
Antigen receptors serve critical roles in the maturation of lymphocytes from progenitors and in all adaptive immune responses. In adaptive immunity, naive lymphocytes recognize antigens to initiate responses, and effector T cells and antibodies recognize antigens to perform their functions.
B and T lymphocytes express different receptors that recognize antigens: membrane-bound antibodies on B cells and T cell receptors (TCRs) on T lymphocytes. The principal function of cellular receptors in the immune system, as in other biologic systems, is to detect external stimuli and trigger responses of the cells on which the receptors are expressed. To recognize a large variety of different antigens, the antigen receptors of lymphocytes must be able to bind to and distinguish between many, often closely related, chemical structures. Antigen receptors are clonally distributed, meaning that each lymphocyte clone is specific for a distinct antigen and has a unique receptor, different from the receptors of all other clones. (Recall that a clone consists of a parent cell and its progeny.) The total number of distinct lymphocyte clones is very large, and this entire collection makes up the immune repertoire . Although each clone of B lymphocytes or T lymphocytes recognizes a different antigen, the antigen receptors transmit biochemical signals that are fundamentally the same in all lymphocytes and are unrelated to specificity. These features of lymphocyte recognition and antigen receptors raise the following questions:
- •
How do the antigen receptors of lymphocytes recognize extremely diverse antigens and transmit activating signals to the cells?
- •
What are the differences in the recognition properties of antigen receptors on B cells and T cells?
- •
How is the vast diversity of receptor structures in the lymphocyte repertoire generated? The diversity of antigen recognition implies the existence of many structurally different antigen receptor proteins, more than can be encoded in the inherited genome (germline). Therefore, special mechanisms must exist for generating this diversity.
In this chapter, we describe the structures of the antigen receptors of B and T lymphocytes and how these receptors recognize antigens. We also discuss how the diversity of antigen receptors is generated during the process of lymphocyte development, thus giving rise to the repertoire of mature lymphocytes. The process of antigen-induced lymphocyte activation is described in later chapters.
Antigen Receptors of Lymphocytes
The antigen receptors of B and T lymphocytes have several features that are important for their functions in adaptive immunity ( Fig. 4.1 ). Although these receptors have many similarities in terms of structure and mechanisms of signaling, there are fundamental differences related to the types of antigenic structures that B cells and T cells recognize.
- •
Membrane-bound antibodies, which serve as the antigen receptors of B lymphocytes, can recognize many types of chemical structures, while T cell antigen receptors recognize only peptides bound to major histocompatibility complex (MHC) molecules. B lymphocyte antigen receptors and the antibodies that B cells secrete can recognize the shapes, or conformations, of macromolecules, including proteins, lipids, carbohydrates, and nucleic acids, as well as simpler, smaller chemical moieties. This broad specificity of B cells for structurally different types of molecules in their native form enables the humoral immune system to recognize, respond to, and eliminate diverse microbes and toxins. In striking contrast, T cells see only peptides displayed on antigen-presenting cells (APCs) bound to MHC molecules. This specificity ensures that T cells never interact with free or soluble antigens and that they only interact with microbial or tumor antigens present inside other cells in the body.
- •
Antigen receptor molecules consist of regions (domains) involved in antigen recognition—therefore varying between clones of lymphocytes—and other regions required for structural integrity and effector functions—thus relatively conserved among all clones. The antigen-recognizing domains of the receptors are called variable (V) regions, and the conserved portions are the constant (C) regions. Even within each V region, most of the sequence variation is concentrated within short stretches, which are called hypervariable regions, or complementarity-determining regions (CDRs), because they form the parts of the receptor that bind antigens (i.e., they are complementary to the shapes of antigens). By concentrating sequence variation in small regions of the receptor, it is possible to maximize the variability of the antigen-binding part while retaining the basic structure of the receptors. As discussed later, special mechanisms exist in developing lymphocytes to create genes that encode different variable regions of antigen receptor proteins in individual clones.
- •
Antigen receptor chains are associated with invariant membrane proteins whose function is to deliver intracellular signals following antigen recognition (see Fig. 4.1 ). These signals, which are transmitted to the cytosol and the nucleus, may cause a lymphocyte to divide, to differentiate, to perform effector functions, or in certain circumstances to die. Thus, the two functions of lymphocyte receptors for antigen—specific antigen recognition and signal transduction—are mediated by different polypeptides. This again allows variability to be segregated in one set of molecules—the antigen receptors themselves—while leaving the conserved function of signal transduction to the other invariant proteins. The set of plasma membrane antigen receptor and signaling molecules in B lymphocytes is called the B cell receptor (BCR) complex, and in T lymphocytes it is called the T cell receptor (TCR) complex. When antigens bind to the extracellular portions of the antigen receptors of lymphocytes, intracellular portions of the associated signaling proteins are phosphorylated on conserved tyrosine residues by enzymes called protein tyrosine kinases. Phosphorylation triggers complex signaling cascades that culminate in the transcriptional activation of many genes and the production of numerous proteins that mediate the responses of the lymphocytes. We return to the processes of T and B lymphocyte activation in Chapter 5 , Chapter 7 , respectively.
Fig. 4.1
Properties of antibodies and T cell antigen receptors (TCRs).
Antibodies (also called immunoglobulins) may be expressed as membrane receptors or secreted proteins; TCRs only function as membrane receptors. When immunoglobulin (Ig) or TCR molecules recognize antigens, signals are delivered to the lymphocytes by proteins associated with the antigen receptors. The antigen receptors and attached signaling proteins form the B cell receptor (BCR) and TCR complexes. Note that single antigen receptors are shown recognizing antigens, but signaling typically requires the binding of two or more receptors to adjacent antigen molecules. The important characteristics of these antigen-recognizing molecules are summarized. ∗The total number of possible receptors with unique binding sites is very large, but only ~10 7 –10 9 clones with distinct specificities are present in adults. APCs, Antigen-presenting cells; Ig, immunoglobulin; MHC, major histocompatibility complex.
- •
Antibodies exist in two forms—as membrane-bound antigen receptors on B cells and as secreted proteins—but TCRs exist only as membrane receptors on T cells. Secreted antibodies are present in the blood and mucosal secretions, where they provide protection against microbes (i.e., they are the effector molecules of humoral immunity). Antibodies are also called immunoglobulins (Igs), referring to immunity-conferring proteins with the physical characteristics of globulins. Secreted antibodies recognize microbial antigens and toxins by their variable domains, the same as the membrane-bound antigen receptors of B lymphocytes. The constant regions of some secreted antibodies have the ability to bind to other molecules that participate in the elimination of antigens: these molecules include receptors on phagocytes and proteins of the complement system. Thus, antibodies serve different functions at different stages of humoral immune responses: membrane-bound antibodies on B cells recognize antigens to initiate B cell activation, and secreted antibodies neutralize and eliminate microbes and their toxins in the effector phase of humoral immunity. In cell-mediated immunity, the effector function of microbe elimination is performed by T lymphocytes themselves and by other leukocytes responding to the T cells. The antigen receptors of T cells are involved only in antigen recognition and T cell activation, and these proteins are not secreted and do not mediate effector functions.
With this introduction, we describe next the antigen receptors of lymphocytes, first antibodies and then TCRs.
Antibodies
An antibody molecule is composed of four polypeptide chains—two identical heavy (H) chains and two identical light (L) chains—with each chain containing a variable region and a constant region ( Fig. 4.2 ). The four chains are assembled to form a Y -shaped molecule. Each light chain is attached to one heavy chain, and the two heavy chains are attached to each other, all by disulfide bonds. A light chain is made up of one V and one C domain, and a heavy chain has one V and three or four C domains. Each domain folds into a characteristic three-dimensional shape, called the immunoglobulin (Ig) domain (see Fig. 4.2D ). An Ig domain consists of two layers of a β-pleated sheet held together by a disulfide bridge. The adjacent strands of each β-sheet are connected by short, protruding α-helical loops; in the V regions of Ig molecules, three of these loops make up the three CDRs responsible for antigen recognition. Ig domains without hypervariable loops are present in many other proteins in the immune system, as well as outside the immune system, and most of these proteins are involved in responding to stimuli from the environment and from other cells. All of these proteins are said to be members of the immunoglobulin superfamily.

The antigen-binding site of an antibody is composed of the V regions of both the heavy chain and the light chain, and the core antibody structure contains two identical antigen binding sites (see Fig. 4.2 ). Each variable region of the heavy chain (called V H ) or of the light chain (called V L ) contains three hypervariable regions, or CDRs. Of these three, the greatest variability is in CDR3, which is located at the junction of the V and C regions. As may be predicted from this variability, CDR3 is also the portion of the Ig molecule that contributes most to antigen binding.
Functionally distinct portions of antibody molecules were first identified based on proteolysis, which generated fragments that were composed of different parts of antibody proteins. The fragment of an antibody that contains a whole light chain (with its single V and C domains) attached to the V and first C domains of a heavy chain is required for antigen recognition and is therefore called the Fab (fragment, antigen-binding) region. The remaining heavy-chain C domains make up the Fc (fragment, crystalline) region; because this fragment is identical in all antibody molecules of a particular type, it tends to crystallize in solution. In each Ig molecule, there are two identical Fab regions that bind antigen attached to one Fc region that is responsible for most of the biologic activity and effector functions of the antibodies. (As discussed later, some types of antibodies exist as multimers of two or five Ig molecules attached to one another.) Linking the Fab and Fc regions of most antibody molecules is a flexible portion called the hinge region. The hinge allows the two antigen-binding Fab regions of each antibody molecule to move independent of each other, enabling them to simultaneously bind antigen epitopes that are separated from one another by varying distances.
The C-terminal end of the heavy chain may be anchored in the plasma membrane, as seen in BCRs, or it may terminate in a tail piece that lacks the membrane anchor so that the antibody is produced as a secreted protein. Light chains in Ig molecules are not directly attached to cell membranes.
There are five types of Ig heavy chains, called μ, δ, γ, ε, and α, which differ in their C regions; in humans, there are four subtypes of γ chain, called γ1, γ2, γ3, γ4, and two of the α chain, called α1 and α2. Antibodies that contain different heavy chains belong to different classes , or isotypes , and are named according to their heavy chains (IgM, IgD, IgG, IgE, and IgA). Each isotype has distinct physical and biologic properties and effector functions ( Fig. 4.3 ). The IgG subtypes also differ from one another in functional properties, but the IgA subtypes do not. The antigen receptors of naive B lymphocytes, which are mature B cells that have not encountered antigen, are membrane-bound IgM and IgD. After stimulation by antigen and helper T lymphocytes, the antigen-specific B lymphocyte clone may expand and differentiate into progeny that secrete antibodies. Some of the progeny of IgM and IgD expressing B cells may secrete IgM, and other progeny of the same B cells may produce antibodies of other heavy-chain classes. This change in Ig isotype production is called heavy-chain class (or isotype ) switching ; its mechanism and importance are discussed in Chapter 7 .

The two types of light chains, called κ and λ, differ in their C regions. Each antibody has only κ or λ light chains, but not both, and all the antibodies made by any B cell have the same type of light chain. Each type of light chain may complex with any type of heavy chain in an antibody molecule. The light-chain class (κ or λ) also remains fixed throughout the life of each B cell clone, regardless of whether or not heavy-chain class switching has occurred. The function of light chains is to form the antigen-binding surface of antibodies, along with the heavy chains; light chains do not participate in effector functions, except binding and neutralizing microbes and toxins.
Binding of Antigens to Antibodies
Antibodies are capable of binding a wide variety of antigens, including macromolecules and small chemicals. The reason for this is that the antigen-binding CDR loops of antibody molecules can either come together to form clefts capable of accommodating small molecules or form more extended surfaces capable of accommodating larger molecules ( Fig. 4.4 ). Antibodies bind to antigens by reversible, noncovalent interactions, including hydrogen bonds, hydrophobic interactions, and charge-based interactions. The parts of antigens that are recognized by antibodies are called epitopes , or determinants. Some epitopes of protein antigens may be a contiguous stretch of amino acids in the primary structure of the protein; these are called linear epitopes. Sometimes, amino acids that are not next to one another in the primary structure may be brought into proximity when the protein folds, forming a distinct shape that is recognized by an antibody; such determinants are called conformational epitopes.

The strength with which one antigen-binding site of an antibody binds to one epitope of an antigen is called the affinity of the interaction. Affinity often is expressed as the dissociation constant (K d ), which is the molar concentration of an antigen required to occupy half the available antibody molecules in a solution; the lower the K d , the higher the affinity. Most antibodies produced in a primary immune response have a K d in the range of 10 −6 to 10 −9 M, but with repeated stimulation (e.g., in a secondary immune response), the affinity increases to a K d of 10 −8 to 10 −11 M. This increase in antigen-binding strength is called affinity maturation (see Chapter 7 ).
Each IgG, IgD, and IgE antibody molecule has two antigen-binding sites. Secreted IgA is a dimer of two linked IgA molecules and therefore has four antigen-binding sites, and secreted IgM is a pentamer, with 10 antigen-binding sites. Therefore, each antibody molecule can bind 2 to 10 epitopes of an antigen, or epitopes on two or more neighboring antigens. The total strength of binding is much greater than the affinity of a single antigen-antibody bond and is called the avidity of the interaction. Antibodies produced against one antigen may bind other, structurally similar antigens. Such binding to similar epitopes is called a cross-reaction .
In B lymphocytes, membrane-bound Ig molecules are noncovalently associated with two other proteins, called Igα and Igβ; these latter proteins combine with the membrane Ig to make up the BCR complex. When the BCR recognizes antigen, Igα and Igβ transmit signals to the interior of the B cell that initiate the process of B cell activation. These and other signals in humoral immune responses are discussed in Chapter 7 .
Monoclonal Antibodies
The realization that one clone of B cells makes an antibody of only one specificity has been exploited to produce monoclonal antibodies , one of the most important technical advances in immunology, with far-reaching implications for clinical medicine and research. To produce monoclonal antibodies, B cells, which have a short life span in vitro, are obtained from an animal immunized with an antigen and fused in vitro with myeloma cells (tumors of plasma cells), which can be propagated indefinitely in tissue culture ( Fig. 4.5 ). The myeloma cell line lacks a specific enzyme, as a result of which these cells cannot grow in the presence of a certain toxic drug; fused cells, containing both myeloma and normal B cell nuclei, however, do grow in the presence of this drug because the normal B cells provide the missing enzyme. Thus, by fusing the two cell populations and culturing them with the drug, it is possible to grow out fused cells that are hybrids of the B cells and the myeloma, and are called hybridomas . These hybridoma cells produce antibodies, like normal B cells, but grow continuously, having acquired the immortal property of the myeloma tumor. From a population of hybridomas, one can select and expand individual cells that secrete the antibody of desired specificity; such antibodies, derived from a single B cell clone, are homogeneous monoclonal antibodies. Monoclonal antibodies against virtually any epitope on any antigen can be produced using this technology.

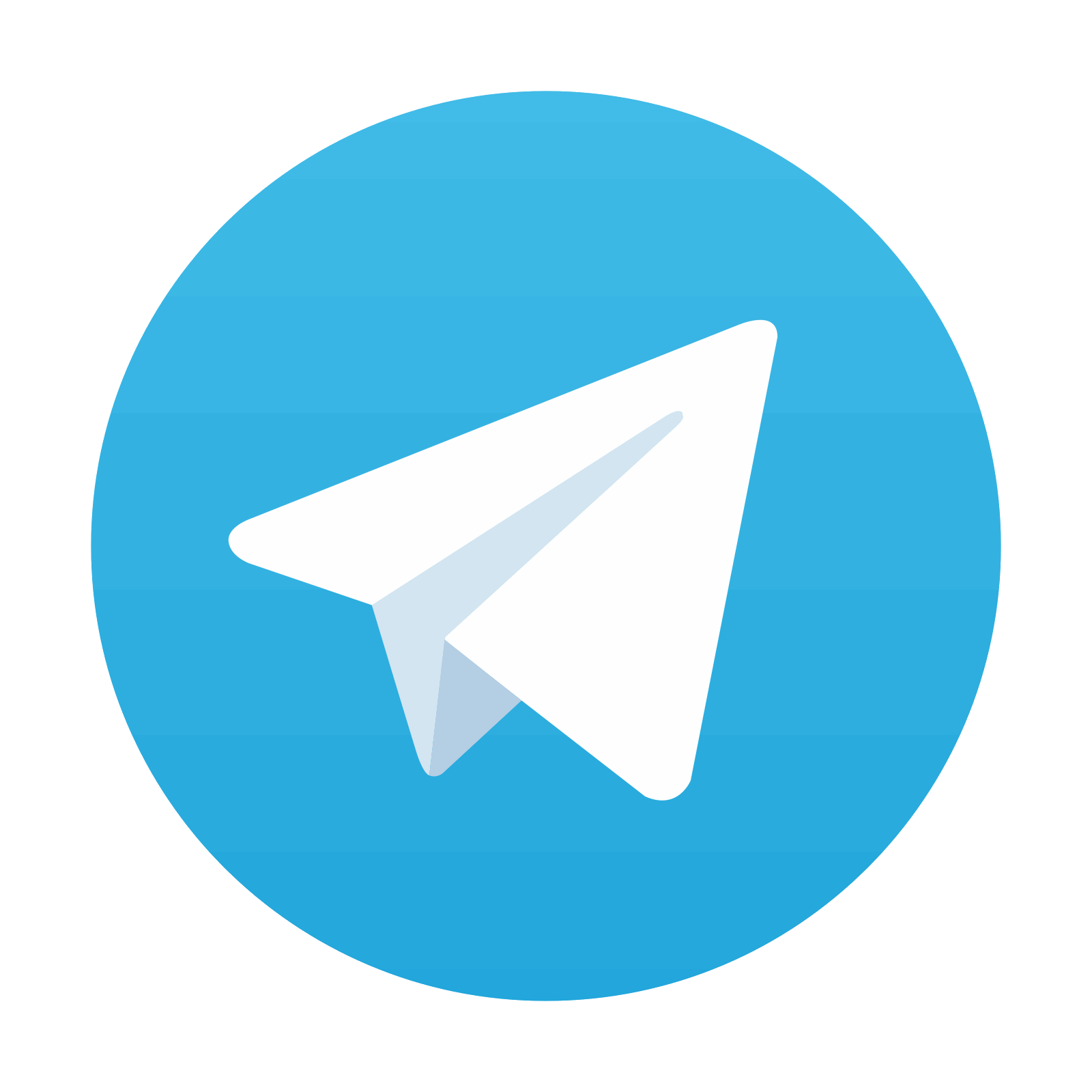
Stay updated, free articles. Join our Telegram channel

Full access? Get Clinical Tree
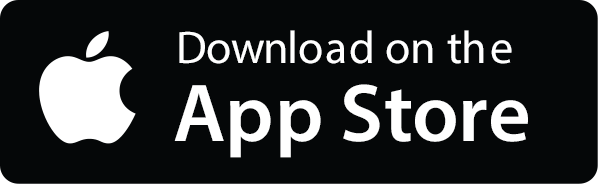
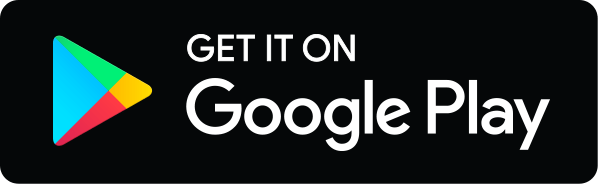
