiates synthesis of viral DNA. Acyclovir molecules entering the cell are converted to acyclovir monophosphate by virus-induced thymidine kinase. Host-cell enzymes add 2 more phosphates to form acyclovir triphosphate, which is transported into the nucleus. After the herpes DNA polymerase cleaves pyrophosphate from acyclovir triphosphate (indicated by the blue arrow in the inset), viral DNA polymerase inserts acyclovir monophosphate rather than 2’-deoxyguanosine monophosphate into the viral DNA (indicated by black arrows in the inset). Further elongation of the chain is impossible because acyclovir monophosphate lacks the 3’ hydroxyl group necessary for the insertion of an additional nucleotide, and the exonuclease associated with the viral DNA polymerase cannot remove the acyclovir moiety. In contrast, ganciclovir and penciclovir have a 3’ hydroxyl group; therefore, further synthesis of viral DNA is possible in the presence of these drugs. Foscarnet acts at the pyrophosphate-binding site of viral DNA polymerase and prevents cleavage of the pyrophosphate from nucleoside triphosphates, thus stalling further primer template extension. The blue bands between the viral DNA strands in the inset indicate hydrogen bonding of the base pairs. (Adapted from Balfour HH. Antiviral drugs. N Engl J Med. 1999, 340:1255–1268.)
FIGURE 44-3 Interferon-mediated antiviral activity occurs via multiple mechanisms. The binding of interferon (IFN) to specific cell surface receptor molecules signals the cell to produce a series of antiviral proteins. The stages of viral replication that are inhibited by various IFN-induced antiviral proteins are shown. Most of these act to inhibit the translation of viral proteins (mechanism 2), but other steps in viral replication also are affected (mechanisms 1, 3, and 4). The roles of these mechanisms in the other actions of IFNs are under study. 2’5’A, 2’-5’-oligoadenylates; eIF-2α, protein synthesis initiation factor; IFN, interferon; mRNA, messenger RNA; Mx, IFN-induced cellular protein with antiviral activity; RNase L, latent cellular endoribonuclease; tRNA, transfer RNA. (Modified from Baron, S., Coppenhaver, D.H., and Dianzani, F., et al. Introduction to the interferon system. In, Interferons: Principles and Medical Applications. [Baron, S., Dianzani, F., Stanton, G.J., et al., eds.] University of Texas Medical Branch Dept. of Microbiology, Galveston, TX, 1992, pp. 1–15. With permission.)
FIGURE 44-4 Structures and mechanism of nucleoside and nucleotide reverse transcriptase inhibitors.
FIGURE 44-5 Replicative cycle of HIV-1 showing the sites of action of available antiretroviral agents. Available antiretroviral agents are shown in blue. cDNA, complementary DNA; gp120 + gp41, extracellular and intracellular domains, respectively, of envelope glycoprotein mRNA, messenger RNA; RNase H, ribonuclease H; RT, reverse transcriptase. (Adapted from Hirsch MS and D’Aquila RT. Therapy for human immunodeficiency virus infection. N Engl J Med. 1993, 328:1686–1695.)
FIGURE 44-6 Mechanism of action of an HIV protease inhibitor. Shown here is a phenylalanine-proline target peptide sequence (in blue) for the protease enzyme (in black) with chemical structures of the native amino acids (in lower box) to emphasize homology of their structures to that of saquinavir (at top).
FIGURE 44-7 Structures and mechanism of non-nucleoside reverse transcriptase inhibitors.
FIGURE 44-8 Mechanism of action of the HIV entry inhibitor maraviroc.
FIGURE 44-9 Mechanism of action of the HIV integrase inhibitor raltegravir.
A 47-year-old man is immunocompromised because of the medications he is taking to prevent a heart transplant rejection. He develops a herpes simplex virus (HSV) infection of his lower lip.
a. What are his treatment options?
Infection with herpes simplex virus 1 (HSV-1) typically causes diseases of the mouth, face, skin, esophagus, or brain. Herpes simplex virus 2 (HSV-2) causes infections of the genitals, rectum, skin, hands, or meninges. Acyclovir and its ester prodrug, valacyclovir, are the prototypes of a class of drugs that are phosphorylated intracellularly to become inhibitors of viral DNA synthesis. Other drugs that use the same strategy include penciclovir, its prodrug famciclovir, and ganciclovir, and its prodrug valganciclovir. Cidofovir and foscarnet are available to treat acyclovir-resistant HSV infections. Idoxuridine is available for the topical (ophthalmic) treatment of HSV keratitis. Trifluridine is available for the topical treatment of keratoconjunctivitis and recurrent epithelial keratitis owing to HSV-1 and HSV-2. Topical trifluridine appears to be effective in some patients with acyclovir-resistant HSV cutaneous infections.
b. What is the mechanism of action of acyclovir?
The stages of viral replication and possible targets of action of antiviral agents are shown in Table 44-1. Acyclovir inhibits viral DNA synthesis via a mechanism outlined in Figure 44-2. Its selectivity of action depends on interaction with 2 viral proteins: HSV thymidine kinase and DNA polymerase.
TABLE 44-1 Stages of Virus Replication and Possible Targets of Action of Antiviral Agents
c. What are the possible mechanisms of acyclovir resistance?
Acyclovir resistance in HSV has been linked to one of three mechanisms (see Table MECHANISMS OF ACTION AND RESISTANCE OF ANTIVIRAL DRUGS): impaired production of viral thymidine kinase, altered thymidine kinase substrate specificity (eg, phosphorylation of thymidine but not acyclovir), or altered viral DNA polymerase. The most common resistance mechanism in clinical HSV isolates is absent or deficient viral thymidine kinase activity.
d. If he is treated with acyclovir, what adverse events should this patient be warned about?
Oral acyclovir is associated with nausea, vomiting, diarrhea, rash, and headache. The principal dose-limiting toxicities of intravenous acyclovir are renal insufficiency and CNS side effects. The renal effects are due to high urine concentrations of the drug causing crystalline nephropathy. Neurotoxicity is manifested by altered sensorium, tremor, myoclonus, delirium, seizures, or extrapyramidal signs.
An otherwise healthy 76-year-old woman developed a rash on her side and back 5 days ago. She now describes considerable pain in the area. Her physician suspects varicella zoster virus (VZV) infection, commonly known as shingles.
a. What are the treatment options for this woman?
The 2 drugs most commonly used for VZV infections are acyclovir and penciclovir, or their prodrugs, valacyclovir, and famciclovir, respectively. Both drugs are effective if started within 24 hours of the rash.
b. Her physician elects to use famciclovir. Is it likely to be effective?
In immunocompetent adults with herpes zoster of less than or equal to 3 days’ duration, famciclovir (500 mg 3 times a day for 10 days) is at least as effective as acyclovir (800 mg 5 times daily) in reducing healing time and zoster-associated pain, particularly in those more than or equal to 50 years of age. The fact that the rash started 5 days prior to the start of treatment makes it less likely that the treatment will be effective.
c. How is famciclovir absorbed and metabolized?
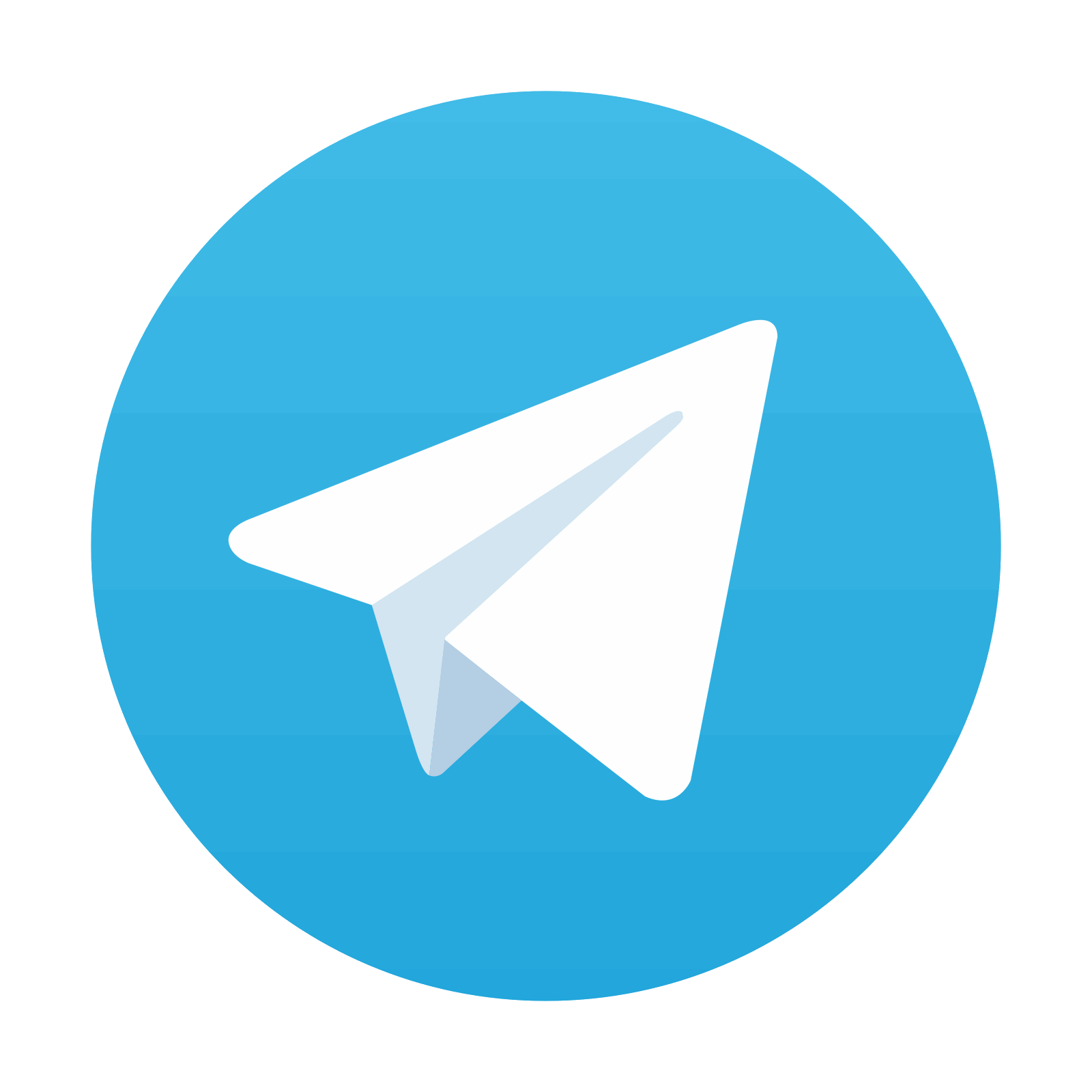
Stay updated, free articles. Join our Telegram channel

Full access? Get Clinical Tree
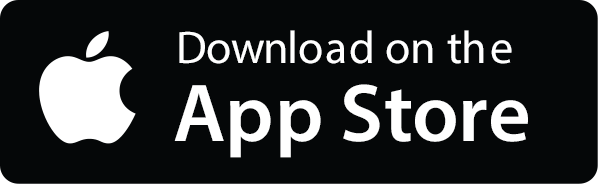
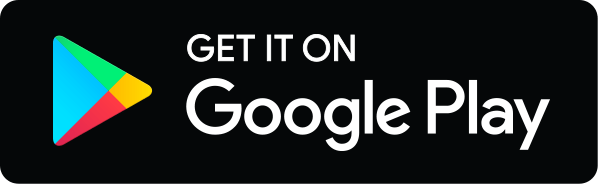