Chapter 26 Central and peripheral nervous systems
COMMON CLINICAL PROBLEMS FROM CENTRAL AND PERIPHERAL NERVOUS SYSTEM DISEASE
Pathological basis of neurological signs and symptoms
Sign or symptom | Pathological basis |
---|---|
Headache | |
Neck stiffness | |
Coma or impaired consciousness | Metabolic, e.g.: Brainstem lesions, e.g.: Cerebral hemisphere lesions, e.g.: |
Dementia | Loss of limbic or cortical neurones due to ischaemia, toxic injury or neurodegenerative disease, e.g. Alzheimer’s disease |
Epileptic fits | Paroxysmal neuronal discharges, either idiopathic or emanating from a focus of cortical disease or damage |
Abnormal reflexes | |
Muscle deficit | |
Disease directly or indirectly affecting function of: | |
Sensory impairment and/or paraesthesiae | Disease directly or indirectly affecting function of: |
Visual field defects or blindness | Disease involving the eyes, optic nerves and pathway or visual cortex (e.g. cataracts, tumours (intrinsic or extrinsic to optic neural pathway), inflammation or demyelination in the optic pathway, retinopathy, ischaemia) |
Tinnitus and/or deafness | Impaired transmission of sound through external meatus (e.g. wax) or through middle ear ossicles, or disease affecting the organ of Corti or the auditory nerve |
CENTRAL NERVOUS SYSTEM
NORMAL STRUCTURE AND FUNCTION
The CNS is composed of three principal structures: the brain, brainstem and spinal cord. The brain comprises two hemispheres which are joined by a band of white matter fibres known as the corpus callosum. The grey matter known as the cerebral cortex is located on the outer surface of the hemispheres, and is composed of six layers of neurones. The cerebral cortex is divided into four anatomical regions: the frontal, temporal, parietal and occipital lobes. Each of these has distinct functions, which are summarised in Figure 26.1. The white matter beneath the cerebral cortex is composed of axons which connect the cortical neurones with neurones in other grey matter regions, including the opposite hemisphere. In the centre of the hemispheres there is a complex series of grey matter nuclei known as the basal ganglia, the thalamus and the hypothalamus. Their principal functions are summarised in Table 26.1. The cerebellum is located at the posterior surface of the brainstem, to which it is connected by white matter fibre bundles. The cortex of the cerebellum lies on its outer surface, but its structure is different from that of the cerebral cortex. The function of the cerebellum is summarised in Table 26.1.
Table 26.1 Functions of the basal ganglia, thalamus, hypothalamus and cerebellum
Structure | Functions |
---|---|
Basal ganglia | |
Thalamus | |
Hypothalamus | |
Cerebellum |
The brainstem contains many ascending and descending white matter fibre bundles which connect the spinal cord to the brain; however, it also contains many nuclei, including cranial nerves 3–12, the substantia nigra, the respiratory centre and the vomiting centre. The spinal cord is largely composed of ascending and descending white matter fibre bundles, such as the corticospinal pathways (descending motor fibres) and the posterior columns (ascending sensory fibres). The grey matter of the spinal cord is located in the centre, and contains several groups of neurones, including the anterior horn cells, which are the lower motor neurones supplying all the skeletal muscle in the trunk and limbs. Motor nerve roots leave the anterior spinal cord to form peripheral motor nerves; sensory nerves from the skin, joints and organs enter the spinal cord by the posterior nerve roots, and then pass into the ascending posterior columns.
Neurones
Neurones are the structural and functional units of the CNS, generating electrical impulses that allow rapid cell–cell communication at specialised junctions known as synapses (Fig. 26.2). Many millions of neurones are present, arranged in layers within the cortex on the surface of the cerebellum and the cerebral hemispheres. Groups of functionally related neurones within the subcortical grey matter are known as nuclei (Table 26.1). Neurones are highly specialised post-mitotic cells which cannot be replaced after cell death. They are subject to unique metabolic demands, having to maintain an axon (which may be up to 1m in length) by intracellular transport. This makes neurones particularly vulnerable to a wide range of insults, principally hypoxia and hypoglycaemia.
Neurones contain ion channels within the cell membrane that can be opened by either changing the voltage across the membrane or by the binding of a chemical (neurotransmitter) to a receptor in or near the ion channel. In the resting state, the neuronal cell membrane is relatively impermeable to ions. Opening of the ion channels allows an influx of sodium ions which depolarises the membrane, forming an action potential which is transmitted rapidly down the axon by saltatory conduction. Cell to cell transmission occurs at the synapse (Fig. 26.2). The commonest excitatory neurotransmitter in the CNS is glutamate. Excessive release of glutamate under certain conditions such as cerebral ischaemia and epilepsy can result in excitotoxic neuronal cell death.
The cell body or perikaryon is easily seen by light microscopy (Fig. 26.3). It contains neurofilaments, microtubules, lysosomes, mitochondria, complex stacks of rough endoplasmic reticulum, free ribosomes and a single nucleus with a prominent nucleolus. Some groups of neurones contain the pigment neuromelanin and are readily identifiable with the naked eye as darkly coloured nuclei, e.g. in the substantia nigra.
Glia
Glia are specialised supporting cells of the CNS comprising four main groups:
Astrocytes are process-bearing cells which are poorly visualised by light microscopy (Fig. 26.3) unless special staining techniques are used. They perform several important roles:
Oligodendrocytes are the most numerous cells in the CNS. On light microscopy, they are visible as darkly staining nuclei located around neurones and nerve fibres (Fig. 26.3). The most important function of oligodendrocytes is the synthesis and maintenance of myelin in the CNS.
Connective tissue
The meninges comprise the pia, arachnoid and dura mater, and the arachnoidal granulations which are the main sites of CSF absorption. The meninges are composed of fibroblast-like cells which also extend around meningeal and cerebral blood vessels.
REACTIONS OF CNS CELLS TO INJURY


Neurones
Neurones can undergo various reactive changes to cell injury:
Central chromatolysis is a distinctive reaction which usually occurs in response to axonal damage (Fig. 26.4). This reaction is maximal at around 8 days following axonal damage, and is accompanied by increased RNA and protein synthesis, suggestive of a regenerative response.
Anterograde degeneration occurs as a result of axonal transection, and is usually accompanied by central chromatolysis (Fig. 26.4). Degeneration of the distal part of the axon will occur following its separation from the intact perikaryon, e.g. by transection. Within 4 days, the distal segment degenerates and becomes fragmented. The myelin sheath surrounding the axon also fragments, but this usually occurs only after axonal degeneration is established. Axonal and myelin debris is then phagocytosed by macrophages, which often remain around the site of injury for several months. Attempts at axonal regeneration do not occur to a significant extent in the CNS.
DYSFUNCTION OF THE CNS
Diseases of the CNS impair the highly complex integration that is necessary for normal neurological function. The resulting clinical abnormalities can often indicate the anatomical basis of the lesion in the CNS, and this can be investigated in greater detail by imaging of the CNS by MRI scanning. The pathological basis box (p. 749) gives an introduction to some common clinical abnormalities and their pathological basis in the CNS.
INTRACRANIAL SPACE-OCCUPYING LESIONS


Consequences of intracranial space-occupying lesions
The consequences of intracranial space-occupying lesions may be:
Raised intracranial pressure
Intracranial shift and herniation
Lateral shift of the midline structures is a common early complication of intracranial space-occupying lesions. However, patients with acute lateral displacement of the brain due to a hemispheric mass show a depressed level of consciousness even in the absence of an intracranial herniation. The clinical features are summarised in Table 26.2.
Table 26.2 Clinical consequences of intracranial herniation
Site of herniation | Effect | Clinical consequence |
---|---|---|
Transtentorial | ||
Foramen magnum | Brainstem compression and haemorrhage | |
Acute obstruction of CSF pathway |
Herniations occur at several characteristic sites within the cranial cavity, depending on the site of the space-occupying lesion (Fig. 26.5). Transtentorial herniation is frequently fatal because of secondary haemorrhage into the brainstem (Fig. 26.6). This is a common mode of death in patients with large intrinsic neoplasms or intracranial haemorrhage.
CNS TRAUMA


Missile injury to the brain
Non-missile injury to the brain
Primary brain damage
Focal damage
The commonest type of focal damage is contusions. These often occur at the site of impact, particularly if a skull fracture is present. Contusions are commonly asymmetrical and may be more severe on the side opposite the impact—the ‘contrecoup’ lesions (Fig. 26.7). Movement of the brain within the skull brings these areas into contact with adjacent bone, resulting in local injury. Large contusions may be associated with an intracerebral haemorrhage, or accompanied by cortical lacerations. Healed contusions are represented by wedge-shaped areas of gliosis and cortical rarefaction which are yellow–brown due to the presence of haemosiderin.
Diffuse axonal injury
Secondary brain damage
Table 26.3 Mechanisms and clinical manifestations of traumatic intracranial haemorrhage
Site | Mechanism | Clinical manifestations |
---|---|---|
Extradural space | Skull fracture with arterial rupture, e.g. middle meningeal artery | Lucid interval followed by a rapid increase in intracranial pressure |
Subdural space | Rupture of venous sinuses or small bridging veins due to torsion forces | |
Subarachnoid space | Arterial rupture | Meningeal irritation with a rapid increase in intracranial pressure |
Cerebral hemisphere |
Spinal cord injuries
Open injuries
Open injuries cause direct trauma to the spinal cord and nerve roots. Perforating injuries can cause extensive disruption and haemorrhage, but penetrating injuries may result in incomplete cord transection which can be manifested clinically as the Brown–Séquard syndrome (hemisection of the cord resulting in an upper motor neurone lesion and loss of position and vibration sense on the affected side with loss of pain and temperature sense on the contralateral side, below the level of the injury—see Fig. 26.20).
SPINAL CORD AND NERVE ROOT COMPRESSION
The principal causes of spinal cord and nerve root compression are:
Intervertebral disc prolapse
Intervertebral disc prolapse (Ch. 25) occurs in two main ways:
Spondylosis
Spondylosis due to osteoarthritis (Ch. 25) of the vertebral column occurs commonly with age. It affects around 70% of adults over 40 years of age, and is usually accompanied by degenerative disc disease. It is characterised by bony outgrowths, known as osteophytes, on the upper and lower margins of the vertebral bodies. These may encroach upon the spinal canal or intervertebral foramina to produce nerve root pain which is exacerbated by movement.
HYDROCEPHALUS


The cerebrospinal fluid (CSF) is secreted by the choroid plexus epithelium in an active process which carefully regulates its biochemical composition. In adults, the total volume of CSF is around 140ml; this volume is renewed several times daily (Fig. 26.8).
Primary hydrocephalus
Obstructive hydrocephalus
Obstructive hydrocephalus is by far the commonest form; it may be either congenital or acquired.
Congenital hydrocephalus
Congenital hydrocephalus occurs in around 1 per 1000 births and occasionally may be so marked as to enlarge the fetal head considerably and interfere with labour. The more severe forms may be diagnosed antenatally by ultrasonography. Congenital malformations, for example Arnold–Chiari malformation (see Fig. 26.22), are the principal causes of congenital hydrocephalus. A few cases in males are due to an X-linked disorder that results in aqueduct stenosis. Aqueduct stenosis is more commonly due to acquired disorders, for example viral infections, which affect both sexes.
Acquired hydrocephalus
Acquired hydrocephalus can result from any lesion that obstructs the CSF pathway (Fig. 26.8). Expanding lesions in the posterior fossa are particularly prone to cause hydrocephalus, as the fourth ventricle and aqueduct are easily obstructed. Some lesions may cause intermittent obstruction, particularly colloid cysts of the third ventricle which may block the foramen of Monro. Obstructive hydrocephalus commonly results from the organisation of blood clot or inflammatory exudate in the CSF pathway following an episode of haemorrhage or meningitis (Fig. 26.9). Intermittent pressure hydrocephalus is thought to result from defective CSF absorption at the arachnoid villi.
Secondary hydrocephalus
In secondary or compensatory hydrocephalus the increase in CSF volume occurs following a loss of brain tissue, for example cerebral infarction or atrophy, so that overall there is no increase in either intracranial volume or intracranial pressure (see Fig. 26.26).
Complications and treatment
The complications of hydrocephalus can be averted or relieved by the insertion of a ventricular shunt with a one-way valve system to drain CSF into the peritoneum. Untreated patients may suffer irreversible brain damage (Fig. 26.9). Ventricular shunts often need to be replaced in growing children and are prone to become infected with low-virulence bacteria, for example Staphylococcus epidermidis. Infection may result in shunt blockage and exacerbation of symptoms attributable to raised intracranial pressure.
SYRINGOMYELIA
Syringomyelia is an uncommon condition in which a cavity (syrinx) develops within the spinal cord, sometimes extending up into the brainstem (syringobulbia). The cavity is usually situated in the central region of the cord, posterior to the central canal. Syringomyelia occurs most frequently in the cervical region of the cord, and usually extends for several centimetres in a vertical direction. However, extensive cavities involving almost the entire length of the cord have been described. Modern radiological techniques are of great value in delineating the extent of the lesion (see Fig. 26.22).
Syringomyelia can arise in a variety of conditions, which may be considered as follows:
The clinical manifestations of syringomyelia usually occur in adult life, with:
CEREBROVASCULAR DISEASE

Hypoxic damage to the CNS
Stroke
The clinical features of stroke result from focal cerebral ischaemia, and depend on the localisation and nature of the lesion (Table 26.4). Recurrent or multiple strokes often occur in patients with certain risk factors, particularly heart disease, hypertension and diabetes mellitus.
Cerebral infarction
The site and size of a cerebral infarct depend on the site and nature of the vascular lesion. Most infarcts occur within the cerebral hemispheres in the internal carotid territory, particularly in the distribution of the middle cerebral artery. Infarction of the corticospinal pathway in the region of the internal capsule is a common event, resulting in contralateral hemiparesis. Although many infarcts produce clinical symptoms, small infarcts may not result in any apparent neurological disturbance. These micro-infarcts are often found in apparently normal elderly individuals, but are also numerous in the brains of hypertensive patients. Multiple infarcts involving the cerebral cortex may result in dementia (p. 779).
Pathogenesis
The following mechanisms may be responsible for cerebral infarction:
Morphological features
At a very early stage after cerebral infarction, no naked-eye abnormalities are apparent. However, 24 hours after infarction the affected tissue becomes softened and swollen, with a loss of definition between grey and white matter. There may be considerable oedema around the infarct, resulting in a local mass effect. Within 4 days, the infarcted tissue undergoes colliquative necrosis. Histology shows infiltration by macrophages, which are filled with the lipid products of myelin breakdown. Reactive astrocytes and proliferating capillaries are often present at the edge of the infarct. Eventually, all the dead tissue is phagocytosed to leave a fluid-filled cystic cavity with a gliotic wall (Fig. 26.10). Some infarcts are haemorrhagic, possibly due to reflow of blood through anastomotic channels. Anterograde degeneration of nerve fibres occurs distal to the site of infarction, for example in the ipsilateral cerebral peduncle in infarcts involving the internal capsule.
Intracranial haemorrhage
Intracerebral and subarachnoid haemorrhage together account for around 18% of strokes. Extradural and subdural haemorrhages usually occur following trauma and are considered in Table 26.3.
Intracerebral haemorrhage
The commonest cause of intracerebral haemorrhage is hypertensive vascular disease, in which haemorrhages occur most frequently in the basal ganglia (80% of cases), the brainstem, cerebellum and cerebral cortex. Most intracerebral haemorrhages occur in hypertensive adults over 50 years of age. The haematoma acts as a space-occupying lesion, causing a rapid increase in intracranial pressure and intracranial herniation (Fig. 26.11). In survivors, resorption of the haematoma eventually occurs, and a fluid-filled cyst with a gliotic wall is formed. The mortality from spontaneous intracerebral haemorrhage is greater than 80%, and many survivors suffer severe neurological deficit.
The pathogenesis of spontaneous intracerebral haemorrhage is not fully understood. For many years, it was thought that most intracerebral haemorrhages in hypertensive patients occurred following rupture of micro-aneurysms on small arterioles, particularly on the lenticulostriate branch of the middle cerebral artery. Recent studies, however, have found that the ruptured vessels are arterioles, which show replacement of smooth muscle by lipids and fibrous tissue (lipohyalimosis), predisposing to rupture. Intracerebral haemorrhage in children and younger adults may occur as a consequence of trauma, or rupture of an arteriovenous malformation. In older adults, haemorrhage into the lobes of the brain may be due to amyloid depostion in the vessel walls (amyloid angiopathy), which is associated with Alzheimer’s disease (p. 779).
Subarachnoid haemorrhage
Saccular aneurysms
Saccular aneurysms are usually sited at proximal branching points on the anterior portion of the circle of Willis, particularly on the internal carotid, anterior communicating and middle cerebral arteries. Most are less than 10mm in diameter, but some may be partly filled by thrombus, which can obscure their true size on radiological studies (Fig. 26.12). Their pathogenesis is thought to relate to congenital defects in the smooth muscle of the tunica media at the site of an arterial bifurcation, where local haemodynamic factors act to produce a slowly enlarging aneurysm.
Systemic hypertension and the CNS
Intracranial haemorrhage in neonates
Intracranial haemorrhage in neonates has a markedly different pathology from intracranial haemorrhage in adults (Table 26.5). Haemorrhage from the subependymal germinal matrix is particularly important, and is the major cause of death in premature neonates.
Table 26.5 Intracranial haemorrhage in neonates
Site | Pathogenesis | Complication |
---|---|---|
Rupture of veins (birth trauma) | ||
Subdural space | ||
Subarachnoid space | Capillary or arterial rupture | Hydrocephalus |
Subependymal germinal matrix | Prematurity, hypoxia (hyaline membrane disease) | Intraventricular haemorrhage |
Venous congestion | Venous infarction in white matter (periventricular leukomalacia) | |
Arterial spasm | Hydrocephalus |
CNS INFECTIONS
Bacterial infections



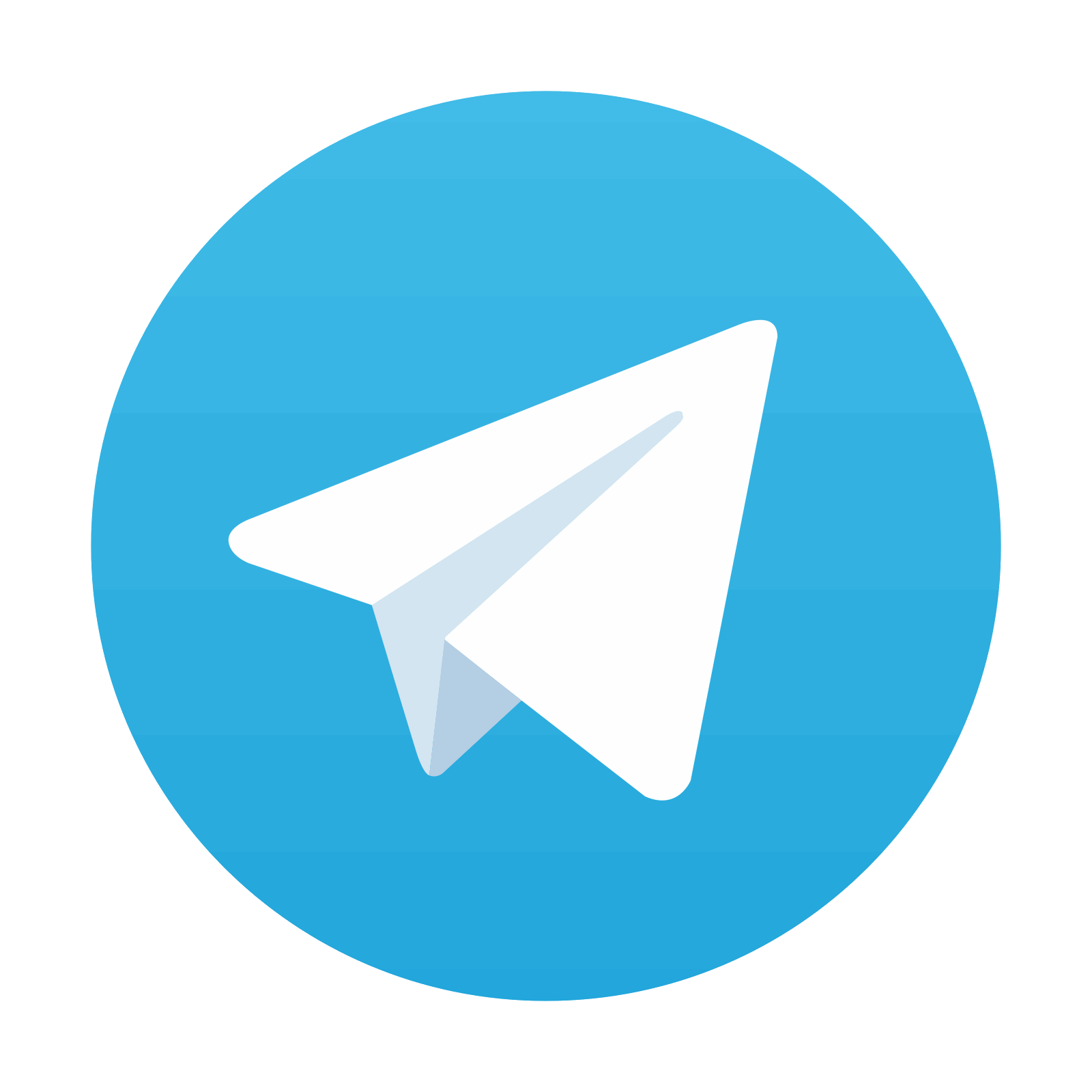
Stay updated, free articles. Join our Telegram channel

Full access? Get Clinical Tree
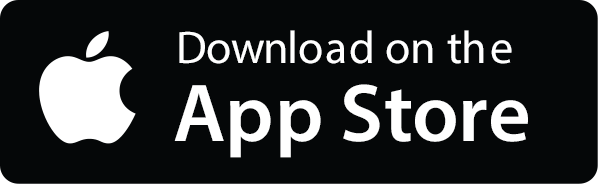
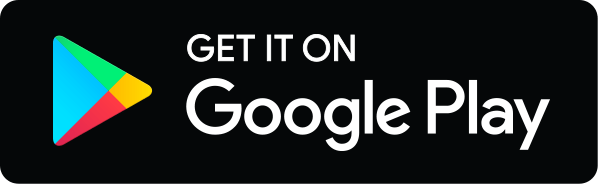