Chapter 19 Hydrocarbons and derivatives
HYDROCARBONS
Although not featuring strongly in the pharmaceutical armamentarium, hydrocarbons are important in nature as components of cuticular waxes. The majority of these are odd-numbered long-chain alkanes within the range C25–35 formed by decarboxylation of the next higher, even-numbered, free fatty acid. In recent years the long-chain polyenes of the Compositae have been systematically investigated in relation to their chemotaxonomic importance. Isoprene (C5H8), the unsaturated hydrocarbon moiety from which the terpenoids (isoprenoids) can be constructed (Fig. 18.17), has not to date been found free in nature. A number of cyclic terpenoid hydrocarbons including limonene, pinene, phellandrene and cadinene are components of essential oils. Rubber, gutta and the carotenes are polyunsaturated terpenoids.
MONOBASIC ACIDS
Organic acids possess one or more carboxyl groups and a monobasic acid may be represented as RCOOH. The very high frequency of the biochemical occurrence of the carboxyl group means that acids are found in all living organisms and as derivatives of all the major metabolic groups. They participate in essential metabolism and in this capacity range from the simple acids of the respiratory sequence to the complex deoxyribonucleic acids associated with the storage and transmission of hereditary characters. In the metabolic cycles they frequently function in association with coenzymes, and may accumulate as simple salts, esters and amides, or less frequently in the free state. Amino acids are discussed in Chapter 18.
C1–C6 Monocarboxylic acids
Some common acids are illustrated in Table 19.1.
Table 19.1 Examples of C1–C6 monocarboxylic acids.
Name | Structure | Comments |
---|---|---|
Formic acid | ![]() | Name derives from its first isolation from the ant, Formica rufa. A decomposition product of many vegetable materials. Occurs free in the hairs of the stinging nettle; combined in the gitaloxigenin series of cardioactive glycosides. N-formyl-L-methionine is involved in the initiation of protein synthesis on ribosomes |
Acetic acid | ![]() | An essential primary metabolite, particularly as acetyl-CoA. Common in the esterified form |
Propionic acid | ![]() ![]() | Produced in the fatty acid oxidative cycle when an acyl-CoA with an odd number of carbon atoms is involved. Esterified as a tropane alkaloid |
n-Butyric acid | ![]() | Occurs in traces in many fats |
n-Valeric acid | ![]() | Not common; component of Convolvulaceous resins |
iso-Valeric acid | ![]() | Free and esterified in Valeriana spp. Combined in some tropane alkaloids (e.g. valeroidine) and in the pyranocoumarin, dihydrosamidin. Intermediate in the metabolism of leucine |
2-Methylbutyric acid | ![]() | Component of some tropane and Veratrum alkaloids, Convolvulaceous glycosides and the pyranocoumarin visnadin |
Caproic acid | ![]() | Occurs in traces in many fats |
Crotonic acid (trans– butenoic acid) | ![]() | Constituent of croton oil |
Tiglic acid | ![]() | Occurs in croton oil (glycoside) from Croton tiglium. The acid of many minor tropane alkaloids, e.g. tigloidine. Component of Convolvulaceous resins and Symphytum alkaloids. Biosynthetically derived from isoleucine |
Angelic acid | ![]() | Occurs in the rhizome of Angelica. Esterifying acid of the Schizanthus alkaloid schizanthine X and of some volatile oils, e.g. chamomile oils. Component of the Cevadilla seed alkaloid cevadine and Symphytum alkaloids |
Senecioic acid | ![]() | First isolated from a species of Senecio (Compositae). Occurs as the esterifying acid of some alkaloids of Dioscorea and Schizanthus. Component of the pyranocoumarin samidin |
Fatty acids
These acids are important as components of plant oils (acyl lipids) in which they occur as esters with the trihydric alcohol glycerol. They are also components of the resins of the Convolvulaceae and of waxes in which they are esterified with long-chain alcohols. Most are C10 to C20straight-chain monocarboxylic acids with an even number of carbon atoms. Over 200 have been isolated from natural sources but relatively few are ubiquitous in their occurrence. They may be saturated (e.g. palmitic and stearic acids) or unsaturated (e.g. oleic acid). The double bonds, with a few minor exceptions such as the seed oil of pomegranate, are cis.
Less commonly they are cyclic compounds such as hydnocarpic acid and the prostaglandins. The latter are a group of physiologically active essential fatty acids found in most body tissues and are involved in the platelet-aggregation and inflammatory processes. They promote smooth muscle contraction making them of clinical use as effective abortifacients and for inducing labour. All the active natural prostaglandins are derivatives of prostanoic acid (see Table 19.4). A rich source of prostaglandin A2 (PGA2) is the soft coral Plexaura homomalla. Although recognized in the 1930s, and their structures determined in 1962, it was not until 1988 that prostaglandins were unequivocally established as components of some higher plants (cambial zones and buds of Larix and Populus spp.)
Table 19.4 Cyclic unsaturated acids.
Common name | Structural formula |
---|---|
Hydnocarpic | ![]() |
Chaulmoogric | ![]() |
Gorlic | ![]() |
Prostanoic | ![]() |
PGA2 | ![]() |
Examples of fatty acids are listed in Tables 19.2–19.4. It will be noted that some have more than one unsaturated bond, the bonds being interspersed by methylene groups. These polyunsaturated acids have received much attention in recent years both regarding their role in dietary fats and as medicinals. All the common acids have trivial names but in order to indicate more precisely their structures without recourse to the full systematic chemical name each can be represented by a symbol. Thus α-linolenic, systematic name all-cis-Δ9,12,15-octadecatrienoic acid, has 18 carbons and three double bonds which can be represented by 18:3. The position of the double bonds is then indicated by the n-x convention where n = number of carbon atoms in the molecule and x is the number of inclusive carbon atoms from the methyl (ω) end to the first carbon of the first double bond, in this case 3, so that the symbol for α-linolenic acid is 18:3(n-3). The positions of the two remaining double bonds are deduced by the fact that they will follow on from each other being separated only by one methylene (-CH2-) group. In this area students may find the literature situation somewhat confusing because in some texts the acids may be symbolized on the basis of conventional chemical systematic numbering—for fatty acids the carboxyl carbon being C1. For α-linolenic acid this is represented as 18:3(9c.12c.15c), c indicating a cis-bond. The advantage of the first system is that it indicates any bioequivalence of the double bonds in acids of different chain-length, bearing in mind that chain elongation in vivo proceeds at the carboxyl end of the molecule by the addition of 2C units. Thus it can be seen from Table 19.3 that γ-linolenic acid and arachidonic acid both fall into the biochemical ω-6 family of unsaturated fatty acids and their respective symbols 18:3(n-6) and 20:4(n-6) reflect this whereas symbols based on chemical nomenclature for these acids viz 18:3(6c,9c,12c) and 20:4(5c,8c,11c,14c) do not. A comparison of symbols for some common unsaturated acids is shown in Table 19.5.
Table 19.2 Straight-chain saturated acids.
Common name | Systematic name | Structural formula |
---|---|---|
Caprylic | n-Octanoic | CH3(CH2)6COOH |
Capric | n-Decanoic | CH3(CH2)8COOH |
Lauric | n-Dodecanoic | CH3(CH2)10COOH |
Myristic | n-Tetradecanoic | CH3(CH2)12COOH |
Palmitic | n-Hexadecanoic | CH3(CH2)14COOH |
Stearic | n-Octadecanoic | CH3(CH2)16COOH |
Arachidic | n-Eicosanoic | CH3(CH2)18COOH |
Table 19.3 Straight-chain unsaturated acids.
Common name | Number of unsaturated bonds | Structural formula |
---|---|---|
Palmitoleic | 1 | CH3(CH2)5CH=CH(CH2)7COOH |
Oleic | 1 | CH3(CH2)7CH=CH(CH2)7COOH |
Petroselinic | 1 | CH3(CH2)10CH=CH(CH2)4COOH |
Ricinoleic | 1 | CH3(CH2)5CH(OH)CH2CH=CH–(CH2)7COOH |
Erucic | 1 | CH3(CH2)7CH=CH(CH2)11COOH |
Linolenic | 2 | CH3(CH2)4CH=CHCH2CH=CH–(CH2)7COOH |
α-Linoleic | 3 | CH3CH2CH=CHCH2CH=CHCH2–CH=CH(CH2)7COOH |
γ-Linolenic | 3 | CH3(CH2)4CH=CHCH2CH=CHCH2CH=CH(CH2)4COOH |
Arachidonic | 4 | CH3(CH2)4CH=CHCH2CH=CHCH2–CH=CHCH2CH=CH(CH2)3COOH |
Table 19.5 Comparison of symbols ascribed to unsaturated fatty acids.
Common name of acid | Symbol employing biochemical equivalence of double bonds | Symbol based on chemical nomenclature |
---|---|---|
Palmitoleic | 16:1 (n-7) | 16:1 (9c) |
Oleic | 18:1 (n-9) | 18:1 (9c) |
Petroselinic | 18:1 (n-12) | 18:1(6c) |
Ricinoleic | 18:1 (n-9) (hydroxy at n-7) | D(+)-12h-18:1(9c) (h = hydroxy) |
Erucic | 22:1 (n-9) | 22:1 (13c) |
Linoleic | 18:2 (n-6) | 18:2 (9c,12c) |
Eicosadienoic | 20:2 (n-6) | 20:2 (11c,14c) |
Biosynthesis of unsaturated fatty acids
Before the elucidation of the overall chemistry of formation of polyunsaturated fatty acids such as linoleic in the early 1960s by Bloch, knowledge concerning the biosynthesis of these compounds lagged behind that of the saturated acids. Recent progress has been much more rapid and, in general, it now appears that in aerobic organisms, monoenoic acids with the double bond in the 9,10-position arise by direct dehydrogenation of saturated acids. In higher plants, for this reaction, coenzyme A may be replaced by the acyl carrier protein (ACP), and Bloch has demonstrated that stearoyl-S-ACP is an effective enzyme substrate of the desaturase system of isolated plant leaf chloroplasts. The reduced forms of nicotinamide adenine dinucleotide (NADH) or nicotinamide adenine dinucleotide phosphate (NADPH) and molecular oxygen are cofactors.
The position of the introduced double bond in respect to the carboxyl group is governed by the enzyme; hence, chain length of the substrate acid is most important. The hydrogen elimination is specifically cis but a few unusual fatty acids such as that in the seed oil of Punica granatum with the structure 18:3 (9c,11t,13c) have trans bonds. As illustrated in Fig. 19.1, further double bonds may be similarly introduced to give linoleic and linolenic acid.
Unsaturated fatty acids can also be formed in plants by elongation of a medium-chain-length unsaturated acid. This appears to occur by the formation of an intermediate, β,γ-unsaturated acid rather than the α,β-unsaturated acid normally produced in saturated fatty acid biosynthesis; the β,γ-bond is not reduced and more C2 units are added in the usual way (Fig. 19.2).
Sterculic acid, a component of seed oils of the Malvaceae and Sterculiaceae, is a cyclopropene and is also derived from oleic acid, with methionine supplying the extra carbon atom to give, first, the cyclopropane. Ricinoleic acid is a hydroxy fatty acid found in castor oil seeds and is again biosynthesized from oleic acid (Fig. 19.3).
Some of the natural acetylenes and acetylenic fatty acids have obvious structural similarities to the more common fatty acids. The hypothesis that triple bonds are formed from double bonds by a mechanism analogous to that for the formation of double bonds and involving structurally and stereochemically specific enzymes has now received experimental support. By this means (Fig. 19.4) the range of acetylenes found in Basidiomycetes and in the Compositae, Araliaceae and Umbelliferae can be derived from linoleic acid via its acetylenic 12,13-dehydroderivative, crepenynic acid, an acid first isolated from seeds oils of Crepis spp.
Aromatic acids
Two common aromatic acids are benzoic acid and cinnamic acid (unsaturated side-chain), which are widely distributed in nature and often occur free and combined in considerable amounts in drugs such as balsams. Truxillic acid, a polymer of cinnamic acid, occurs in coca leaves. Other related acids of fairly common occurrence are those having phenolic or other groupings in addition to a carboxyl group; such are: salicyclic acid (o-hydroxybenzoic acid), protocatechuic acid (3,4-dihydroxybenzoic acid), veratric acid (3,4-dimethoxybenzoic acid), gallic acid (3,4,5-trihydroxybenzoic acid) and 3,4,5-trimethoxybenzoic acid. Similarly, derived from cinnamic acid, one finds p-coumaric acid (p-hydroxycinnamic acid), ferulic acid (hydroxymethoxycinnamic acid), caffeic acid (hydroxycinnamic acid) and 3,4,5-trimethoxycinnamic acid. Unbelliferone, which occurs in combination in asafoetida, is the lactone of dihydroxycinnamic acid.
Acids having an alcohol group are quinic acid (tetrahydroxyhexahydrobenzoic acid), which occurs in cinchona bark and in some gymnosperms; mandelic acid, C6H5CHOHCOOH, which occurs in combination in cyanogenetic glycosides such as those of bitter almonds and other species of Prunus; and shikimic acid, an important intermediate metabolite (see Fig. 18.8). Shikimic acid has itself acquired recent pharmaceutical importance as the starting material for the semi-synthesis of the antiviral drug oseltamivir (Tamiflu®) for use against bird flu infections in humans. Its principal source has been star-anise fruits (q.v.), leading to a supply shortage of the plant material. Other natural sources rich in this acid and of potential future use are needles of the Pinaceae (S. Marshall, Pharm. J., 2007, 279, 719) and the fruits (gumballs) of the American sweet gum tree Liquidamber styraciflua (q.v.). The acid is also produced commercially by the fermentation of genetically modified Escherichia coli. Tropic acid and phenyllactic acid are two aromatic hydroxy acids that occur as esters in tropane alkaloids (q.v.). For examples of the above see Fig. 19.5.
Chlorogenic or caffeotannic acid is a condensation product of caffeic acid and quinic acid. It occurs in maté, coffee, elder flowers, lime flowers, hops and nux vomica and is converted into a green compound, which serves for its detection, when an aqueous extract is treated with ammonia and exposed to air. See also ‘Pseudotannins’ (Chapter 21).
The biogenesis of the aromatic ring has been discussed in Chapter 18.
DIBASIC AND TRIBASIC ACIDS
The tribasic acids, citric, isocitric and aconitic are closely related to one another. The Krebs’ citric acid cycle, which is discussed in Chapter 18, is very important. Citric acid is abundant in fruit juices, and aconitic acid, which occurs in Aconitum spp., is anhydrocitric acid. It forms part of the Krebs’ cycle and the glyoxalate cycle in microorganisms.
Of interest are opines, a group of substances formed by a host plant after infection with Agrobacterium spp.; a number of these compounds are di- and tri-carboxylic acids. For further details see Chapters 13 and 14.
ALCOHOLS
Monohydric aromatic alcohols
Included in this class is coniferyl alcohol, which forms an important component of the lignin molecule. Lignins are extremely complex phenylpropane polymers; they form an important strengthening material of plant cell walls and vary in composition according to their source, see Chapter 21, section on ‘Lignans and Lignin’.
Dihydric alcohols
Dihydric alcohols or glycols are compounds containing two hydroxyl groups; they are found naturally in many structural classes of compounds. The bicyclic amino alcohol 3,6-dihydroxytropane occurs free and as esters in a number of species of the Solanaceae and Erythroxylaceae, the dihydric alcohol panaxadiol is a component of some ginseng steroids, and oenanthotoxin, the poisonous constituent of the hemlock water dropworts (Oenanthe spp.), is a polyene diol.
ESTERS
Fats and fixed oils
As agricultural crops, seeds used for the extraction of fixed oils rate in importance second only to cereals. Over the last 60 years the production of oils for the food industry has increased enormously, whereas consumption by industrial and other users has remained relatively static but, in the pharmaceutical industry at least, not without interesting developments. Fixed oils are also obtained from fruit pericarps and in some instances such as the palm, Elaeis guineensis (Palmae), two oils differing in properties and chemical composition are obtained—the pleasantly flavoured palm kernel oil from the endosperm and palm oil from the orange-yellow fleshy pericarp. Oil seed crops are particularly advantageous commercially as following the expression of the oil a valuable high protein cattle feed remains. Also, such crops have benefited from plant breeding both regarding the yield and nature of the oil produced, and the morphology of the plant itself (see Chapter 14).
If the fatty acids represented by R1, R2 and R3 are the same, the triacylglycerol is known as a simple triacylglycerol—for example, tripalmitin on hydrolysis yields three molecules of palmatic acid. In nature, however, R1, R2 and R3 are usually different and the ester is known as a mixed triacylglycerol. On hydrolysis they frequently yieldboth saturated and unsaturated acids (Fig. 19.6); there is a strong tendency for unsaturated acids, particularly the C18 olefinic acids, to be linked to the secondary hydroxyl.
Biogenesis
Acylglycerols are formed from the fatty acyl-CoA or, more probably, the fatty acyl carrier protein (ACP) and L-α-glycerophosphate, as indicated in Fig. 19.7.
Quantitative tests
Under unsuitable storage conditions, such as exposure to light and air, fixed oils undergo secondary oxidation to give peroxides that then generate aldehydes and ketones. Such deterioration is detected by measurement of the peroxide value and anisidine value. The former is described by the Pharmacopoeia as ‘the number that expresses in milliequivalents of oxygen the quantity of peroxide contained in 1000 g of the substance, as determined by the prescribed methods’. These methods involve the liberation of iodine from a potassium iodide solution by the peroxides present in the sample and titration with 0.01 Msodium thiosulphate solution. For refined oils such as olive, borage, evening primrose and wheat-germ the typical value is 10; if these oils are to be used for parental dosage forms the maximum is 5. The maximum permissible value is higher for the virgin oils, e.g. olive = 20. Peroxide values are also used for fish oils, e.g. maximum value for farmed salmon oil, 5.0.
Certain physical constants of fixed oils and fats are significant: specific gravity, melting point, refractive index and sometimes optical rotation (e.g. castor oil). Table 19.6 shows how chemical standards are related to chemical composition. The gas chromatographic separation and quantification of the acids produced by the hydrolysis of specific fixed oils is an official method for their identification and quality control; type chromatograms are included in the BP/EP. Such detailed analyses often eliminate the necessity of rountinely applying all the above quantitative standards. Some examples of this application are given for the oils in Table 16.4. Comments on the detection of adulterants in the more expensive oils can be found under individual headings.
Waxes
While fats consist almost entirely of esters, waxes, in addition to esters of the cetyl palmitate type, often contain appreciable quantities of free acids, hydrocarbons, free alcohols and sterols. The hydrocarbons and sterols are unsaponifiable and both spermaceti and wool fat, which contain considerable quantities of these, have high saponification values. If analytical data for fats and waxes are compared, it will be noted that the acid values of waxes tend to be higher—for example, beeswax contains about 15% of free cerotic acid, C26H53COOH. In most waxes, iodine values are relatively low and unsaponifiable matter is high (Table 19.7).
DRUGS CONTAINING ACIDS, ALCOHOLS AND ESTERS
ROSELLE
The dried calyces and epicalyces of Hibiscus sabdariffa L., family Malvaceae, collected during the fruiting period, constitute the drug ‘roselle’. As an ornamental, the plant is grown globally in subtropical areas and the leaves, stems and seeds also find use as colourants, flavourings and as a source of fibre (rosella hemp). Its common name, Jamaica sorrel, arose following its early introduction to that country. Commercial supplies of the drug come principally from S.E. Asia, Egypt and the Sudan.
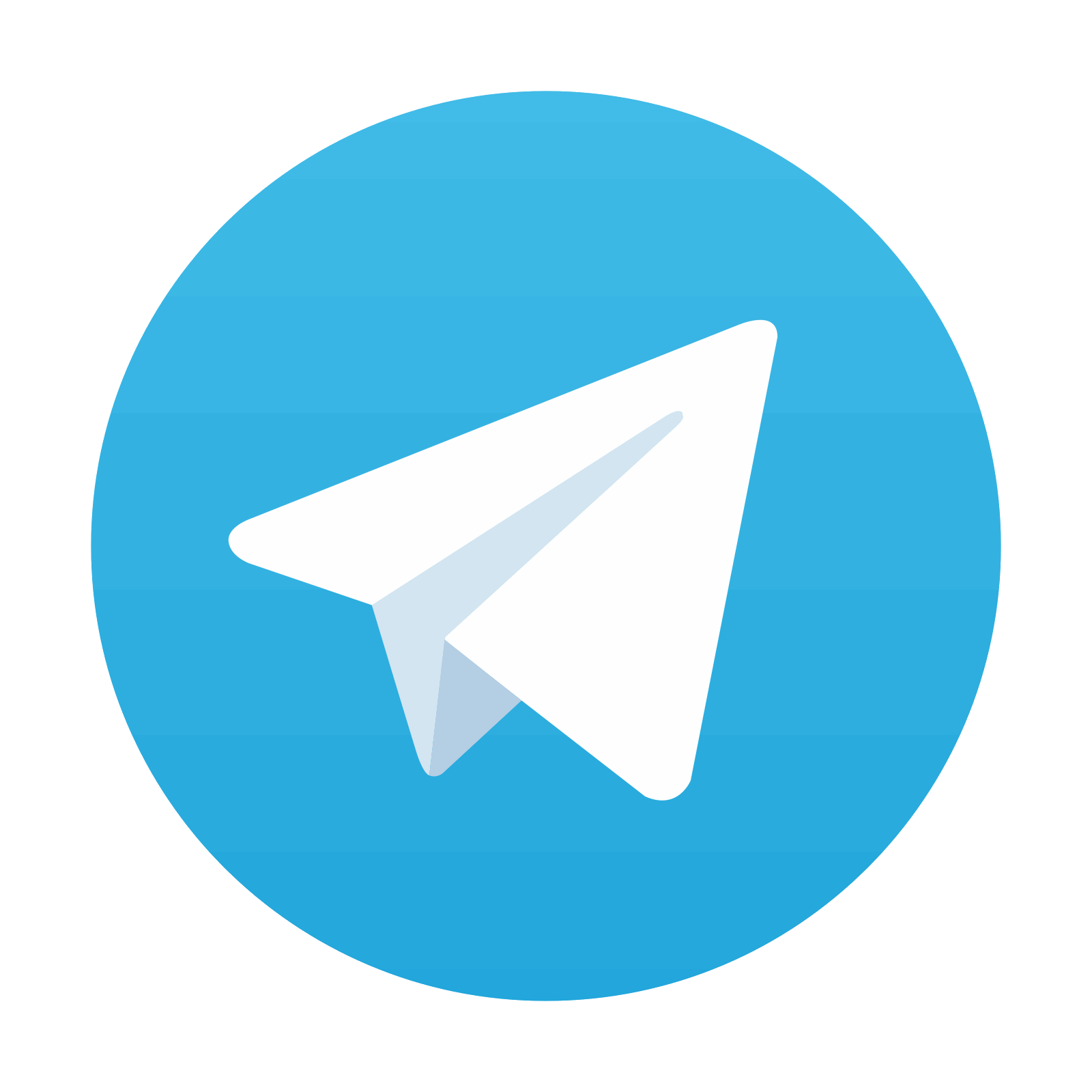
Stay updated, free articles. Join our Telegram channel

Full access? Get Clinical Tree
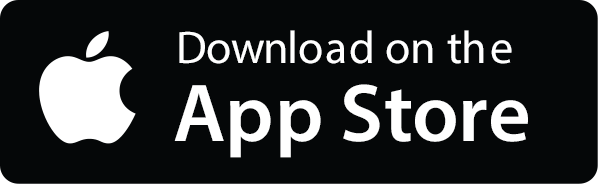
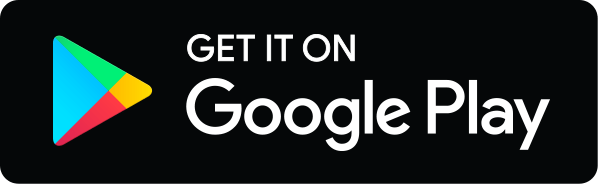