Anatomy, physiology, and classification of the generators of auditory evoked potentials
ANATOMY OF THE EAR AND THE AUDITORY PATHWAY
External auditory meatus and the middle ear
The anatomy of the ear is shown in Figure 2.1, and some of its structures will be described in order to clarify their function in transforming acoustic stimuli into mechanical oscillations and in conducting the stimuli through into the inner ear.
Fig. 2.1 Anatomy of the external auditory meatus, middle ear, inner ear, and internal auditory meatus. (Reproduced with permission from Nicol T & Chao-Charia K K 1981.)
The external auditory apparatus consists of the pinna and external ear canal, which is divided into a lateral membrano-cartilaginous part and a medial bony part. The external auditory meatus has a depth of approximately 28 mm and a diameter of approximately 7 mm. However, the shape of the canal varies to some extent among subjects. Its direction is anteromedial, and the meatus terminates at the tympanic membrane.
The tympanic membrane is approximately 10 mm high and 8 mm long anteroposteriorly, and it resembles the conical diaphragm of a loudspeaker, with a surface area of about 80 mm2. The larger, vibrating part of the eardrum has a fibrous layer and is called the pars tensa. The smaller, upper portion of the eardrum has little or no fibrous layer and is called the pars flaccida.
The middle-ear cavity is ventilated through the Eustachian tube and contains the articulating ossicles, which are supported by ligaments. The malleus is attached to the tympanic membrane and articulates with the incus. The incus is connected to the head of the stapes, and the footplate of the stapes is, in area, about 3.5 mm2 and fits into the oval window of the bony labyrinth. The annular ligament surrounds the footplate and allows the stapes to move in the oval window. The tensor tympani muscle is attached to the malleus, and the stapedial muscle to the stapes: these muscles pull in opposite directions and modify the motion of the ossicles. The ossicular chain transmits sound to the cochlea, and the pivotal point of this motion goes through the intermediate ossicle, the body of the incus. The manubrium of the malleus is about 1.3 times longer than the long process of the incus which together can be modelled by a folded lever (Zwislocki 1965).
Cochlea and auditory nerve
As the neuro-anatomy of hearing is extremely complex, only the main anatomical sources pertinent to generation of the different evoked potentials will be mentioned in this chapter. The schematic anatomy of the organ of Corti is shown in Figure 2.2.
Fig. 2.2 Transverse section of the cochlear duct (A) and organ of Corti (B).The potentials within the endolymph and the hair cells are shown. This leaves a large potential difference of about 140 mV across the upper surface of the hair cells. (After (A) Pickles J O 1985 and (B) Durrant J D & Lovrinic J H 1984, with permission.)
The organ of Corti is about 35 mm long and is inside the cochlear duct. It consists of sensory hair cells of 10 μm in diameter and is bounded by the basilar membrane. The sensory cells are surrounded by a variety of supporting cells and by the overlying, gelatinous, tectorial membrane (Fig. 2.2). There is a single row of about 3500 inner hair cells (IHC) and three or four rows of 12 000 outer hair cells (OHC), according to Spoendlin (1986). The outer and inner hair cells are separated by the pillar cells forming the tunnel of Corti, which is filled with the fluid, corticolymph.
Both inner and outer hair cells have the characteristics of mechanoreceptors. The microvilli, or stereocilia, on the surface of the hair cells project towards the tectorial membrane. It is thought that some stereocilia of the outer hair cells are embedded in this membrane. The stereocilia of the same row on each hair cell are joined by horizontally running links. The shorter stereocilia tips also give rise to vertical links, which run upwards to join the taller adjacent stereocilia of the next row (Rhys Evans et al 1985). The movement of the basilar membrane with the endolymph in the cochlear duct produces the shearing movement of the stereocilia and distortion of the link, a movement which is responsible for bio-electrical transduction.
About 30 000 afferent fibres pass to the hair cells. The cell bodies of the first-order neurones or ganglia are situated in the spiral bony canal in the modiolus. The fibres vary in diameter from 1 to 8 nm, and, as a rule, those connecting to the inner hair cells are thicker than those connecting to the outer hair cells (Gacek & Rasmussen 1961). There is a correlation among fibre size, spontaneous activity, and sensitivity – the thicker fibres being more sensitive and efficient (Liberman 1982). The fibres lose their myelin sheath as they pass into the basilar membrane through small bony canals in the habenula perforata.
About 95% of the afferent neural fibres pass directly to the IHC, while 5% of the afferent fibres cross the tunnel of Corti, mostly in a radial direction, and turn towards the three rows of outer hair cells (see Fig. 2.2). The latter fibres are longer and thinner, and travel towards the basal turn of the cochlea for from one-third to one-fourth of the length of the basilar membrane. It is assumed that they represent a less efficient system than do thicker fibres for transmission of information (Liberman 1982). Thus, it appears that innervation of the outer hair cells is convergent – one neurone communicating with as many as about 10 hair cells – and that innervation of the inner hair cells is divergent – one inner hair cell attaching to many neurones. In general, IHC have a rich afferent nerve supply in contrast to OHC, which have poor afferent innervation and a rich efferent nerve supply (Spoendlin 1986).
The cochlea is innervated by ipsilateral and contralateral efferent fibres. Thin, unmyelinated, efferent fibres make contact with the afferent fibres of the IHC. About 500 efferent fibres of the contralateral olivocochlear bundle cross the tunnel of Corti. They are thought to innervate the outer hair cells and to have a presynaptic inhibitory effect. The good efferent supply and poor afferent system of the OHC suggest that they have an important role at the receptor level, and that the main information transfer relies on the inner hair system and its more efficient afferent pathways (Spoendlin 1986).
Auditory pathway
In this chapter, the afferent auditory pathway is outlined so that its anatomy can be associated with the electrophysiology and generation of evoked potentials in different parts of brain. This pathway is shown schematically in Figure 2.3, which is based on descriptions and reviews by Stelmasiak (1954), Galambos (1958), Crosby and Humphrey (1962), Moller (1983), and Harrison (1987).
The fibres of the first-order neurones originating from the ganglia form the cochlear nerve and enter the brainstem in the upper medulla, terminating in the second-order neurones of the dorsal cochlear nucleus (DCN) or of the anterior and posterior ventral cochlear nucleus (AVCN and PVCN) on the same side. These primary acoustic nuclei constitute neuro-anatomically separate areas and have been fully described by Lorente de No’ (1981).
The fibres originating in the ventral cochlear nucleus form synapses with the third-order neurones on both sides in the subnuclei of the superior olivary complex situated in the pontomedullary level. Those fibres from both sides cross over in the ventral stria of the trapezoid body. Fibres from the dorsal cochlear nucleus also cross over, in the dorsal stria of Monakov. In this way, the superior olivary complex, consisting of the lateral nucleus of the superior olive (LSO), the medial nucleus of the superior olive (MSO), and the medial nucleus of the trapezoid body (MTB), receives and relays input from both ears.
Both crossed and uncrossed fibres arising from the cochlear nucleus do not necessarily terminate in the superior olivary complex, but, instead, they may go directly to the inferior colliculus, or they may terminate in the nucleus of the lateral lemniscus.
Most of the third-order neurones from the superior olivary complex pass through the lateral lemniscus, relaying input from the opposite ear, and terminating in the neurones in the inferior colliculus. From there they relay input to the medial geniculate body in the thalamus. There are also interconnections between the two sides at the level of the inferior colliculi. Collaterals from the brainstem auditory pathway go to the reticular formation and the cerebellum.
From the geniculate body the fibres of the fourth-order neurones pass through the acoustic radiation into the tonotopically arranged primary auditory cortex in the temporal lobe. The areas surrounding the primary auditory cortex also participate in auditory function; they are known as secondary and associated auditory areas.
There are also descending corticocochlear efferent pathways, the most peripheral of which are connections to the cochlea from the well-formed crossed and uncrossed fibres from the olivocochlear bundle of Rasmussen (1942), Ward (1978).
BASIC PHYSIOLOGY OF THE AUDITORY SYSTEM
The outer and the middle ears have an influence on the sound spectrum reaching the ear, and the normal ear in man tends to be most effective in transmitting the frequencies between 2000 and 3000 Hz at low-stimulus intensities (Dallos 1976).
The role of the external and middle ears is to improve the impedance match between air and the higher impedance of the fluid medium in the cochlea (Tonndorf & Khanna 1976). In the external ear, there is amplification due to the shape of the pinna, diffraction around the head and auricle, and the resonance of the ear canal, all of which combine to give a maximum gain of 20 dB at 3000 Hz (Tonndorf & Khanna 1976). In the middle ear, the major anatomical factor is that the ratio of the large effective area of the tympanic membrane to the small area of the footplate of the stapes is about 18.6. This means that the sound pressure is amplified 18.6 times. Additional amplification of about 1.3 times is caused by the leverage effect of the ossicles; all together, the sound pressure is amplified by about 24 times (1.3 × 18.6) (Zwislocki 1965, 1975). However, one should bear in mind that the sound pressure transmission is effective only if there is sufficiently rapid vibration of the stapes. The mechanical transmission of the middle ear causes a displacement of the basilar membrane, leading to mechano-electrical effects which generate the cochlear microphonic (CM). The CM magnitude, in the basal turn of the cochlea, is largely determined by the middle-ear transmission and by the velocity of the movement of the stapes (Dallos 1976).
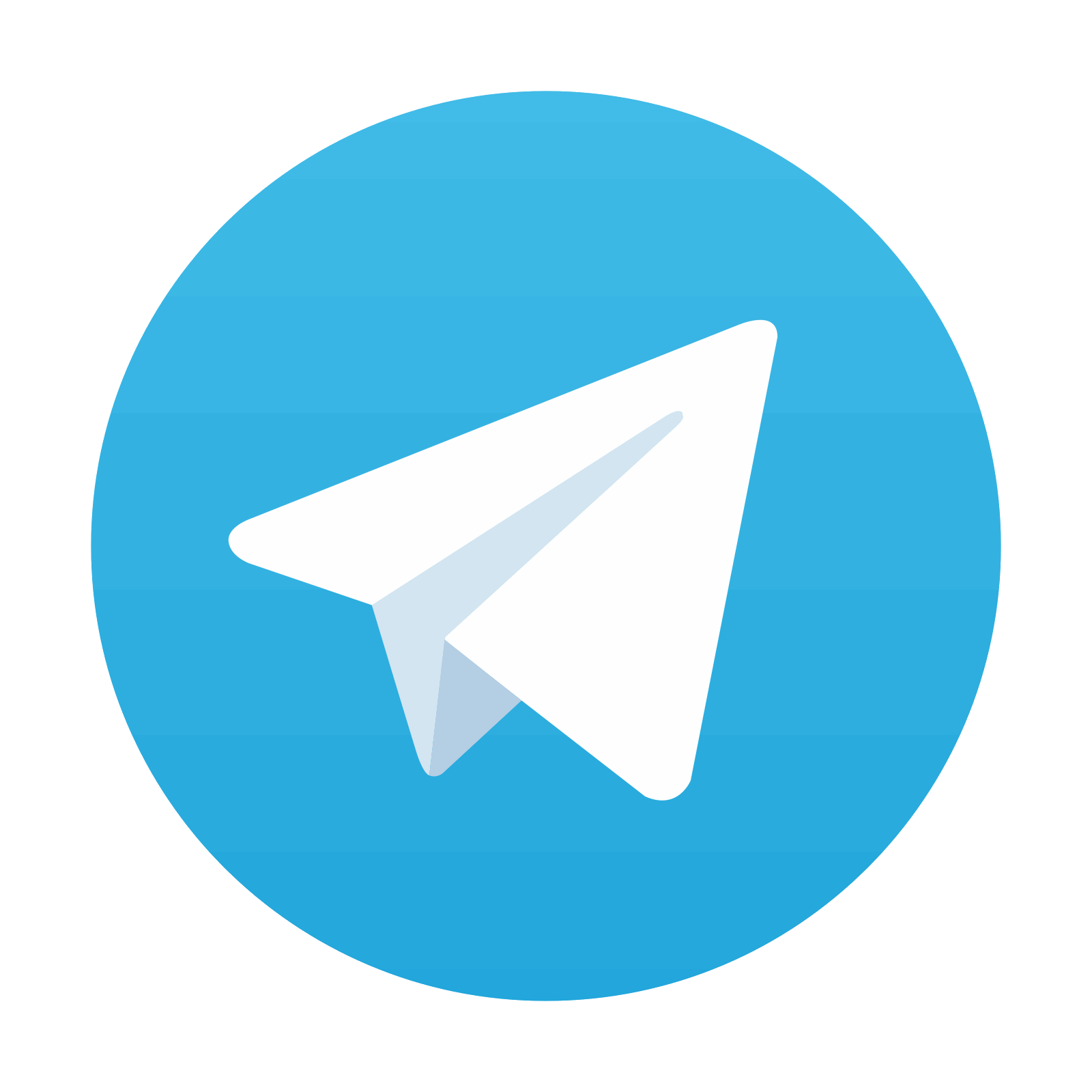
Stay updated, free articles. Join our Telegram channel

Full access? Get Clinical Tree
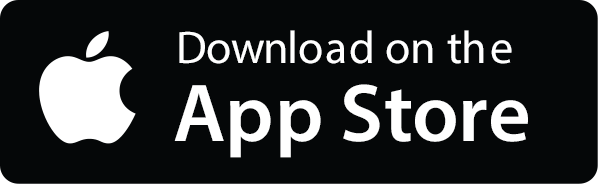
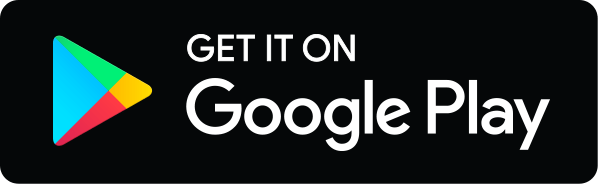