and Jürgen Roth2
(1)
Medical University of Vienna, Vienna, Austria
(2)
University of Zurich, Zurich, Switzerland
Junctional Complex
Cell to cell contacts are necessary for any higher organization of cells and provide the basis for the formation of tissues and organs. In epithelia, sheets of cells are formed by close attachment of cells to each other, which is facilitated by cell adhesion molecules and further stabilized and differentiated by formation of specialized cell junctions. In multiple epithelia, cells are attached to each other by junctional complexes, composed of a characteristic combination of occluding and adhering junctions that regulate paracellular traffic and stabilize the tissue. Junctional complexes are symmetrical structures formed between adjacent cells and consist of three components: a band of tight junctions, forming an occluding zone in the top position (zonula occludens, ZO, cf. also Fig. 99); a band of anchoring junctions in the middle position (belt desmosome, zonula adhaerens, ZA); and a circle of spot desmosomes in the bottom position (maculae adhaerentes, MA, cf. also Fig. 101). The complexes are localized mainly in the apical epithelial regions, as shown in panel A, where all three parts of a junctional complex of the small intestinal epithelium are on display (bracket), together with adhering interdigitated regions of the lateral cell surfaces (arrow).
The uppermost occluding zone (ZO) occupies cell surface areas close to the apical microvilli and determines the border between apical and basolateral regions of the plasma membrane. The plasma membranes of the neighboring cells are completely linked at regular intervals, the sealing sites forming interconnected ridges and networks that surround the cells completely. In the thin section of the occluding zone shown in panel A, the sealing ridges appear as connection points (“kissing points”). The anastomozing ridges are visible in freeze fracture replica electron micrographs shown on the next page (cf. Fig. 99).
The zonula adhaerens (ZA) is usually localized below the occluding zone. It also forms a belt surrounding the cells completely. In panel A, the adherent zone is cross sectioned. A flat section through the adherent belt at a connection site of three neighboring cells is displayed in panel B. The adherent belt is associated with densely packed actin filaments. In the cytoplasm nearby, multiple cross sections of the actin filament rootlets of the brush border microvilli extending into the terminal web are visible (arrows; cf. Fig. 88). Formation of belt desmosomes requires interactions of membrane-spanning cadherins (desmocollins, desmogleins), plaque proteins (desmoplakin, plakoglobin), and catenins. E-cadherin molecules bind to partners localized in the apposing plasma membrane of neighboring cells. At the cytoplasmic side, interactions of the E-cadherin tails with alpha- and beta-catenins and formin-1 induce actin polymerization and the assembly of the adherent belt-associated actin cytoskeleton, which is responsible for the cell-to-cell spreading skeleton and motion systems (cf. also Fig. 88). The unraveling of the molecular mechanisms of adherens junction organization with the involvement of actin, microtubules and endocytosis led to greater insight into the processes of tissue morphogenesis.
Spot desmosomes are shown in panel A (MA) and, at higher magnification, in panel C. In a circle-like arrangement below the belt desmosome, they represent the third part of junctional complexes, but they also exist independently of other cell contacts and, like buttons, attach cells to each other at multiple sites of the lateral cell surfaces. They are associated with intermediate filaments, bands of which build up interconnections between the individual spot desmosomes at the lateral walls of cells and the basal hemidesmosomes (cf. Fig. 109). The intercellular space is wider than it is in the adhering belt and is occupied by the extracellular glycosylated portions of cadherins appearing as a dense, zipper-like midline (arrow in panel C). Cytoplasmic plaques (arrowhead) attached to the plasma membrane function as anchors for the cadherins and for the intermediate filaments, which insert into the plaques in a hairpin-like fashion (cf. Fig. 101).
References
Bazzoni G (2003) The JAM family of junctional adhesion molecules. Curr Opin Cell Biol 15:525
Brieher WM, Yap AS (2013) Cadherin junctions and their cytoskeleton(s). Curr Opin Cell Biol 25:39
Ebnet K (2008) Organization of multiprotein complexes at cell-cell junctions. Histochem Cell Biol 130:1
Green KJ, Gaudry CA (2000) Are desmosomes more than tethers for intermediate filaments? Nat Rev Mol Cell Biol 1:208
Harris TJC, Tepass U (2010) Adherens junctions: from molecules to morphogenesis. Nat Rev Mol Cell Biol 11:502
Kobielak A, Pasolli HA, Fuchs E (2004) Mammalian forming-1 participates in adherens junctions and polymerisation of linear actin cables. Nat Cell Biol 6:21
Zigmond S (2004) Forming adherens junctions. Nat Cell Biol 6:12


Fig. 98
Magnification: ×88,000 (A), ×20,300 (B), ×120,000 (C)
Tight Junctions and Gap Junctions
Tight junctions have several major functions. They seal the intercellular space in epithelial and endothelial cell layers and prevent free paracellular passage of substances. They determine the polarity of epithelial cells by creating a boundary between the apical domain of the plasma membrane and the basolateral domain and prevent diffusion of lipids and proteins between them. Tight junctions recruit multiple cytoskeletal and signaling molecules at their cytoplasmic surfaces, which is seen in connection with regulatory processes involving the actinomyosin cytoskeleton and with intercellular adhesion signaling within epithelia and endothelia. Furthermore, actual cell biology research is focused on the roles of tight junction proteins in cell proliferation, transformations, and metastasis. Tight junctions may occur independently of other cell contacts but are more often part of junctional complexes forming an occluding belt in the top position (cf. Fig. 98).
In freeze-fracture replica electron micrographs, tight junctions are particularly visible as continuous, anastomozing strands of particles appearing on the protoplasmic face (P-face; TJ in panels A and B), forming a band or complex network. The number of tight junction strands is a crucial factor in determining the barrier properties of tight junctions. Transepithelial resistance increases with the number of parallel tight junction strands. The strands consist mainly of aggregations of the proteins claudin and occludin, and associated zonula proteins (ZO-1, ZO-2, and ZO-3). Individual strands associate with those of the apposing membrane of an adjacent cell to form paired strands and to establish the “kissing points,” visible in thin sections under the electron microscope (cf. Fig. 98). There is evidence that claudins form the backbone of tight junction strands. Not only proteins but also lipids are assumed to contribute to the formation of tight junction strands by forming inverted cylindrical micelles.
Most types of epithelia and endothelia contain occluding junctions. They have a crucial role in the formation of distinct barriers, such as the blood-brain barrier (cf. Fig. 178) and the blood-thymus barrier in endothelial cells, the blood-testis barrier built by the Sertoli cells, and the barrier sealing the bile canaliculi in the liver epithelium. The latter is shown in an in vitro culture system, using hepatoma cells that organize themselves three dimensionally and form anastomizing canaliculi lined by an apical dense microvilli border (panel A, inset). Peroxidase-conjugated wheat germ agglutinin, visualized by electron dense oxidized diaminobenzidine, labels both the apical microvilli membrane of the canaliculi and the basolateral plasma membrane, shown at the left-hand side, but is prevented from entering the tight junction area (TJ).
Gap junctions, or communicating junctions or nexus, are cell contacts providing cell-to-cell communication by transport of ions and small molecules up to approximately 1 kDa. They are formed by integral membrane proteins, the connexins. Six connexins assemble to form a hollow cylindrical structure called a connexon. Connexons align with connexon partners present in the apposing membrane of neighboring cells, forming hydrophilic channels of communication between the cytoplasms of adjacent cells. High Ca2+– concentrations lead to a closing of the connexon channels. Gap junctions allow chemical and electrical coupling of adjacent cells that are particularly critical for heart and smooth muscle cell action and regular embryogenesis.
The connexons are visible in freeze fracture replicas under the electron microscope, appearing as aggregated particles organized in spots or large areas (GJ in panel B). They can be distinguished clearly from the tight junction strands (TJ). Electron micrographs of thin sections show that the gap junction plasma membranes are closely apposed. The intercellular space is extremely regular and narrow but is visible as a gap none the less (panel B, inset), which is how the term “gap junction” was coined.
References
Capaldo CT, Farkas AE, Hilgarth RS, Krug SM, Wolf MF, Benedik JK, Fromm M, Koval M, Parkos C, Nusrat A (2014) Proinflammatory cytokine-induced tight junction remodelling through dynamic self-assembly of claudins. Mol Biol Cell E14:773
Fletcher SJ, Rappoport JZ (2014) Tight junction regulation through vesicle trafficking: bringing cells together. Biochem Soc Trans 42:195
Förster C (2008) Tight junctions and the modulation of barrier function in disease. Histochem Cell Biol 130:55
Kachar B, Reese TS (1982) Evidence for the lipid nature of tight junction strands. Nature 296:464
Kumar NM, Gilula NB (1996) The gap junction communication channel. Cell 84:381
Prochnow N, Dermietzel R (2008) Connexons and cell adhesion: a romantic phase. Histochem Cell Biol 130:71
Runkle EA, Mu D (2013) Tight junction proteins: from barrier to tumorgenesis. Cancer Lett 337:41


Fig. 99
Magnification: ×90,500 (A), ×34,000 (inset), ×71,000 (B), ×81,000 (inset)
Tunneling Nanotubes
Cells in diverse organs and also when grown in culture can communicate with each other by several means, including gap junctions (cf. Fig. 99) and chemical synapses (cf. Figs. 178 and 179). A novel type of cell-cell communication has been discovered, which is represented by tunneling nanotubes.
Tunneling nanotubes are membranous nanotubes with a diameter varying depending on the cell type. In the pheochromocytoma cell line PC12, their diameter is 50–200 nm and their length is up to several cell diameters, as shown in panel A. This is a scanning electron micrograph showing a tunneling nanotube bridging two PC12 cells (arrow), the cell type in which tunneling nanotubes were discovered. In contrast to other cellular protrusions, tunneling nanotubes of cultured cells do not contact the substratum and bridge two cells at their nearest distance. In panel B, a transmission electron micrograph of a tunneling nanotube (arrow) connecting two PC12 cells is shown. Panels C and D are higher-resolution micrographs from consecutive serial sections of this tunneling nanotube, which demonstrate the continuity between the tunneling nanotube membrane and the plasma membrane of the two connected cells (arrowheads). In all cell types analyzed, tunneling nanotubes contain F-actin. The seamless membrane contact provided by tunneling nanotubes permits the lateral diffusion of certain plasma membrane proteins and viruses between the connected cells. Probably equally important, tunneling nanotubes permit the intercellular transfer of organelles and cytoplasmic proteins and protein aggregates as well as ions. Organelles delivered between cells by tunneling nanotubes are represented by endosomal/lysosomal elements and mitochondria. Tunneling nanotubes have been reported to mediate bacterial communication.
Tunneling nanotubes are formed de novo and have a dynamic nature with a variable lifetime, thereby providing only transient cell-cell contacts. For their formation, actin polymerization appears to be important.
There is evidence for structural diversity of tunneling nanotubes. In PC12 cells and many other cell types, tunneling nanotubes are open-ended structures as described above and illustrated in the panels B–D. In T-cells, however, the tunneling nanotubes are of closed-end type. Here, the tunneling nanotubes formed by one cell only protrudes into an invagination of the other cell and establishes a junctional contact.
Although tunneling nanotubes were observed in many types of cultured cells, they appear to exist also in tissues where they probably participate in communicative processes in embryonic and adult tissues.
References
Costanzo M, Abounit S, Marzo L, Danckaert A, Chamoun Z, Roux P, Zurzolo C (2013) Transfer of polyglutamine aggregates in neuronal cells occurs in tunneling nanotubes. J Cell Sci 126:3678
Davis DM, Sowinski F (2008) Membrane nanotubes: dynamic long-distance connections between animal cells. Nat Rev Molec Cell Biol 9:431
Dubay GP, Ben-Yehuda S (2011) Intercellular nanotubes mediate bacterial communication. Cell 144:590
Gerdes HJ, Carvalho RN (2008) Intercellular transfer mediated by tunneling nanotubes. Curr Opin Cell Biol 20:470
Gousset K et al (2009) Prions hijack tunnelling nanotubes for intercellular spread. Nat Cell Biol 11:328
Gurke S, Barroso JF, Gerdes HH (2008) The art of cellular communication: tunneling nanotubes bridge the divide. Histochem Cell Biol 129:539
Hase K et al (2009) M-Sec promotes membrane nanotube formation by interacting with Ral and the exocyst complex. Nat Cell Biol 11:1427
Hodneland E, Lundervold A, Gurke S, Tai XC, Rustom A, Gerdes HH (2006) Automated detection of tunneling nanotubes in 3D images. Cytometry A 69:96
Rustom A, Saffrich R, Markovic I, Walther P, Gerdes HH (2004) Nanotubular highways for intercellular organelle transport. Science 303:1007
Sherer NM (2013) Long-distance relationships: do membrane nanotubes regulate cell–cell communication and disease progression? Mol Biol Cell 24:1095
Sowinski S et al (2008) Membrane nanotubes physically connect T cells over long distances presenting a novel route for HIV-1 transmission. Nat Cell Biol 10:21


Fig. 100
Magnification: ×5,800 (A); ×7,500 (B); ×40,000 (C, D)
Spot Desmosomes
Spot desmosomes (maculae adhaerentes) are anchoring junctions associated with intermediate filaments. They are arranged in circles in the lower-most position of junctional complexes (cf. Fig. 98) but are common also outside complex junctions, occurring independently of other cell contacts. Spot desmosomes are particularly abundant in all tissues that are exposed to mechanical stress. Like buttons, they link neighboring cells to each other and help to stabilize cells and tissues and make them resistant to mechanical injuries. However, it is becoming clearer that spot desmosomes do not merely play a mechanical role by welding cells together. They also perform signaling functions and are considered to have a role as sensors that respond to cellular and environmental signals by changing their organization and modulating their assembly state. Intracellular calcium homeostasis is crucial for desmosomal adhesion.
Panel A shows a segment of the stratified epithelium of the spinous layer of the epidermis (cf. Figs. 138 and 139). Within the extended interdigitations, the neighboring keratinocytes are attached to each other by numerous spot desmosomes (arrows). Intermediate (cytokeratin) filaments, called tonofilaments, form thick bundles of tonofibrils (TF). They build up stabilizing cables within the cytoplasm, and are anchored in the plaques of the spot desmosomes (arrows). At higher magnification (inset), the dense midline and the inner and outer zones of the plaques, where the tonofilaments attach, are visible. The arrowheads in panel A point to melanin granules.
In panel B, cytokeratin in the epidermis is localized by immunogold labeling by the use of an anti-cyto-keratin monoclonal antibody recognizing a conserved epitope localized on the surface of cytokeratin filaments. Gold particles label in the outer parts of the dense desmosome plaques (arrows), where cytokeratin filaments are anchored by interacting with desmoplakin, which is one of the plaque proteins. Desmoplakin, via other plaque proteins, plakoglobin and plakophyllin, in turn is connected with membrane-spanning proteins of the cadherin family, desmocollins and desmogleins. Heterophilic rather than homophilic interactions of desmocollins and desmogleins are required for desmosomal adhesion and are responsible for the electron-dense midline visible under the electron microscope (inset and Fig. 98C). Results point to different roles of cadherins in the assembly of the desmosome complex and regulation of its structural integrity. Furthermore, studies on the presence of desmosomal cadherins outside of desmosomes and their functions in adhesion-dependent and adhesion-independent signaling provide increased insights into mechanisms involved in pemphigus skin blistering.
References
Chiraev NA, Troyanovsky SM (1997) Direct Ca2+-dependent heterophilic interaction between desmosomal cadherins, desmoglein and desmocollin, contributes to cell-cell adhesion. J Cell Biol 138:193
Franke WW, Winter S, von Overbeck J, Gudat F, Heitz PU, Stahli C (1987) Identification of the conserved, conformation-dependent cytokeratin epitope recognised by monoclonal antibody (lu-5). Virch Arch A Pathol Anat Histopathol 411:137
Green KJ, Gaudry CA (2000) Are desmosomes more than tethers for intermediate filaments? Nat Rev Mol Cell Biol 1:208
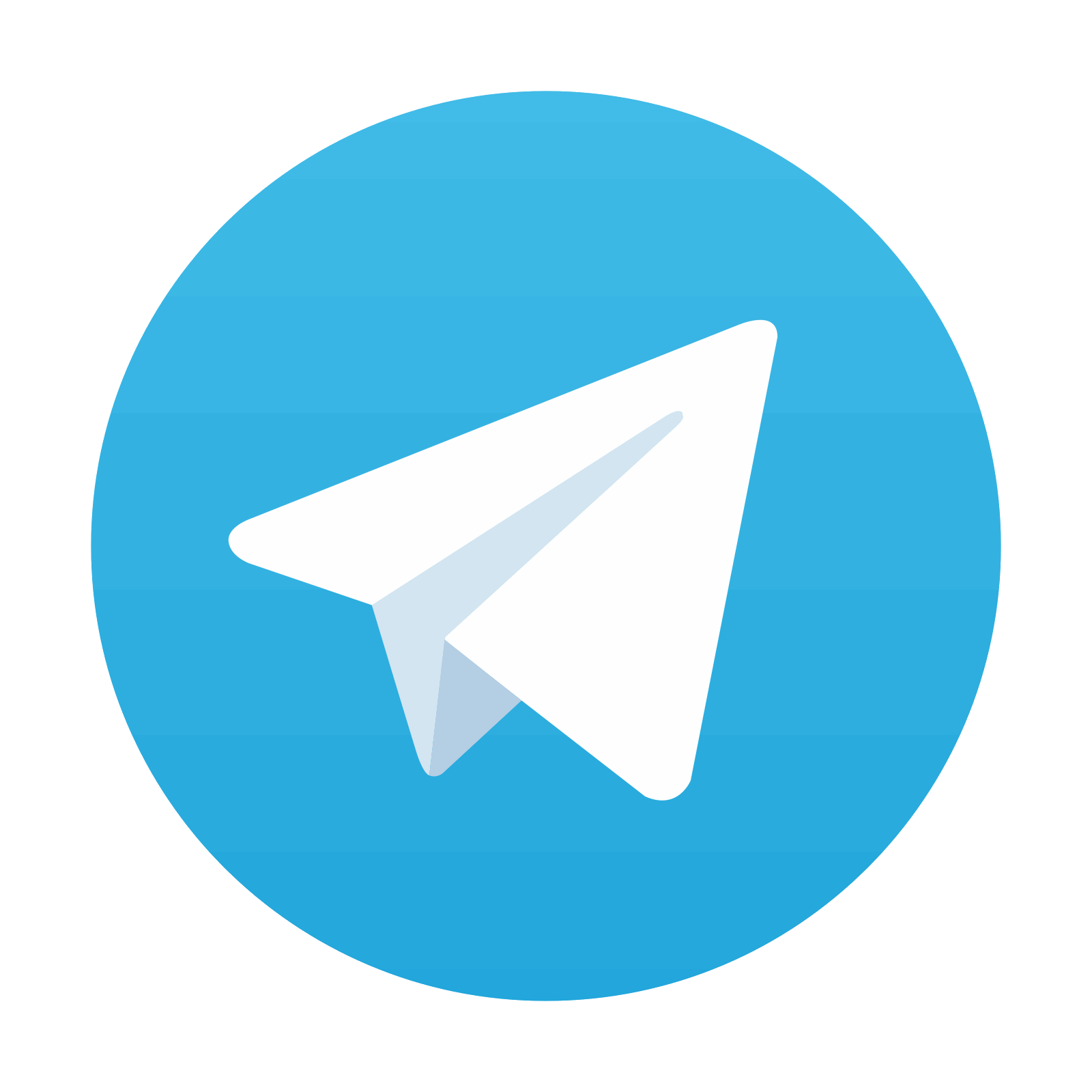
Stay updated, free articles. Join our Telegram channel

Full access? Get Clinical Tree
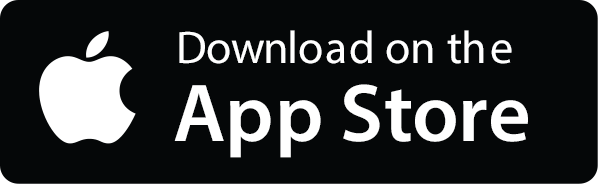
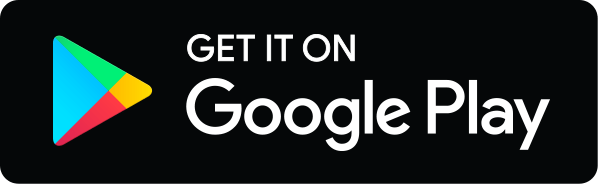