Antimicrobial Dyes
Mark Wainwright
Man’s need to color his environment, and often himself, has been felt since prehistoric times, as might be seen in ancient cave paintings and the tradition, particularly among druidic sects, of staining the skin with the indole pigment woad.1 Whereas self-coloration, in terms of cosmetics, became increasingly popular from the middle ages, especially among women, the medical use of colorants is relatively recent and may be traced back to 19th century Europe and medico-scientists such as Gram, Koch, and Ehrlich who recognized the differential cell-staining potential of the then new aniline dyes when applied to microscopic samples.2 This, in turn allowed Ehrlich’s thesis regarding the medical use of related dyes, particularly in an antimicrobial capacity, with the eventual use of methylene blue (MB) as an antimalarial in 1890 and the introduction by Browning of antiseptic “flavine therapy” during World War I.3 Further research, particularly in postwar Germany, produced new “colored antimicrobials,” which led to the development of a range of conventional drugs against infectious and tropical disease that are still in use.4 However, this productive era was rapidly sidelined, initially by the dye-derived sulfonamide antibacterials and then the penicillins, in the period 1935 to 1945.5 The natural product nature of the original penicillins and streptomycin altered the drug discovery paradigm and further distanced previously useful dyes from the clinic. This situation lasted until perhaps the late 1980s, when conventional drug resistance became a significant clinical problem in Western medicine. At this time, the potential of photoactivated cationic dyes, such as MB, as antimicrobials (“photoantimicrobials”) was revisited and, ironically, they now represent one of the few demonstrably effective methods of circumventing conventional drug resistance. This chapter deals with antimicrobial dyes, both as conventional and photoactivatable agents. Within the chapter, the term dye covers only organic colorants.

Conventionally, dyes are synthesized in order to produce a colored effect on a given substrate—acid dyes on wool, for example. In this way, the desired results are coloration and fastness (ie, permanent adherence to the substrate), neither of which criteria is obviously associated with an antimicrobial effect. Dyes were discovered to have antimicrobial effects as a result, usually, of their chance testing—because, scientifically, this testing would be considered haphazard, rather than structurally systematic. Furthermore, very little scientific method was ever applied to investigating the modes of action of antimicrobial dyes, so that structure-activity relationships were uncommon in the past, the only real example being the sterling work of Albert et al on aminoacridine antibacterials and the establishment of bacterial DNA as a site of action.6
The outcome of almost 130 years of research, thus, does not allow anything approaching activity relationships by chemical class, that is, azoic, triphenylmethane, and so forth because logically, antimicrobial activity arises from direct interactions of chemical groups attached to the molecule—usually at its periphery—with groups or regions within the microbial target. Such groups, for example, quaternary ammonium (-NR3+) or iminium (=NR2+) moieties, are not usually unique to specific dye classes and are, indeed, often interchangeable or even ubiquitous among examples.
Obviously, dye structures vary with class, but the overall constitution is that of a larger, heteroaromatic chromophore (color-forming) portion, often with smaller pendant groups that can alter the chromophoric electron cloud via delocalization, therefore affecting the resulting light absorption profile. Such groups are thus termed auxochromes, a good example of which is the
dimethylaminium group (=NMe2+) found in the phenothiazinium dye MB (as well as many others, including toluidine blue, acridine orange, and neutral red). As noted earlier, in addition to their contribution to molecular light absorption, these groups are often responsible for, at least, the initial interaction with microbial biomolecules such as the lipoteichoic acid in gram-positive bacterial cell wall exteriors.
dimethylaminium group (=NMe2+) found in the phenothiazinium dye MB (as well as many others, including toluidine blue, acridine orange, and neutral red). As noted earlier, in addition to their contribution to molecular light absorption, these groups are often responsible for, at least, the initial interaction with microbial biomolecules such as the lipoteichoic acid in gram-positive bacterial cell wall exteriors.
How different dyes interact with microbial targets depends on several general factors: overall charge, molecular shape, and hydrophilic/lipophilic balance (effectively relative water/oil solubility). For conventional antimicrobial action, this does not of course differ from that of nondye molecules such as antibiotics or biocides. Similarly, how the same dye interacts with different cells can demonstrate morphologic variations, as is seen quite obviously with the triphenylmethane dye crystal (gentian) violet in the Gram stain, differentiating between the two main classes of bacteria by virtue of decreased dye interaction/penetration due to the presence of the outer membrane in gram-negative species.7
From the point of view of antimicrobial action, such variations in interaction with the target(s) are important because decreased interaction suggests weaker activity. It is important, therefore, to understand the processes involved so that activity can be maintained. Much of this understanding has emanated from the renaissance in photoantimicrobial research, which began in the early 1990s, and is based mostly on MB as a lead compound.8 This work has demonstrated the importance of cationic nature for broad-spectrum activity (ie, against gram-positive and gram-negative bacteria) because anionic and electronically neutral dyes have little activity against gram-negative species. The idea of assisted uptake of cationic examples was suggested by Hamblin and Hasan,9 describing the displacement by such dyes of stabilizing magnesium and calcium ions among the head groups in bacterial membranes, leading to membrane defects that allow increased dye ingress and subsequent internal damage.
Dye aggregation is a well-established phenomenon caused by the generally planar nature of such molecules, due to the extended nature of the conjugated pi/aromatic regions essential in dye structures. Aggregation can be envisaged as a kind of plate- or tray-stacking and is, indeed, essential in the visualization of cells by microscopy, that is, aggregation endows intensity of coloration in the cells being stained. Interestingly, dye aggregation is a disadvantage in the far more effective method of cell killing via photodynamic action (see in the following text).
The following sections have been arranged by chemical class because this allows a simpler approach to structure activity but are also discussed under “conventional” and “photoantimicrobial” headings because there are significant differences in levels of range and activity, and thus utility.

Azoic Dyes
Although of little current utility in terms of infection control, azo dyes have a considerable pedigree in terms of the development of conventional drugs and antiseptics. It was, after all, the azo dyes KL695 and prontosil that ushered in what is now considered to be the “antibiotic era” in the 1930s, as a result of a considerable chemical and microbiological effort at the Bayer company premises in Elberfeld.10 The discovery that the azoic structure represented a prodrug, breaking down to sulfanilamide in the mammalian colon, gave rise to the sulfonamide class of antibacterial agents, and it is this class—not the penicillins—that furnished the commencement of useful clinical infection control.10 The continued presence, although in diminishing import, of drugs such as sulfadiazine and sulfamethoxazole is entirely due to Domagk’s screening of azoic dyes, but it is less well appreciated that other related drug classes were developed by him during World War II, such as the thiosemicarbazones with useful activity against mycobacterial infection.11
The demonstrable efficacy of the many sulfanilamide derivatives arising from Bayer’s eventual disclosure of prontosil in 1935 belies the fact that several azoic dyes had been previously reported to have antibacterial activity that did not contain the azobenzenesulfonamide moiety (ie, would not produce sulfanilamide on breakdown).
Azo dyes had, in fact, been suggested and trialed in vitro as antiseptic agents as early as 1913. Excellent results had previously been reported for the simple dye chrysoidine by Eisenberg12 and for pyridium and serenium by Ostromislensky.13 The latter two were still in use as urinary antiseptics in the 1930s,14 and pyridium remains in use.15 Although such results were not observed in Bayer’s animal testing,16 the structural similarity to the prontosil rubrum molecule is obvious, but the lack of a sulfonamide residue (Figure 13.1) suggests that the mode of antibacterial action is different.
Triarylmethanes
It can be argued that the first major impact of dyes as antimicrobial agents was, in fact, the use of the triarylmethane derivative crystal (gentian) violet (Figure 13.2) in
Hans-Christian Gram’s development of bacterial typing. Although this immensely valuable work was not aimed at bacterial killing, the demonstrated dye-cell interactions and selectivity underpinned the ensuing labors of scientists such as Koch and Ehrlich and led, ultimately, to the development of antimicrobial chemotherapy.1 As noted earlier, “flavine therapy” was introduced by Browning and Gulbransen3 during the second half of World War I, “flavines” referring generally to the dyes employed for wound disinfection, rather than being chemically specific. One of the principal dyes used here was brilliant green, a closely related analogue of crystal violet (see Figure 13.2). Similar work was carried out at the same time by others.17,18,19,20
Hans-Christian Gram’s development of bacterial typing. Although this immensely valuable work was not aimed at bacterial killing, the demonstrated dye-cell interactions and selectivity underpinned the ensuing labors of scientists such as Koch and Ehrlich and led, ultimately, to the development of antimicrobial chemotherapy.1 As noted earlier, “flavine therapy” was introduced by Browning and Gulbransen3 during the second half of World War I, “flavines” referring generally to the dyes employed for wound disinfection, rather than being chemically specific. One of the principal dyes used here was brilliant green, a closely related analogue of crystal violet (see Figure 13.2). Similar work was carried out at the same time by others.17,18,19,20
Interestingly, Fung and Miller21 examined the effects of a range of dyes—42 in all—from different classes and observed a distinct difference in activity along Gram classification lines, with very little activity shown against gram-negative bacteria but high activity by over half of the examples used against gram-positive bacteria. Little modern work has focused on this class of agents in terms of new and improved derivatives. Crystal violet was also found to be active against fungi and was used until relatively recently against skin infections such as ringworm.22 In a parallel field, the dye was, until recently, added to donated blood by South American agencies to inactivate the parasite responsible for Chagas disease, Trypanosoma cruzi.23 Additionally, crystal violet has proved to be highly effective in the treatment of patients infected with methicillin-resistant Staphylococcus aureus (MRSA).24
Acridines
The use of acridines as antimicrobial agents was first proposed25 following the clinical introduction of proflavine and acriflavine as wound disinfectants3 in 1917; several other derivatives were introduced during the World War II, mainly due to the work of Albert et al26 and including the antimalarial drug mepacrine. Albert et al’s efforts in this area allowed the development of structure-activity ideas, indicating that the microbial target was DNA and that active acridines intercalated into the structure. However, the interaction of quinolone antibacterials27 and some acridine-based anticancer drugs28 with DNA-managing enzymes such as gyrases and topoisomerases suggests that alternative/additional modes of action are possible. Albert et al’s experimentally derived criteria for antibacterial activity among the acridines, to include their cationic nature, high levels of ionization at physiological pH, and a planar molecular surface area ≥38 Å2, led to the discovery that the heteroaromatic chromophore could be varied, or that a purely benzenoid structure was equally effective.29 Thus, activity became predictable (or explainable) not only in the related azine dyes (phenazines, phenoxazines, phenothiazines, etc) but also in cationic anthracene derivatives.29 Furthermore, nonlinear ring fusion (as in the phenanthridines) or bridged structures, where the bridge is part of the delocalized pi system of the molecule (eg, styryl and cyanine derivatives), also furnished active examples.
In terms of the clinical use of acridine derivatives, the principal entries are provided in Table 13.1.
In antiseptic and disinfection applications, the main acridine derivatives used have been proflavine, acriflavine/euflavine, and aminacrine, although local use of these agents was rapidly superseded by systemic β-lactam administration in the mid-1940s. Local application, for example, in wound disinfection, continued for a considerable time, both alone and in combination with sulfonamide derivatives.31 In addition, acriflavine, the quaternary 10-methyl derivative of proflavine containing varying levels of proflavine hydrochloride, had been used as a systemic therapy in cases of trypanosomiasis (as trypaflavin) and gonorrhoea (as gonaflavin), the latter being administered intravenously at 0.1 g 3 times per week,32 or in 10 doses of 40 to 80 mg at 2- to 3-day intervals.33 The pure quaternary compound is euflavine (see Table 13.1).
The aqueous solubility of aminoacridine derivatives is obviously an important parameter in the proposed use of such compounds as injectable antibacterials, that is, for systemic use. The hydrophilic nature of the simple aminoacridines covered here furnishes correspondingly short half-lives in the bloodstream, and their use in the clinical treatment of bloodborne infection, for example, staphylococcal septicemia, has never been a realistic option as a consequence. For example, intravenous treatment of septicemia with argoflavin, a mixture of euflavine lactate and silver lactate, was not usually successful,34 the concentration in the blood of administered acriflavine (200 mg) having decreased by 90% over 5 minutes and being undetectable at 30 minutes.35 Aminacrine and rivanol exhibited similar pharmacokinetics.36,37 Despite the absence of currently suitable injectables, there is a considerable patent literature, particularly concerning quaternized acridines, which emanated from earlier work on euflavine. However, simple aminoacridines were synthesized as blood antimicrobials, notably by IG Farben, for example, 3-amino-10-methyl-6-haloacridinium species.38,39,40
Further forays in this area might well use the antimalarial acridine mepacrine (quinacrine, atebrin) as a lead compound from a pharmacologic standpoint, with alterations to the much longer C-9 side chains, for example,
with Nitroakridin 3582. This compound, [2,3-dimethoxy-6-nitro-9-(3′-diethylamino-2′-hydroxypropylamino) acridine], has been used in the treatment of clinical typhus.41 The works of Steck et al42 on antirickettsial acridines, based on Nitroakridin 3582, and of Elslager and Tendick43 on acridine N-oxides represent the last major research efforts in systemic acridine antibacterials. Tabern’s44,45 “Phenacridane” series was intended as a topical anti-infective, and this has indeed been the major area of acridine-based antibacterial treatment since that time, mainly using proflavine or acriflavine.
with Nitroakridin 3582. This compound, [2,3-dimethoxy-6-nitro-9-(3′-diethylamino-2′-hydroxypropylamino) acridine], has been used in the treatment of clinical typhus.41 The works of Steck et al42 on antirickettsial acridines, based on Nitroakridin 3582, and of Elslager and Tendick43 on acridine N-oxides represent the last major research efforts in systemic acridine antibacterials. Tabern’s44,45 “Phenacridane” series was intended as a topical anti-infective, and this has indeed been the major area of acridine-based antibacterial treatment since that time, mainly using proflavine or acriflavine.
TABLE 13.1 Clinical antibacterial acridinesa | |||||||||||||||||||||||||||||||||||||||||||||||||||||||||||||||||||||||||||||||||||||||||||
---|---|---|---|---|---|---|---|---|---|---|---|---|---|---|---|---|---|---|---|---|---|---|---|---|---|---|---|---|---|---|---|---|---|---|---|---|---|---|---|---|---|---|---|---|---|---|---|---|---|---|---|---|---|---|---|---|---|---|---|---|---|---|---|---|---|---|---|---|---|---|---|---|---|---|---|---|---|---|---|---|---|---|---|---|---|---|---|---|---|---|---|
|
The use of orally administered acridine drugs has been much more common, for example, euflavine pastilles employed for throat disinfection (Panflavine or Planacrine brands). Gonorrhoea was also treated via the oral route using acriflavine.46 Ethacridine (2-ethoxy-6,9-diaminoacridine; see Table 13.1) has found long-term use in the oral treatment of enteric disease such as shigellosis due to low absorption and lower toxicity than acriflavine.36,47
Phenazines
Heteroatomic replacement of the C9 carbon of the acridine nucleus with nitrogen furnishes the phenazine analogue, responsible for biological stains such as neutral red and safranin, among others. Although a range of phenazines was included in Browning’s examination of a range of available dyes for antimicrobial activity in the post-World War I period,48 no significant activity was reported. However, clofazimine, a phenazine-containing molecule, has formed part of the antimycobacterial effort since the 1950s. Clofazimine (Figure 13.3) was originally synthesized in the search for drugs against Hansen disease.49
Clofazimine is a purple- or damson-colored compound. Although, as noted, Barry’s original drug target was Hansen bacillus (Mycobacterium leprae),50 it is also active against Mycobacterium avium, one of the opportunistic pathogens associated with human immunodeficiency virus (HIV)-positive status.51 Clofazimine has also found use in the treatment of Crohn disease52 and in drug-resistant tuberculosis.53
Cyanine and Styryl Dyes
Research in this area grew out of the success of flavine therapy during World War I and was due, in the most part, to Carl Browning, a one-time assistant of Paul Ehrlich.54 Browning and his main chemical collaborator, Cohen, deconstructed the acridine chromophore and thus experimented on amino derivatives of pyridines and quinolines with olefinic groups attached in partial replacement of the fused benzene rings in acridine, producing both cyanine- and styryl-type molecules. As Browning was interested both in bacterial and trypanosomal infection, the resulting compounds were tested against both types of pathogen, producing a considerable body of work. In addition, styryl (aryl-CH=CH-aryl) and imino (aryl-CH=N-aryl) derivatives were examined.48,55,56,57,58,59
The activity of several of the derivatives was such that a clinical trial was run with compound 48S (Table 13.2) at the General Infirmary in Leeds, United Kingdom,61 and included more than 2000 subjects. The 48S was employed in most areas requiring antisepsis/antiseptic dressings, local application, wound or infection site irrigation, and even intravenous administration in septicemia. Due to the instability of imino structures in acidic solution where hydrolysis occurs, 48S was not administered by mouth. Generally, the activity of 48S in human subjects was sufficient to give cures in most cases where it was applied directly rather than systemically, although some successful treatments of septicemia were reported. In many ways, 48S appears to be similar in activity to acriflavine and proflavine, although these are not effective in blood-borne disease. That the clinical trial of the iminoquinoline was not extended cannot be due to lack of efficacy, and it was described as being bland and nonirritating to the tissues. As can be seen from Table 13.2, the variation in amino side chain length produced considerable differences in activity, longer chain derivatives generally being more active, in many cases more so than 48S itself. This may indicate a “lipophilic tail” influence due to interaction with outer/inner membrane structures in the bacterial target.
TABLE 13.2 Antibacterial activity of analogues of clinical compound 48, provided in µmol (10-6 M)a | |||||||||||||||||||||||||||||||||||||||||||||
---|---|---|---|---|---|---|---|---|---|---|---|---|---|---|---|---|---|---|---|---|---|---|---|---|---|---|---|---|---|---|---|---|---|---|---|---|---|---|---|---|---|---|---|---|---|
|
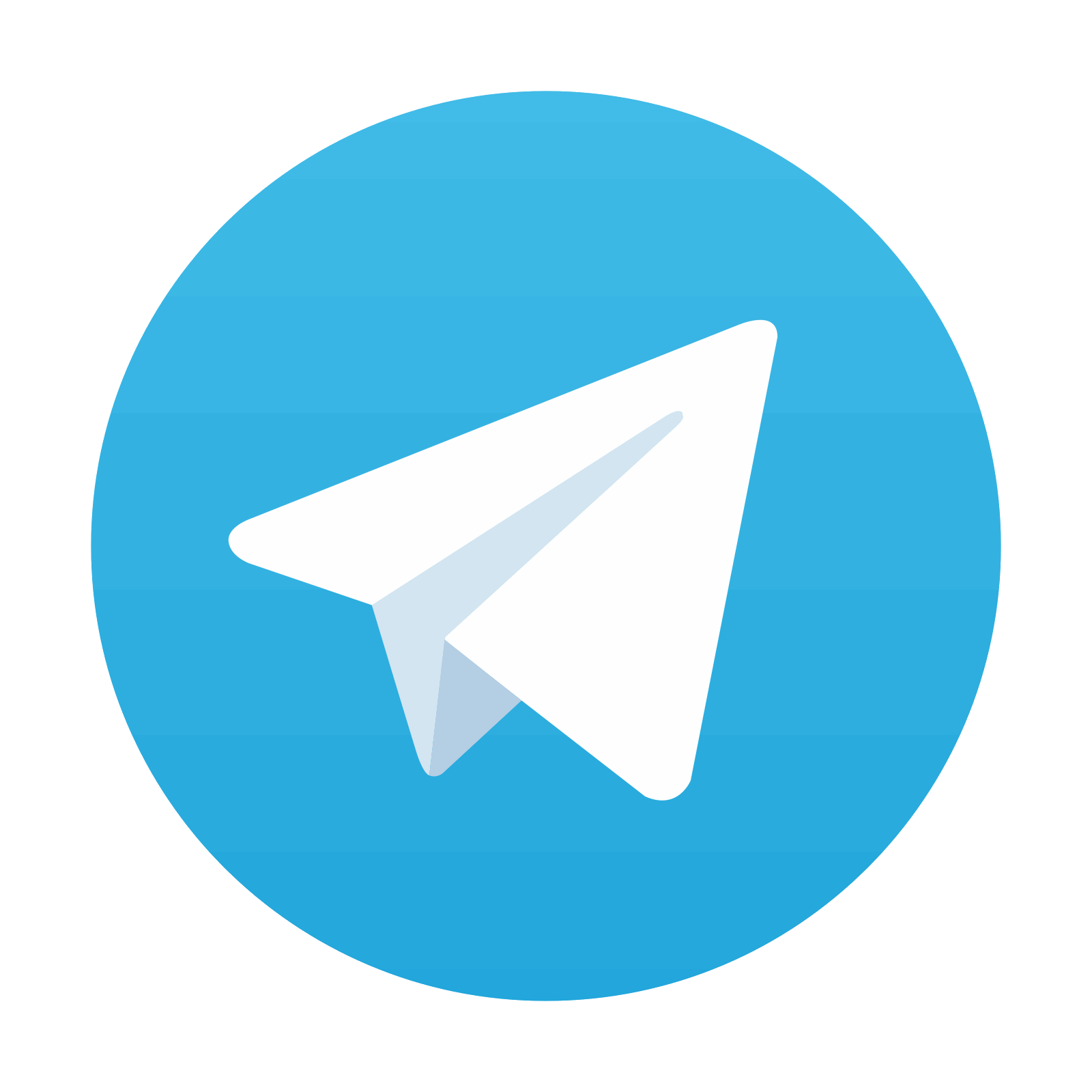
Stay updated, free articles. Join our Telegram channel

Full access? Get Clinical Tree
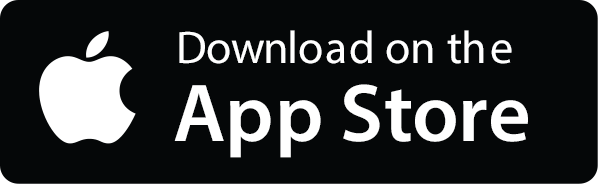
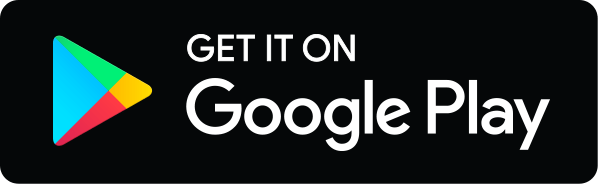