
Adaptive immune responses are initiated by the recognition of antigens by antigen receptors of lymphocytes. B and T lymphocytes differ in the types of antigens they recognize. The antigen receptors of B lymphocytes—namely, membrane-bound antibodies—can recognize a variety of macromolecules (proteins, polysaccharides, lipids, nucleic acids), in soluble form or cell surface–associated form, as well as small chemicals. Therefore, B cell–mediated humoral immune responses may be generated against many types of microbial cell wall and soluble antigens. The antigen receptors of most T lymphocytes, on the other hand, can see only peptide fragments of protein antigens, and only when these peptides are displayed on host cell surfaces bound to specialized proteins called major histocompatibility complex (MHC) molecules. Because the association of antigenic peptides and MHC molecules occurs inside cells, T cell–mediated immune responses may be generated only against protein antigens that are either produced in or taken up by host cells. This chapter focuses on the nature of the antigens that are recognized by lymphocytes. Chapter 4 describes the receptors used by lymphocytes to detect these antigens.
The induction of immune responses by antigens is a highly orchestrated process with a number of remarkable features. The first is that very few naive lymphocytes are specific for any one antigen, as few as 1 in 10 5 or 10 6 circulating lymphocytes, and this small fraction of the body’s lymphocytes needs to locate and react rapidly to the antigen, wherever it is introduced. Second, different types of adaptive immune responses are required to defend against different types of microbes. In fact, the immune system has to react in different ways even to the same microbe at different stages of the microbe’s life cycle. For example, defense against a microbe (e.g., a virus) that has entered the bloodstream depends on antibodies that bind the microbe, prevent it from infecting host cells, and help to eliminate it. The production of potent antibodies requires the activation of CD4 + helper T cells. After it has infected host cells, however, the microbe is safe from antibodies, which cannot enter the cells. As a result, activation of CD8 + cytotoxic T lymphocytes (CTLs) may be necessary to kill the infected cells and eliminate the reservoir of infection. Thus, we are faced with two important questions:
- •
How do the rare naive lymphocytes specific for any microbial antigen find that microbe, especially considering that microbes may enter anywhere in the body?
- •
How do different types of T cells recognize microbes in different cellular compartments? Specifically, helper T cells recognize and respond to both extracellular and intracellular microbes that can be internalized into vesicular compartments in host cells, whereas CTLs kill infected cells that harbor microbial antigens in the cytosol and nucleus outside vesicular compartments. As we shall see in this chapter, MHC molecules play a central role in this segregation of antigen recognition by T cells.
The answer to both questions is that the immune system has developed a highly specialized system for capturing and displaying antigens to lymphocytes. Research by immunologists, cell biologists, and biochemists has led to a sophisticated understanding of how protein antigens are captured, broken down, and displayed for recognition by T lymphocytes. This is the major topic of discussion in this chapter.
Antigens Recognized by T Lymphocytes
The majority of T lymphocytes recognize peptide antigens that are bound to and displayed by the MHC molecules of antigen-presenting cells (APCs). The MHC is a genetic locus whose principal protein products function as the peptide display molecules of the immune system. CD4 + and CD8 + T cells can see peptides only when these peptides are displayed by that individual’s MHC molecules. This property of T cells is called MHC restriction . The T cell receptor (TCR) recognizes some amino acid residues of the peptide antigen and simultaneously also recognizes residues of the MHC molecule that is displaying that peptide ( Fig. 3.1 ). Each TCR, and hence each clone of CD4 + or CD8 + T cells, recognizes one peptide displayed by one of the many MHC molecules in every individual. The properties of MHC molecules and the significance of MHC restriction are described later in this chapter. How we generate T cells that recognize peptides presented only by self MHC molecules is described in Chapter 4 . Also, some small populations of T cells recognize lipid and other nonpeptide antigens either presented by nonpolymorphic class I MHC–like molecules or without a requirement for a specialized antigen display system.

The cells that capture microbial antigens and display them for recognition by T lymphocytes are called antigen-presenting cells (APCs). Naive T lymphocytes must see protein antigens presented by dendritic cells to initiate clonal expansion and differentiation of the T cells into effector and memory cells. Differentiated effector T cells again need to see antigens, which may be presented by various kinds of APCs besides dendritic cells, to activate the effector functions of the T cells in both humoral and cell-mediated immune responses. We first describe how APCs capture and present antigens to trigger immune responses and then examine the role of MHC molecules in antigen presentation to T cells.
Capture of Protein Antigens by Antigen-Presenting Cells
Protein antigens of microbes that enter the body are captured mainly by dendritic cells and concentrated in the peripheral (secondary) lymphoid organs, where immune responses are initiated ( Fig. 3.2 ). Microbes usually enter the body through the skin (by contact), the gastrointestinal tract (by ingestion), the respiratory tract (by inhalation), and the genitourinary tract (by sexual contact). Some microbes may enter the bloodstream. Microbial antigens can also be produced in any infected tissue. Because of the vast surface area of the epithelial barriers and the large volume of blood, connective tissues, and internal organs, it would be impossible for lymphocytes of all possible specificities to efficiently patrol all these sites searching for foreign invaders; instead, antigens are taken to the lymphoid organs through which lymphocytes recirculate.

Antigens are taken to peripheral lymphoid organs in two ways.
- •
Microbes or their antigens may enter the lymph or blood and circulate to lymph nodes or spleen, respectively, where they are captured by resident dendritic cells and presented to T cells. Other APCs may also capture antigens and display them to B cells in these organs.
- •
Dendritic cells in epithelia, connective tissues, and organs transport microbial antigens to lymphoid organs. This process involves a series of events following the encounter of dendritic cells with microbes—capture of antigens, activation of the dendritic cells, migration of the antigen-carrying cells to lymph nodes, and display of the antigen to T cells. These steps are described next.
All the interfaces between the body and the external environment are lined by continuous epithelia, which provide barriers to infection. The epithelia and subepithelial tissues contain a network of cells with long processes, called dendritic cells ; these cells are also present in the T cell–rich areas of peripheral lymphoid organs and, in smaller numbers, in most other organs ( Fig. 3.3 ). There are two major populations of dendritic cells, called conventional (or classical) and plasmacytoid, which differ in their locations and responses ( Fig. 3.4 ). The majority of dendritic cells in tissues and lymphoid organs belong to the classical subset. In the skin, the epidermal dendritic cells are called Langerhans cells. Plasmacytoid dendritic cells are named because of their morphologic resemblance to plasma cells; they are present in the blood and tissues. Plasmacytoid dendritic cells are also the major source of type I interferons in innate immune responses to viral infections (see Chapter 2 ).


Dendritic cells use various membrane receptors to bind microbes, such as cell surface lectins that recognize carbohydrate structures typical of microbial but not mammalian glycoproteins. These microbes or their antigens are taken up by dendritic cells by phagocytosis or receptor-mediated endocytosis. At the same time that the dendritic cells are capturing antigens, products of the microbes stimulate innate immune reactions by binding to Toll-like receptors (TLRs) and to other innate pattern-recognition receptors in the dendritic cells, tissue epithelial cells, and resident macrophages (see Chapter 2 ). This results in the production of inflammatory cytokines such as tumor necrosis factor (TNF) and interleukin-1 (IL-1). The combination of innate receptor signaling and cytokines activates the dendritic cells, resulting in several changes in their phenotype, migration, and function.
Upon activation, classical dendritic cells lose their adhesiveness for epithelia and begin to express the chemokine receptor CCR7, which is specific for chemoattracting cytokines (chemokines) produced by lymphatic endothelium and by stromal cells in the T cell zones of lymph nodes. These chemokines direct the dendritic cells to exit the epithelium and migrate through lymphatic vessels to the lymph nodes draining that epithelium ( Fig. 3.5 ). During the process of migration, the dendritic cells mature from cells designed to capture antigens into APCs capable of stimulating T lymphocytes. This maturation is reflected by increased synthesis and stable expression of MHC molecules, which display antigens to T cells, and of costimulators, which were introduced in Chapter 2 as molecules required for full T cell responses.

The net result of this sequence of events is that the protein antigens of microbes that enter the body are transported to and concentrated in the regions of the lymph nodes (and spleen) where the antigens are most likely to encounter T lymphocytes. Recall that naive T lymphocytes continuously recirculate through lymph nodes and also express CCR7, which promotes their entry into the T cell zones of lymph nodes (see Chapter 1 ). Therefore, dendritic cells bearing captured antigen and naive T cells poised to recognize antigens come together in lymph nodes. This process is remarkably efficient; it is estimated that if a microbial antigen is introduced at any site in the body, a T cell response to the antigen begins in the lymph nodes draining that site within 12 to 18 hours.
Different types of APC serve distinct functions in T cell–dependent immune responses ( Fig. 3.6 ).
- •
Dendritic cells are the principal inducers of T-dependent responses, because these cells are located at sites of microbe entry and are the most potent APCs for activating naive T lymphocytes.
- •
One important type of APC for effector T cells, especially of the helper T cell lineage, is the macrophage, which is abundant in all tissues. In cell-mediated immune reactions, macrophages phagocytose microbes and display the antigens of these microbes to effector T cells, which then are reactivated and induce the macrophages to kill these ingested microbes (see Chapter 6 ).
- •
B lymphocytes endocytose protein antigens and display them to helper T cells within lymphoid tissues; this process is important for the development of humoral immune responses to protein antigens (see Chapter 7 ).
- •
As discussed later in this chapter, any nucleated cell containing foreign (microbial or tumor) protein antigens in the cytosol can present peptides derived from these antigens to CD8 + T cells.

Now that we know how protein antigens are captured, transported to, and concentrated in peripheral lymphoid organs, we next ask, how are these antigens displayed to T lymphocytes? To answer this question, we first need to appreciate the structure of MHC molecules and examine how they function in immune responses.
Structure and Function of Major Histocompatibility Complex Molecules
MHC molecules are membrane proteins on APCs that display peptide antigens for recognition by T lymphocytes. The MHC was discovered as the genetic locus that is the principal determinant of acceptance or rejection of tissue grafts exchanged between individuals (tissue, or histo, compatibility). In other words, individuals who are identical at their MHC locus (inbred animals and identical twins) will accept grafts from one another, and individuals who differ at their MHC loci will reject such grafts. Because graft rejection is not a natural biologic phenomenon, MHC genes and the molecules they encode must have evolved to perform other functions. We now know that the physiologic role of MHC molecules is to display peptides derived from microbial protein antigens to antigen-specific T lymphocytes as a first step in protective T cell–mediated immune responses to microbes. This function of MHC molecules explains the phenomenon of MHC restriction of T cells, mentioned earlier.
All vertebrates possess maternally and paternally inherited MHC loci, which include genes encoding the MHC proteins (and other proteins involved in immune responses) ( Fig. 3.7 ). MHC molecules were first discovered as proteins encoded by the murine MHC locus involved in graft rejection. They were rediscovered in humans when it was found that women who had multiple pregnancies, or recipients of multiple blood transfusions, made antibodies that recognized proteins on the white blood cells (leukocytes) of paternal or donor origin, respectively. These proteins were called human leukocyte antigens (HLAs) and were soon shown to be analogous to the MHC molecules identified in mice. (Pregnancy and transfusions expose individuals to cellular antigens of other individuals, so antibodies produced against these cells reflect histoincompatibility, as in the mouse grafting experiments.) In all vertebrates, the MHC contains two sets of highly polymorphic genes, called the class I and class II MHC genes. (As discussed later, polymorphism refers to the presence of many variants of these genes in the population.) These genes encode the class I and class II MHC molecules that display peptides to T cells. In addition to the polymorphic genes, the MHC contains many nonpolymorphic genes, some of which code for proteins involved in antigen presentation.

Structure of MHC Molecules
Class I and class II MHC molecules are membrane proteins that each contains an extracellular peptide-binding cleft. Although the two classes of molecules differ in subunit composition, they are very similar in overall structure ( Fig. 3.8 ).

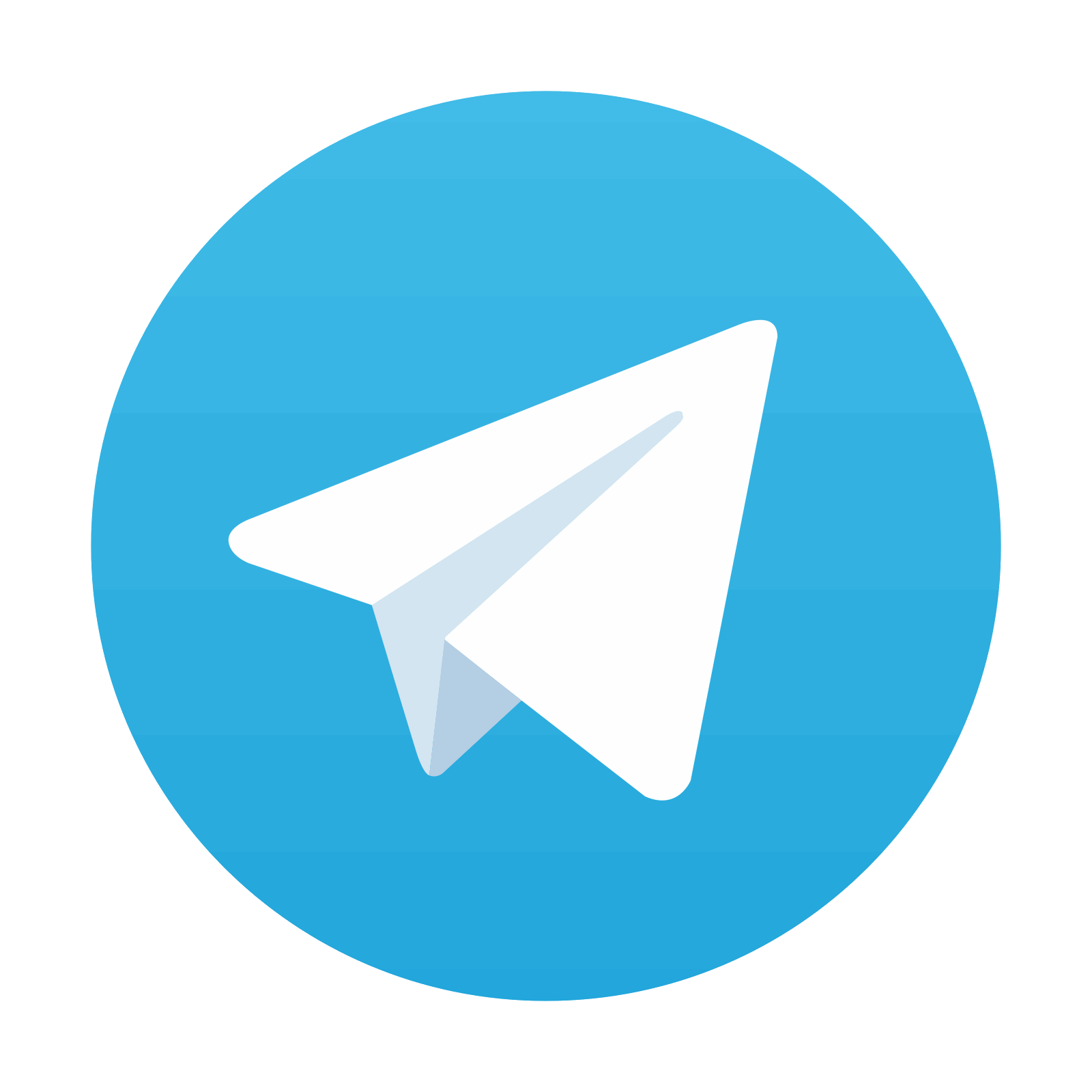
Stay updated, free articles. Join our Telegram channel

Full access? Get Clinical Tree
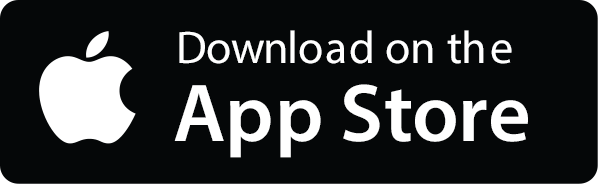
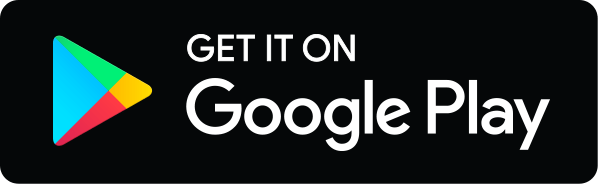
