Chapter 9 Immunology and immunopathology
DEFENCE AGAINST INFECTION

Non-specific defences
Many non-specific mechanisms prevent invasion of the body by micro-organisms:
Innate immunity
KEY MOLECULES






Antigens
Antigens are substances able to provoke an immune response and react with the immune products. They react both with the T-cell recognition receptor and with antibody. An antigenic molecule may have several antigenic determinants (epitopes); each epitope can bind with an individual antibody, and a single antigenic molecule can therefore provoke many antibody molecules with different binding sites. Some low molecular weight molecules, called haptens, are unable to provoke an immune response themselves, although they can react with existing antibodies. Such substances need to be coupled to a carrier molecule in order to have sufficient epitopes to be antigenic. For some chemicals, such as drugs, the carrier may be a host protein—called an auto-antigen. The tertiary structure, as well as the amino acid sequence, is important in determining antigenicity.
Antibody
Humoral immunity is dependent on the production of antibodies and their actions. All antibodies belong to the immunoglobulin class of proteins and are produced by plasma cells, themselves derived from B-lymphocytes. The basic structure of an immunoglobulin molecule is shown in Figure 9.2. It has a four-chain structure: two identical heavy (H) chains (molecular weight 50 kD) and two identical light (L) chains (mol wt 25 kD). There are two alternative types of light chain, known as kappa and lambda; an antibody molecule has either two kappa or two lambda light chains, never one of each. In contrast, there are five types of heavy chain, each with important functional differences (Table 9.1). The heavy chains determine the class (isotype) of the antibody and the physiological function of the antibody molecule. Once the antigen-binding site has reacted with its antigen, the molecule undergoes a change in the conformation of its heavy chains in order to take part in effector functions (Table 9.1).
The amino (N) terminal regions of the heavy and light chains include the antigen-binding sites. The amino acid sequences of these N- terminal domains vary between different antibody molecules of the same isotype and are known as variable (V) regions. Most of these differences reside in three hypervariable areas of the molecule, each only 6–10 amino acid residues long. In the folded molecules, these hypervariable regions in each chain come together, with their counterparts on the other pair of heavy and light chains, to form the antigen-binding site. The structure of this part of the antibody molecule is unique to that molecule and is known as the idiotypic determinant. In any individual, about 106–107 different antibody molecules could be made up by 103 different heavy chain variable regions associating with 103 different light chain variable regions. Somatic mutation during multiple divisions of B-lymphocytes generates further diversity of around 1014 antibody specificities.
IgM is the oldest class of immunoglobulin in evolutionary terms. It is a large molecule consisting of five basic units held together by a joining (J) chain; it penetrates poorly into tissues on account of its large size (Table 9.1). The major physiological role of IgM is intravascular neutralisation of organisms (especially viruses) aided by its 10 antigen-binding sites. IgM also has multiple complement-binding sites; this results in excellent complement activation and lysis of the organism or removal of the antigen–antibody–complement complexes by complement receptors on phagocytic cells. It is the first class of antibody to be formed in response to an initial encounter with an antigen (primary immune response).
There is little free IgD or IgE in serum or normal body fluids. These two classes mainly act as cell receptors. IgD is synthesised by antigen-sensitive B-lymphocytes and acts as a cell surface receptor for antigen. IgE is produced by plasma cells but taken up by specific IgE receptors on mast cells and basophils. IgE probably evolved as a way of expelling intestinal parasites via mast cell degranulation.
T-cell receptors
Like B-cells, each T-cell is committed to a given antigen, which it recognises by one of two types of T-cell receptor (TCR). T-cells have either alpha/beta TCR (a heterodimer of alpha and beta chains) or gamma/delta TCR (a heterodimer of gamma and delta chains). Alpha/beta TCRs predominate in adults, although 10% of T-cells in epithelial structures are of the gamma/delta TCR type. Each type of TCR is associated with several transmembrane proteins which make up the cluster differentiation 3 (CD3) molecule (Fig. 9.3) to form the CD3–TCR complex responsible for taking the antigen recognition signal inside the cell (transduction). The CD3 antigen is widely used as a marker of mature T-cells in diagnostic and investigative pathology.
Major histocompatibility complex antigens
Histocompatibility antigens were so named because of the vigorous reactions they provoked during mismatched organ transplantation. However, these antigens play a fundamental role in the normal immune response by presenting antigenic peptides to T-cells. Human major histocompatibility complex (MHC) antigens are also known as human leukocyte antigens(HLAs). MHC antigens are cell surface glycoproteins of two basic types: class I and class II (Fig. 9.4). They exhibit extensive genetic polymorphism with multiple alleles at each locus. As a result, genetic variability between individuals is very great and most unrelated individuals possess different HLA molecules. This means that it is very difficult to obtain perfect HLA matches between unrelated persons for transplantation.
MHC class I antigens are subdivided into three groups: A, B and C. Each group is controlled by a different gene locus within the major histocompatibility complex on chromosome 6 (Fig. 9.4). The products of the genes at all three loci are chemically similar. MHC class I antigens (Fig. 9.5) are made up of a heavy chain (alpha) controlled by a gene in the relevant MHC locus, associated with a smaller chain called beta-2-microglobulin, controlled by a gene on chromosome 15. The differences between individual MHC class I antigens are due to variations in the alpha chains; the beta-2-microglobulin component is constant. The detailed structure of class I antigens was determined by X-ray crystallography. This shows that small antigenic peptides are tightly bound to a groove in the surface alpha chains.
MHC class II antigens have a folded structure similar to class I antigens with the peptide-binding groove found between the alpha and beta chains (Fig. 9.5). Whereas class I molecules are expressed by most nucleated cells, expression of class II molecules is restricted to dendritic cells, B-lymphocytes, activated T-cells, macrophages, inflamed vascular endothelium and some epithelial cells. However, other cells (e.g. thyroid, pancreas, gut epithelium) can be induced to express class II molecules under the influence of interferon-gamma released during inflammation. In humans, there are three groups of class II antigen: the loci are known as HLA-DP, HLA-DQ and HLA-DR.
MHC class III antigens (Fig. 9.4) constitute early complement proteins C4 and C2. Other inflammatory proteins, e.g. tumour necrosis factor (TNF), are encoded in adjacent areas.
Accessory and co-stimulatory molecules
Accessory and co-stimulatory molecules are needed for efficient binding and signalling (Fig. 9.6). Each accessory molecule has a corresponding protein, or ligand, to which it binds. The interaction between antigen-presenting cells and T-cells is strongly influenced by accessory molecules which function as co-stimulators; for example, CD80 and CD86 on the activated dendritic cell engage with their counter-receptors CD28 and CTLA-4 (CD152) on the T-cell surface (Fig. 9.6). A functional co-stimulatory pathway is essential for T-cell activation. In the absence of a co-stimulatory signal, interaction between the dendritic cell and T-cell leads to T-cell unresponsiveness (a state called anergy).
The selectin family comprises three glycoproteins designated by the prefixes E- (endothelial), L- (leukocyte) or P- (platelet) to denote the cells on which they were first described. Selectins bind strongly to carbohydrate molecules on leukocytes and endothelial cells, and regulate the homing of these cells to sites of inflammation.
Cytokines
Among the array of cytokines produced by macrophages and T-cells, interleukin-1 (IL-1) and interleukin-2 (IL-2) have a pivotal role in amplifying immune responses. IL-1 acts on a wide range of targets, including T- and B-cells (Table 9.2). In contrast, the effects of IL-2 are restricted largely to lymphocytes: it has a trophic effect on T-cells, IL-2 receptor-bearing B-cells and natural killer (NK) cells. The considerable overlap between individual cytokines and interleukins is summarised in Table 9.3.
Table 9.2 Actions of interleukin-1
Target cell | Effect |
---|---|
T-lymphocytes | |
B-lymphocytes | |
Neutrophils | |
Proliferation/activation | |
Osteoclasts | Reabsorption of bone |
Hepatocytes | Acute-phase protein synthesis |
Hypothalamus | Prostaglandin-induced fever |
Muscle | Prostaglandin-induced proteolysis |
Table 9.3 Cytokines and their actions
Cytokine | Source | Action |
---|---|---|
IL-2 | T-cells | Proliferation and maturation of T-cells, induction of IL-2 receptors and activation of NK cells |
![]() | Induction of MHC class II, FcR and IL-2R on B- and T-cells; induction of isotype switching in B-cells; facilitate IgE production (mainly IL-4) | |
IL-6 | Macrophages, fibroblasts, T-cells, mast cells | Growth and differentiation of T-, B- and haemopoietic cells; acute phase response |
IL-8 | Monocytes/macrophages, T-cells, fibroblasts, keratinocytes, endothelial cells, NK cells | Chemotaxis of neutrophils |
IL-10 | T-cells, monocytes, keratinocytes | Inhibitory cytokine production |
IL-12 | B-cells, monocytes/macrophages | Synergism with IL-2; regulates IFN-gamma production |
IL-13 | T-cells | Actions overlap with IL-4 |
GM-CSF | T-cells, macrophages, endothelial cells | Stimulates growth of polymorphs and mononuclear progenitors |
IFN-gamma | T-cells, NK cells | Activation of macrophages, endothelial cells and NK cells; increases expression of MHC class I and II; inhibits IgE production |
TNF | Macrophages, T-cells, B-cells | Promotion of inflammation; interferes with catabolism in muscle and fat |
STRUCTURAL ORGANISATION OF THE IMMUNE SYSTEM

T- and B-lymphocyte development
All lymphoid cells originate in the bone marrow although the nature of the uncommitted lymphoid stem cell remains unclear (Fig. 9.7). An understanding of the developmental pathway is important, not only to clarify the physiology of the normal immune response but also because some leukaemias and immunodeficiency states reflect maturation arrest of cells in their early stages of development. Lymphoid progenitors destined to become T-lymphocytes migrate from the bone marrow into the cortex of the thymus where further differentiation into mature T-cells occurs. Passage of T-cells from the thymic cortex to the medulla is associated with the acquisition of characteristic surface glycoprotein molecules so that medullary thymocytes resemble mature peripheral blood T-cells. T-cell development in the thymus is characterised by a process of positive selection whereby T-cells that recognise and bind with low affinity to fragments of self-antigen in association with self-MHC molecules proceed to full maturation. In contrast, T-cells that do not recognise self-MHC or that recognise and bind with high affinity to self-antigen are selected out—negative selection—and do not develop further. Negatively selected T-cells kill themselves by apoptosis, i.e. programmed cell death. This process is an important mechanism in preventing autoimmune disease. In summary, the thymus selects out the useful, neglects the useless and destroys the harmful, i.e. autoreactive T-cells.
Primary and secondary lymphoid organs
Peripheral T- and B-cells circulate in a characteristic pattern through the secondary lymphoid organs. Most of the recirculating cells are T-cells and the complete cycle takes about 24 hours; some B-cells, including long-lived memory B-cells, also recirculate. Lymphocyte circulation is strongly influenced by chemokine receptors on lymphocyte surfaces which act as homing agents. Adhesion molecules direct cells to their respective ligands on high endothelial venules of lymph nodes.
Lymph node architecture is well adapted to its function (Fig. 9.8). Lymphatic vessels draining the tissues penetrate the lymph node capsule and drain into the marginal sinus from which a branching network of sinuses passes through the cortex to the medulla and into the efferent lymphatic. This network provides a filtration system for antigens entering the node from peripheral tissue.

Fig. 9.8 Structure of a normal lymph node. The locations of T- and B-lymphocytes are shown in Figs 9.10–9.12.
The cortex contains primary follicles of B-lymphocytes, surrounded by T-cells in the ‘paracortex’ (Figs 9.9–9.11). There is a meshwork of dendritic cells that express MHC class II antigen throughout the lymph node, and these cells filter and present antigen to lymphoid cells. On antigen challenge, the ‘primary’ follicles of the lymph node develop into ‘secondary’ follicles which contain germinal centres. These comprise mainly B-cells with a few helper T-cells and a mantle zone of the original primary follicle B-cells. B-cells in a secondary follicle are antigen-activated and more mature; most have IgG on their surfaces, whereas those in the primary follicle and mantle zone bear both IgD and IgM. Activated B-cells migrate from the follicle to the medulla, where they develop into plasma cells (Fig. 9.12) in the medullary cords before releasing antibody into the efferent lymph.

Fig. 9.11 Immunohistochemical identification of T-cells. This section, adjacent to that in Fig. 9.10, is stained with a monoclonal antibody that reacts with T-cells (anti-CD3). Cells of the paracortex are stained (arrow) and there are few T-cells in the follicle.
FUNCTIONAL ORGANISATION OF THE IMMUNE RESPONSE



Antigen presentation
The first stage of an immune response to any antigen is the processing and presentation of that antigen to lymphocytes by specialised antigen-presenting cells (APCs). T-cells cannot recognise antigen without it. The interaction between APCs and T-cells is strongly influenced by a group of cell-surface molecules that act as co-stimulators. Thus, CD80 and CD86 on the APC engage with their counter-receptors CD28 and CTLA-4 (cytotoxic T-lymphocyte antigen 4; CD152) on the T-cell surface (Fig. 9.6). Normal functioning of theco-stimulatory pathway is vital for T-cell activation. In the absence of a co-stimulatory signal, interaction between the APC and T-cell leads to T-cell unresponsiveness, or anergy. Antagonists to co-stimulatory molecules disrupt immune responses, an observation of potential therapeutic importance; for instance, antagonists to CTLA-4 are being used experimentally to treat severe autoimmune diseases and to prevent graft rejection.
Antibody production
Antibodies are synthesised by B-cells and their mature progeny, called plasma cells (Fig. 9.12). B-cells are readily recognised because they express immunoglobulin on their surfaces. During development, B-cells first show intra-cellular mu chains and then surface IgM. These cells are able to switch from production of IgM to IgG, IgA or IgE as they mature, a process known as isotype switching (Fig. 9.13). This maturation sequence fits with the kinetics of an antibody response: the primary response is mainly IgM and the secondary response predominantly IgG (Fig. 9.14).
Isotype switching is mediated by the interaction of two important proteins: CD40 expressed on the B-cell surface engages with its ligand, CD40L (CD154), on activated T-cells (under the influence of IL-4) to induce B-cells to switch immunoglobulin production from IgM to IgG, IgA or IgE (Fig. 9.13). Deficiency of either CD40 or CD40L in humans leads to a severe immunodeficiency characterised by inability to switch from IgM to IgG antibody production.
Each B-cell is committed to the production of an antibody that has a unique Vh–Vl combination, the idiotype, and the surface immunoglobulin and secreted immunoglobulin are identical. Contact with antigen and factors released by helper T-cells (IL-4, -5, -6) stimulate the B-cell to divide and differentiate, generating more antibody-producing cells, all of which make the same antibody with the same idiotype. Simultaneously, a population of memory cells is produced which express the same surface immunoglobulin receptor. The result of these cell divisions is that a greater number of antigen-specific B-cells becomes available when the animal is exposed to the same antigen at a later date. This process, known as clonal expansion, helps to account for the amplified secondary response. As well as being quicker and more vigorous (Fig. 9.14), secondary responses are more efficient because the antibodies bind more effectively to the antigen, i.e. with higher affinity.
T-cell help is antigen-specific. Only helper T-cells that have responded to antigen presented by macrophages can subsequently help B-cells already committed to that antigen. Helper T-cells recognise both antigen and MHC class II antigens as a complex on the presenting cells. They then recognise the same combination of antigen and class II molecule on the corresponding B-cell but co-stimulation is also required (Fig. 9.13). When helper T-cells meet an antigen for the first time, the limited number reacting with that antigen are activated to provide help for B-cells. They undergo blast transformation and proliferation, i.e. clonal expansion, so the immune response on second and subsequent exposures is quicker and more vigorous.
Helper T-cells are further grouped into two distinct subsets depending on their cytokine profile. Th1 cells secrete TNF and interferon-gamma and mediate cellular immunity. In contrast, Th2 cells predominantly secrete IL-4, IL-5, IL-10 and IL-13 (Fig. 9.15) and are responsible for stimulating vigorous antibody production by B-cells. T-cells expressing cytokine profiles common to both Th1 and Th2 cells are designated Th0.
NON-SPECIFIC EFFECTOR MECHANISMS


Complement
Complement activation
Complement activation occurs in two sequential phases:
The critical step (Fig. 9.16) is cleavage of C3 by complement-derived enzymes called C3 convertases. The major fragment of activated C3—called C3b—mediates a number of vital biological activities, particularly opsonisation.
The cleavage of C3 is achieved via three main routes, the classical, alternative and lectin pathways, all of which generate C3 convertases but in response to different stimuli (Fig. 9.16).
Classical pathway activation
C1 is a macromolecular complex of three subcomponents—C1q, C1r and C1s. C1q is a collagen-like protein composed of six subunits. C1q reacts with Fc regions via its globular heads but attachment by two critically spaced binding sites is needed for activation. IgM is more efficient than IgG in activating C1q. IgA, IgD and IgE do not activate the classical pathway.
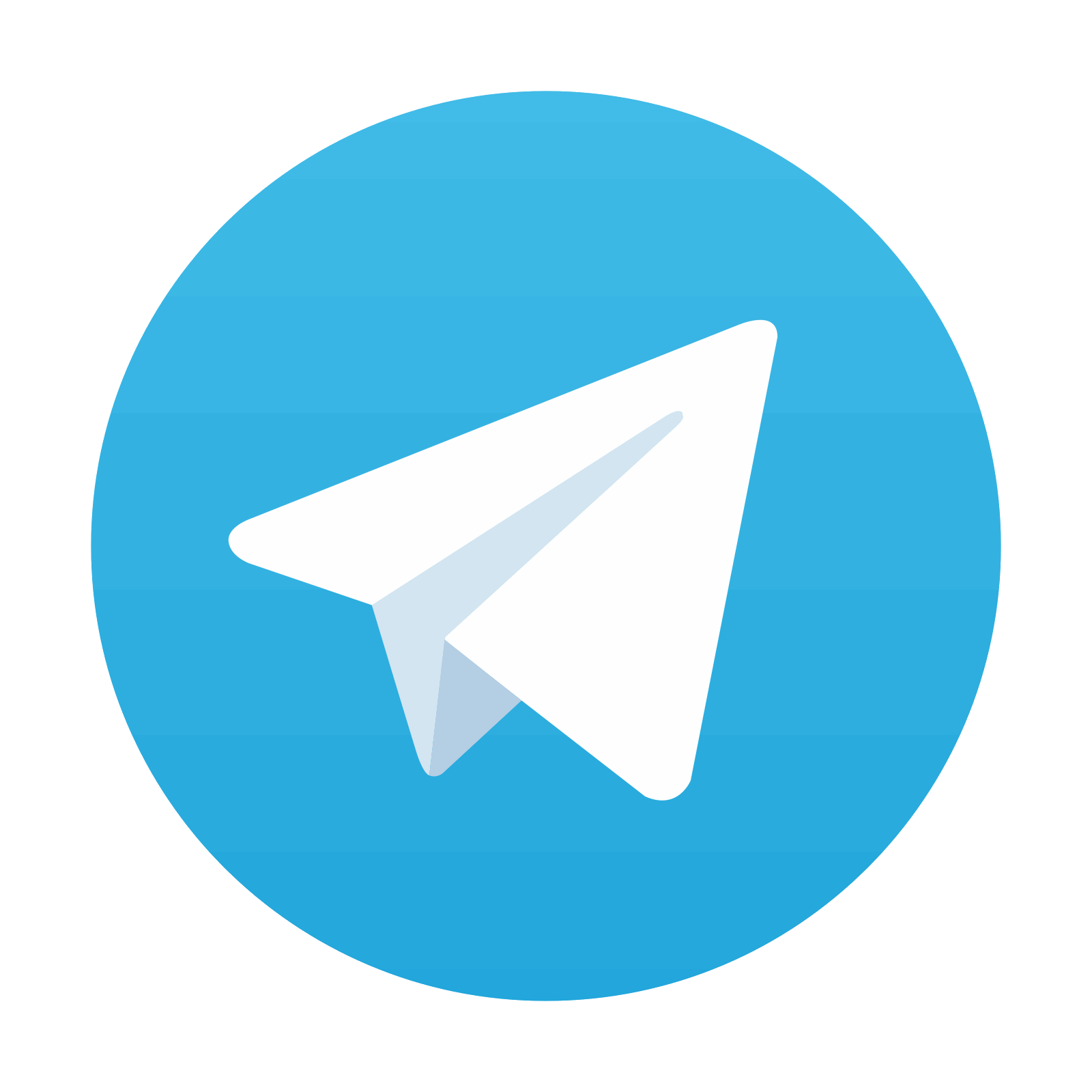
Stay updated, free articles. Join our Telegram channel

Full access? Get Clinical Tree
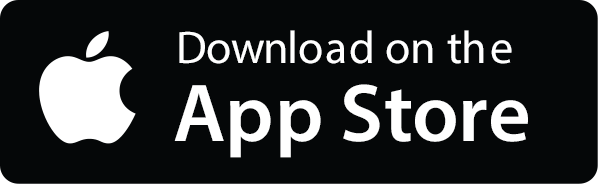
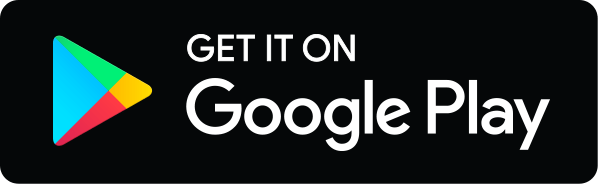