Chapter 5 Analytical techniques
It is important that any oil used in aromatherapy is of the highest quality and purity. Essential oils have widespread applications including perfumery, pharmaceutical products and food flavouring. It is estimated that only 5% of all essential oils produced are used in aromatherapy.
The main technique used is gas–liquid chromatography (abbreviated GLC or nowadays just GC), which is especially useful when combined with mass spectroscopy (MS); the combination is often referred to as GC-MS. They can provide both quantitative and qualitative information that is very accurate and reliable when compared to known analytical measurements of oils that are stored in databases.
CHROMATOGRAPHY
Gas–liquid chromatography (GC or GLC)
In GC the mobile phase is a gas and the stationary phase is a liquid. It is one of the most widely used techniques for separation of materials and for analysis and can give both qualitative and quantitative information about a sample.
A typical GC apparatus is shown in Figure 5.1.
A typical GC analysis chart is shown for a geranium oil in Figure 5.2.
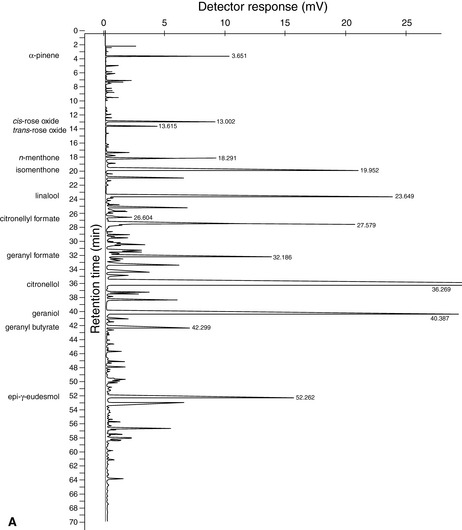
Figure 5.2 Results of GC of geranium oil. (A) This shows the chromatogram, or printed chart, in an analysis that has run for 70 minutes (see the horizontal axis). (B) These are the type of data you would expect to get along with the chart. (Column 1) Peak number in order of retention times – the lower the number, the quicker the compound passes through the column, i.e. the faster it moves in the mobile phase. The order of the peaks represents the volatilities of the compounds: the monoterpenes come off first, sesquiterpenes and their oxygenated compounds in the middle, with the compounds of low volatility last. (Column 2) Peak name identifies the compound. (Column 4) Retention time is the time the vaporized compound takes to pass through the column. (Column 3) Result percentages. These figures are measures of the areas of individual peaks expressed as a percentage of the total area of all of them. This means that all the individual peak areas should add up to 100%. The figures are calculated automatically by a special computer called an integrator. In practice the relative area of each peak is not precisely proportional to the percentage of the corresponding constituent in the essential oil; this has to be worked out using an additional response factor for the substance. For this sample the results show identification of 13 major components out of 289, making up 81.25% of the total components. The volatile monoterpene -pinene (peak 1) is the first off the column. The alcohols citronellol (10) and geraniol (11) are responsible for the odour characteristics of geranium, which is lifted and activated by the two rose oxides (2 and 3). The 6,9-guaiadiene (7) is a non-terpene hydrocarbon that acts as a back note but it is not a powerful odour. Component 12 is geranyl butyrate; component 13 is epi- -eudesmol. Chromatograms and data supplied by Jenny Warden of Traceability.
MASS SPECTROMETRY (MS)
The ions produced are then formed into a beam and accelerated in a magnetic field and deflected by another magnetic field. For ions with the same charge, the deflection in the magnetic field is greatest for ions of lowest mass. The degree of deflection also depends on the amount of charge on the ion – more highly charged ions will be deflected more than ions of the same mass with a lower charge. These two factors are taken into account by the mass spectrometer, which records the relative abundance of each type of particle in terms of its mass (m) to charge (z) ratio (m/z).
Figure 5.3 shows the typical arrangement of a mass spectrometer. Figure 5.4 shows MS charts for the two monoterpenes [ ]-pinene and limonene, and the oxide 1,8-cineole.
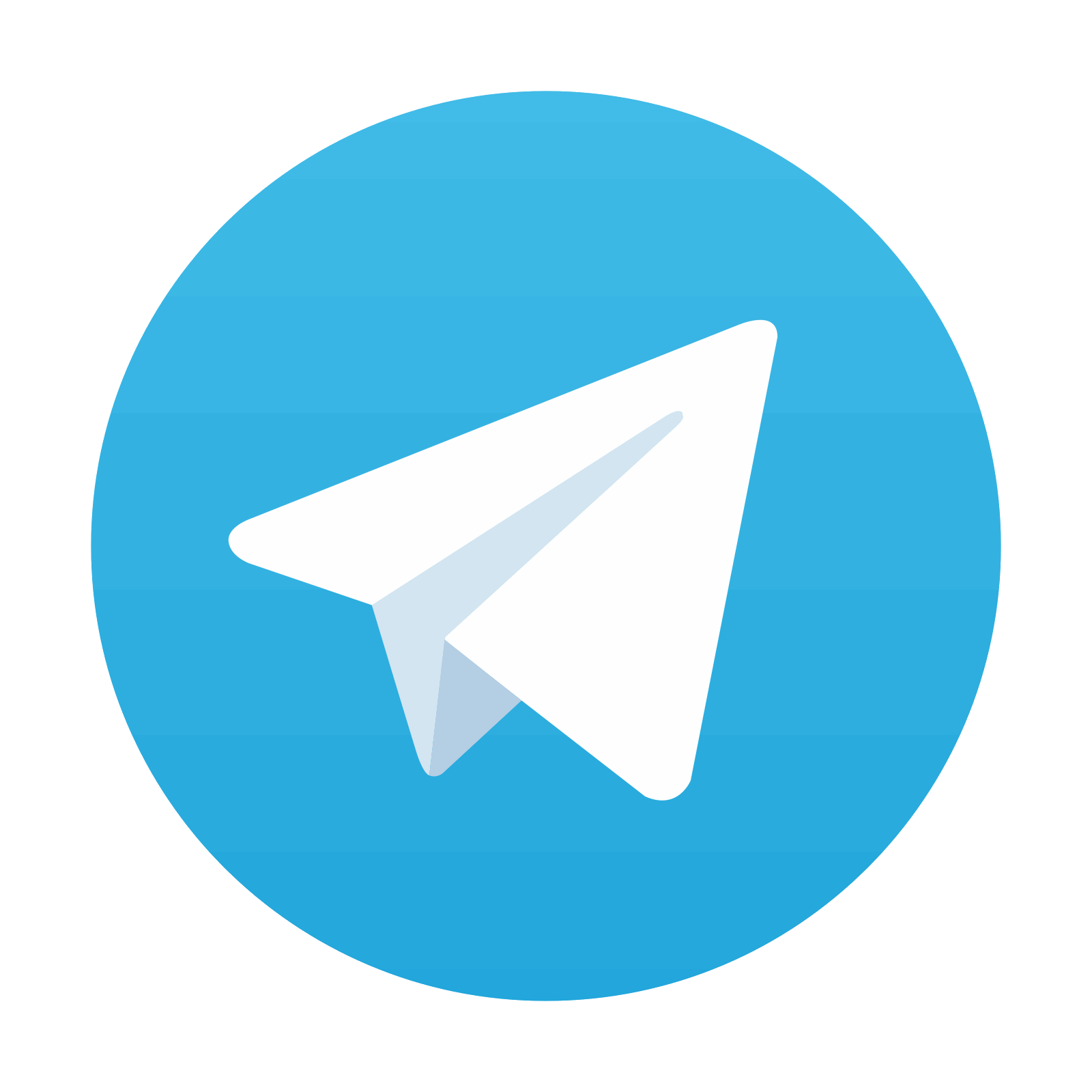
Stay updated, free articles. Join our Telegram channel

Full access? Get Clinical Tree
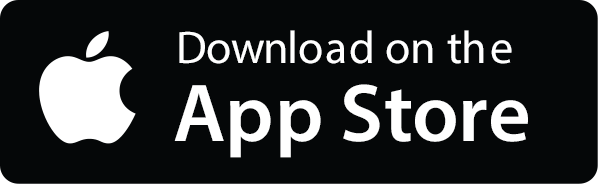
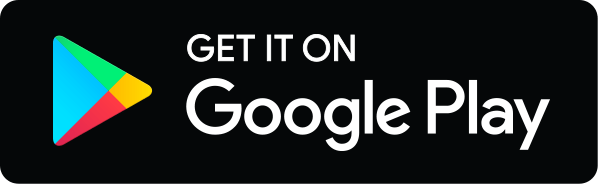