Defective precursor proliferation, e.g. iron deficiency, anaemia of chronic disorder, marrow aplasia or infiltration

There are three key dietary factors that are required for normal red cell synthesis, referred to as haematinics:
Iron
Dietary iron is absorbed from the duodenum and upper jejunum. In an omnivorous diet most iron is absorbed from meat, in which it is present as haem. Haem is the ferrous form of iron (Fe2+) complexed with a porphyrin ring. Haem is readily absorbed from the gut, but non-haem iron in a vegetarian diet, which is mainly in the ferric state (Fe3+), is inefficiently absorbed. Absorption of ferric iron is facilitated by several factors:

Within enterocytes, iron is oxidized to the ferric state and transported to the circulation by the protein ferroportin. In the blood, ferric iron is bound to the globulin transferrin and transported to the bone marrow and iron stores. Cellular iron uptake occurs via transferrin receptors, and in most cells iron is stored as ferritin (a complex of iron with the apoferritin protein). In some tissues iron is also found as relatively insoluble aggregates of degraded forms of ferritin, known as haemosiderin. Two-thirds of the iron in the body is present in circulating red cells, and about half of the remainder is found in macrophages, reticuloendothelial cells and hepatocytes. The rest is present in myoglobin in muscle cells or associated with various intracellular enzymes.
When ageing red cells are broken down by the reticuloendothelial system, most of the released iron is recycled via macrophages for further erythropoiesis. Iron loss from the body is normally low, and occurs through shedding of mucosal cells containing ferritin; there is negligible renal loss of iron.
Iron deficiency
The main cause of iron deficiency in the UK is abnormal loss of blood, particularly from the gut or from exaggerated menstrual loss. Iron malabsorption can result from disease of the upper small intestine, for example coeliac disease, or following partial gastrectomy. Dietary deficiency is rarely a major cause in Western societies, although worldwide a vegetarian diet low in absorbable forms of iron is the commonest contributory cause of iron deficiency.
Therapeutic iron preparations
Oral iron: Oral iron supplements are preferred and are given as ferrous salts; for example, ferrous sulphate, fumarate or gluconate. Tablets are normally used, but some people find that a syrup is more palatable. In the presence of iron deficiency, a daily oral dose equivalent to 100–200 mg of elemental iron produces the maximum rate of rise of haemoglobin (200 mg ferrous sulphate contains 65 mg iron). About one-third of this dose will be absorbed. Some oral iron preparations contain vitamin C, but the therapeutic advantage is minimal compared to the ferrous salt alone.

Parenteral iron: Iron can be given by slow intravenous injection or infusion, or less commonly by deep intramuscular injection. Formulations involve complexing ferric hydroxide to a carrier to form iron sucrose, iron dextran (the only formulation for intramuscular use), ferric carboxymaltose or iron isomaltoside 1000. The iron in these formulations is not bound to transferrin in plasma but accumulates in reticuloendothelial cells. When calculating the amount of iron to give, the approximate total body iron deficit (haemoglobin and body stores) is estimated from the person’s size and haemoglobin concentration.
Therapeutic use of iron
The cause of iron deficiency should always be sought when starting symptomatic treatment with iron. If this is not done, then serious disorders such as gastrointestinal malignancy can be overlooked. Oral iron supplements are adequate for most mild or moderate iron-deficiency anaemias. After an initial delay of a few days while new red cells are formed, oral iron supplements should raise the blood haemoglobin concentration by about 20 g⋅L−1 over the first 3–4 weeks, and about 10 g⋅L−1 per week thereafter. Oral iron supplements should be continued for 3 months after the haemoglobin concentration has been restored, in order to replenish tissue iron stores.
Failure to respond to oral iron can be caused by several factors:
Parenteral iron preparations are used if there are intractable unwanted effects from oral preparations, if there is severe uncorrectable malabsorption or continuing heavy blood loss and when adherence to oral treatment is poor. Parenteral iron does not raise the haemoglobin concentration any faster than oral iron, except during haemodialysis for severe renal failure.
Oral iron supplements are occasionally given for prophylaxis against iron deficiency at times of high demand for iron, for example pregnancy, menorrhagia or if there is a poor dietary intake. The reduced iron absorption after subtotal or total gastrectomy can also be overcome by long-term iron supplements.
Folic acid
Folate is required for a number of cellular biochemical processes, including DNA synthesis, and is essential for cell replication, including the formation of red cells. Folic acid (pteroylglutamic acid) is ingested as conjugated folate polyglutamates, found mainly in fresh leaf vegetables (in which it is heat-labile) and in liver (where it is more heat-stable). Before absorption, the polyglutamates are deconjugated to the monoglutamate. Folate monoglutamate is absorbed principally in the duodenum and jejunum, and is methylated and reduced to 5-methyltetrahydrofolate by dihydrofolate reductase during absorption. Methyltetrahydrofolate enters cells, where it is demethylated and converted back to folate polyglutamates. These are coenzymes in the synthesis of pyrimidines and purines and hence of DNA (see also Ch. 52).
Folate deficiency
The most obvious consequence of folate deficiency is a macrocytic anaemia with the presence of megaloblasts in the marrow, a feature it shares with vitamin B12 deficiency. Folate deficiency can arise for a number of reasons (Box 47.3). Unlike iron, folate cannot be recycled from old red cells that are removed from the circulation.
Therapeutic use of folic acid
Folate deficiency almost always responds to oral folic acid supplements. Folic acid is a poor substrate for dihydrofolate reductase, and is largely absorbed unchanged and then converted to tetrahydrofolic acid in the plasma and liver. Most causes of folate deficiency are self-limiting, and folic acid treatment is usually given for 4 months to correct the anaemia and replace folate stores.
Folic acid is given prophylactically in pregnancy. It is given in higher doses if there is an increased risk of conceiving a child with a neural tube defect. Those at higher risk include a partner with a neural tube defect, history of neural tube defect in a previous pregnancy, or if the woman has coeliac disease, diabetes mellitus, sickle-cell anaemia or is taking antiepileptic drugs (Ch. 23). Folic acid is also given prophylactically to premature infants, during renal dialysis, and for chronic haemolytic anaemia.
Treatment of deficiencies of both vitamin B12 and folate using only folic acid may correct the anaemia, but irreversible neurological damage can be precipitated (see below). Therefore, vitamin B12 deficiency must be excluded before folic acid is used, or both vitamin B12 and folic acid should be given if there is a possibility of vitamin B12 deficiency.
For folate deficiency produced by drugs that inhibit dihydrofolate reductase (e.g. methotrexate; Ch. 52 and Fig. 51.4) it is necessary to ‘bypass’ this enzyme blockade by giving the synthetic tetrahydrofolic acid, folinic acid (5-formyl tetrahydrofolic acid). This is the basis of ‘folinic acid rescue’ to reduce the toxic effects on healthy tissues of high-dose methotrexate used for treatment of malignancy (Ch. 52). Folinic acid is formulated as a salt and given orally, usually as calcium folinate. When low-dose methotrexate is used in a once-a-week regimen for immunosuppression folic acid can be given on a separate day to reduce toxicity.
Vitamin B12
Vitamin B12 has many roles in the body, including participation in DNA synthesis and fatty acid synthesis. The term vitamin B12 refers to a group of cobalt-containing compounds, also known as cobalamins. Bacteria are the only organisms that can synthesise cobalamins de novo. Humans obtain vitamin B12 from meat (particularly liver), from animal products (milk, cheese, eggs, etc.) or from vegetables contaminated by bacteria. Absorption is by an unusual mechanism: dietary vitamin B12 binds in the stomach to a glycoprotein called intrinsic factor that is produced by gastric parietal cells. This complex is absorbed principally from the terminal ileum after binding to receptors on the luminal membranes of ileal cells.
Most vitamin B12 in plasma is bound to a glycoprotein, transcobalamin I, from which it is rapidly taken up by the tissues, especially the liver, which stores about 50% of the body content of vitamin B12. A second protein, transcobalamin II, is mainly responsible for rapid transport of vitamin B12 to tissues, and for enhancing its uptake by the bone marrow via specific receptors. Vitamin B12 is essential as a coenzyme in nucleic acid synthesis, and in other metabolic pathways in conjunction with folate. Many functions of vitamin B12 can be performed by folic acid, but there are two enzyme families that only vitamin B12 can facilitate. These are responsible for isomerisation of methylmalonyl coenzyme A to succinyl coenzyme A, isomerisation of α-leucine to β-leucine, and methylation of homocysteine to methionine (a reaction that results in demethylation of methyltetrahydrofolate).
Vitamin B12 deficiency
Impairment of vitamin B12-dependent enzyme reactions affects DNA synthesis. The major organs affected by vitamin B12 deficiency are those with a rapid cell turnover, particularly the bone marrow and the gastrointestinal tract.
Vitamin B12 deficiency presents with a macrocytic anaemia and a megaloblastic bone marrow. The tongue becomes smooth, and changes to the lining of the small bowel can lead to malabsorption. Damage to the posterior and lateral neuronal tracts in the spinal cord can also occur, leading to a condition known as subacute combined degeneration of the cord. The biochemical basis for the neurological damage is poorly understood, and it may not be fully reversible after correction of vitamin B12 deficiency.
Causes of vitamin B12 deficiency include:
< div class='tao-gold-member'>
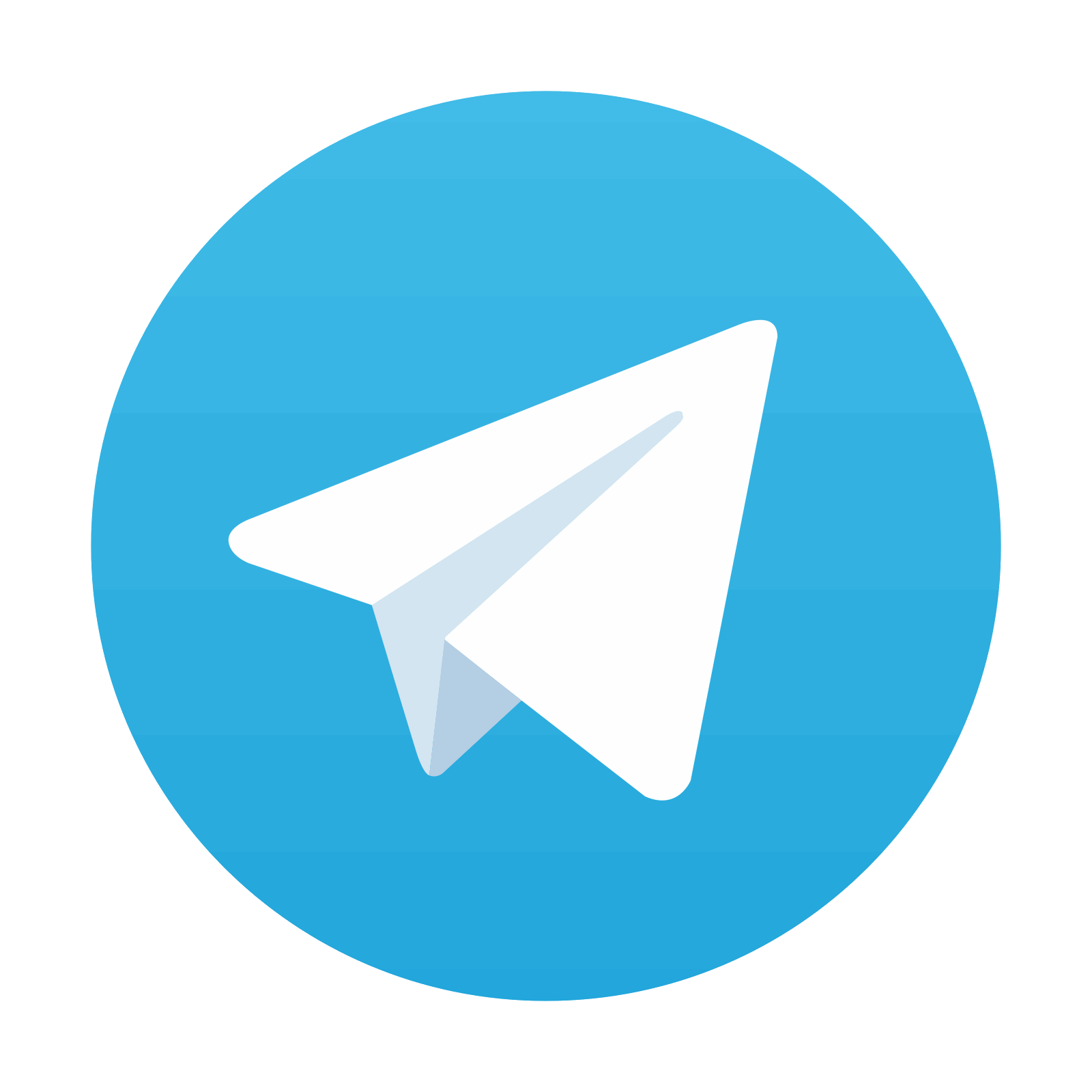
Stay updated, free articles. Join our Telegram channel

Full access? Get Clinical Tree
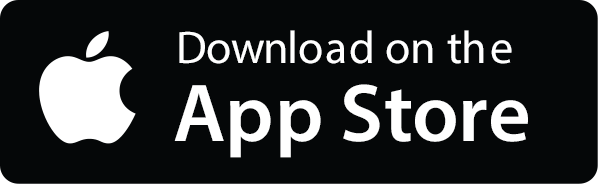
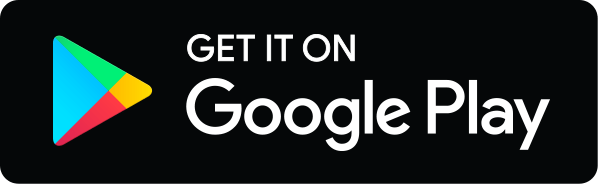