Fig. 1.1
Regenerative strategies for cardiac repair. To provide cardiac repair following ischemic injury such as myocardial infarct (MI), three different aspects should be addressed, that is (1) the modulation of the inflammatory response, (2) supporting neoangiogenesis and (3) providing new functional tissue through myocardial regeneration. Stem cell therapy (a) has been extensively investigated, suggesting a regenerative role for stem cells acting either via direct transdifferentiation into cardiovascular lineages or/and through the secretion of cardioactive soluble factors (cytokines, chemokines, etc. represented in the picture in yellow), which can mediate cardiac function improvement, decrease of the infarct size and local activation of the resident endogenous cardiac progenitor cells (CPC, represented here in red, before activation and in green, after stimulation). The beneficial effects achieved with the use of stem cell-conditioned medium and stem cell-derived soluble factors have suggested a new regenerative approach in paracrine therapy (b) based on the direct administration of these secreted mediators. Cardiac tissue engineering (c) has also been proposed as a therapeutic method to provide tissue grafts (valve replacements, blood vessels constructs and myocardial patches) for chronic stages of cardiovascular disease and for cardiac congenital defects by combining multidimensional biomaterials with stem cell culture
Until recently the stem cell regenerative paradigm for cardiac repair was based on the assumption that progenitor cells play a critical role in tissue repair mainly by means of their plasticity and transdifferentiation potential. In this scenario, various stem cells and progenitors have been tested and extensively analysed to assess their potential to generate cardiac lineages in vitro and in vivo. However, recent studies suggest that the mechanism underlying the benefits of stem cell transplantation might be due to paracrine modulatory effects, rather than replacement of affected cells at the site of injury. In the cardiovascular field several studies support this hypothesis, showing successful reduction of infarct size and improvement of cardiac output most likely attributable to the release of soluble pro-survival factors, rather than de novo cardiomyogenesis of the engrafted stem cells [1]. Significant discrepancy on the in vivo cardiac plasticity of transplanted stem cells has also been reported, with their efficacy in terms of survival and engraftment debatable [2]. Recent studies from the extensively investigated mesenchymal stem cells (MSC) to the more recently discovered induced pluripotent stem cells (iPS) have confirmed that the stem cell regenerative potential might be achieved either by generation of new tissue via transdifferentiation or by local release of paracrine factors/chemokines that act on the endogenous cells to improve the general cardiac outcome by mediating neoangiogenesis and attenuating fibrosis and scarring [3–8]. Hence, the quest for the ultimate treatment for myocardial repair via a stem/progenitor cell-based strategy seems to be based not merely on the identification/isolation of the most suitable stem cell candidate, but also on the paracrine-mediated repair via a new approach for cardiac regeneration.
While stem cell and paracrine therapy are meant to provide tissue repair in the acute phase following myocardial ischemic injury, cardiac tissue engineering has been suggested as a therapeutic strategy for the chronic stages of cardiovascular disease and for repair in case of congenital heart defects. Cardiac tissue engineering is based on the combination of multidimensional biomaterials with cultured stem cells in order to create tissue grafts, such as valve replacements, blood vessels constructs and myocardial patches, and it has recently heralded a new promising and exciting horizon in cardiac regeneration. Significant advances have recently been achieved by creating biomaterials and biocompatible matrices/scaffolds which can provide 3D systems to culture and deliver progenitor cells, improving the intercellular crosstalk with the host tissue, thus sustaining their in situ potential for repair and/or regeneration [9–11].
In the broad scheme of stem cells, amniotic fluid is an appealing source with significant potential for cardiac therapy. Amniotic fluid contains multiple cell types derived from the developing foetus and may represent a therapeutic agent which can be easily collected during amniocentesis. In the last few years, several groups have reported the presence of different progenitors in the amniotic fluid, mainly with mesenchymal characteristics [12, 13]. Amniotic fluid stem (AFS) cells expressing stem cell-specific markers such as c-kit, SSEA4 and OCT4 were shown to possess an “immature” phenotype, intermediate in their properties between embryonic and adult stem cells, making them particularly attractive for cellular regeneration. These cells have been demonstrated to be clonogenic, to possess remarkable self-renewal potential while maintaining a stable karyotype and to be pluripotent, giving rise to derivatives of all the three germ layers [14]. Amniotic fluid stem cells can also be cryopreserved and banked for use in future cell-based therapy, due to their peculiar properties, such as survival at lower oxygen tension and their ability to withstand protracted cryopreservation with unaltered self-renewal potential. Their use may also be envisioned to be used for allogeneic therapies, as they have been shown to express similar immunomodulatory properties as adult mesenchymal stem cells [15]. In this chapter we will broadly discuss the most significant findings and the different methods that have been suggested for the use of amniotic fluid stem (AFS) cells for cardiac regeneration.
2 The Stem Cell-Based Therapy
2.1 Stem Cell Transplantation: Let the Right One In
Within cardiac regenerative medicine, stem cell transplantation has been the most widely investigated approach for the treatment of the ischemic myocardium and to prevent/cure heart failure. Consequently, attention has turned to the identification of the most suitable cell source. Many stem cell populations have been proposed and extensively analysed, from the pluripotent embryonic stem (ES) cells to the multipotent adult stem cells [16, 17]. Significant advances in this field have also arisen via the recent derivation of iPS cells by genetic reprogramming of somatic adult cells [18, 19] and with the direct reprogramming of adult cardiac fibroblast into cardiomyocytes [20, 21]. Furthermore, the possibility of deriving multipotent and pluripotent stem cells from foetal tissues, with properties intermediate between embryonic and adult stem cells, has also been described by many groups. Hence, the final aim is to identify the perfect cell candidate that fulfils numerous requirements: the ideal stem source should be safe, neither immunogenic nor tumorigenic; it should improve cardiac function and mediate tissue repair by modulating the inflammation, sustaining the neovascularization process and also generating new cardiomyocytes that functionally couple with host tissue; it should be easily delivered in vivo and be amenable to safe in vitro expansion while, at the same time, circumventing ethical objections. Numerous studies have highlighted the relative merits of specific stem cell types, according to these different criteria.
Foetal tissue represents a potentially viable source of stem progenitors for cell therapy because of its pluripotency, proliferative ability and lack of immunogenicity; such tissue can be obtained from a direct biopsy of the foetus during gestation or from cord blood, term placenta, villi or amniotic fluid [22–25]. Several foetal mesenchymal stem cells with a therapeutic potential for cardiovascular disease have indeed been identified [26–30]; in addition, trafficking of foetal cells to injured maternal myocardium has recently been reported, confirming their homing potential and plasticity once integrated into the infarcted heart [31]. Though these cells have shown a remarkable cardiovascular potential in vitro and capacity to support cardiac repair and regeneration in vivo, their ability to transdifferentiate directly into mature cardiomyocytes has not been definitively demonstrated and it remains debatable [32, 33].
2.2 The Cardiovascular and Cardiomyogenic Potential of AFS Cells
2.2.1 In Vitro Cardiovascular Differentiation of AFS Cells
Amniotic fluid stem cells have been demonstrated to possess remarkable endothelial and smooth muscle plasticity in several in vitro studies. Endothelial differentiation of human c-kit+ AFS and amniotic fluid-derived mesenchymal stem cells with upregulation of CD31 was observed following treatment using EGM-2 differentiating medium, supplemented with recombinant human bFGF, whereas addition of VEGF and hFGF also resulted in the expression of von Willebrand factor (vWf) [13, 14]. More recently, mesenchymal cells isolated from amniotic fluid were demonstrated to acquire endothelial features when stimulated by shear force in addition to growth factors, producing angiogenic mediators such as VEGF and HGF in response to hypoxia [34]. The potential of AFS and AF-derived mesenchymal stem cells towards phenotypic conversion into the smooth muscle lineage has been supported by different studies, both through in vitro co-culture with smooth muscle cells and by applying specific media [35, 36].
Moreover, undifferentiated c-kit+ AFS cells from human and rat demonstrated expression of smooth muscle and endothelial markers, such as smoothelin, angiopoietin1, CD146 and of the “angioblast-endothelial” lineage, like Flk-1 and vWf, together with the antigens of smooth muscle commitment α smooth muscle actin (SMA), smooth muscle 22α and calponin, both at mRNA and protein levels. Their propensity to acquire endothelial and smooth muscle fate was then enhanced by the use of induction culture media [37]. Similarly, c-kit+ GFP+ rat AFS cells were shown to possess smooth muscle and endothelial features, such as in vitro expression of α smooth muscle actin and Flk-1 [38]. These data seem to suggest that AFS cells may contain a subpopulation of cardiovascular progenitors identified by the expression of endothelial and smooth muscle mRNA and proteins in their undifferentiated state; therefore they possess a cardiovascular plasticity in fieri, which needs to be triggered and enhanced by specific culture conditions.
While the acquisition of a vascular phenotype seems to readily occur in the c-kit+ AFS cells and in the AF-derived mesenchymal stem cells, their in vitro induction into the cardiomyocyte lineage has always been more difficult to prove. Although undifferentiated human AFS and AF-mesenchymal stem cells (AF-MSC) showed expression of cardiac transcription factors, such as mef2, GATA4 and Nkx2.5 in their transcriptome, the acquisition of a mature and functional myocyte phenotype seems to need a stimulation more effective than the use of growth factors or induction media [29, 37, 39]. Myocardial differentiation of human and rat AFS cells has been reported via co-culture with neonatal rat cardiomyocytes, with cardiomyocyte sarcomeric features of 3.5–5 % of treated AFS cells after a few days, increasing to almost 16 % after 9 days [37, 38]. Along with the expression of structural cardiac proteins, such as troponin I and T and sarcomeric α-actinin with myofibrillar organization, c-kit+ rat AFS cells also showed functional acquisition of a more mature phenotype, by expression of synchronous contractile activity and electrical excitability, with detection of action potentials and pace-making activity similar to the surrounding neonatal cardiomyocytes [38]; moreover, other independent studies using AFS cells (with or without c-kit sorting) supported the co-culture method as a tool to induce these stem cells to acquire a cardiogenic fate and develop functional gap junctions, with expression of connexin 43 and N-cadherin proteins between the two cell types [40]. Interestingly, these results were obtained only when AFS cells were in direct contact with the neonatal cardiomyocytes, with no evidence of transdifferentiation in co-culture experiments either using inserts to separate the two cell sources or when using conditioned media [38]. Although the contribution of a fusion process cannot be ruled out, these results seem to suggest that specific features of the neonatal cardiomyocytes, such as direct physical contact or short-range chemical signals, may induce the AFS cells to acquire a cardiogenic phenotype while in co-cultures.
Cardiac differentiation of AFS cells has also been reported by treatment with the demethylating agent 5-aza-2´-deoxycytidine, though despite an initial upregulation of cardiac structural markers in the treated cells, no mature structural and functional phenotype was observed in the long term [39].
A comparison of the cardiomyogenic properties of c-kit+ AFS cells versus the c-kit− counterpart has also been reported, showing that the myocardial differentiation capacity was enhanced in the c-kit+ population, with detection of GATA-4, cTnT, α-actin, connexin 43 mRNA and proteins after myocardial induction, compared to the c-kit− counterpart in which only GATA-4 mRNA and protein were detected [41]. More recently, first trimester human AFS cells were demonstrated to be reprogrammed to complete pluripotency without use of ectopic factors or viral vectors, but only via specific culture conditions, such as medium supplemented with valproic acid, a histone deacetylase inhibitor. This treatment resulted in the formation of embryoid bodies in vitro with rhythmically contracting activity, suggesting transition to a primitive cardiogenic phenotype [42]. Despite all the encouraging results achieved with the techniques mentioned here, some important considerations remain to be addressed, such as the direct transdifferentiation potential of the AFS cells versus cell fusion and the efficiency of the cardiovascular differentiation acquired in vitro, which needs to be tested for the long term, in order to assess the stability and the safety of the phenotypic conversion.
2.2.2 In Vivo Cardiogenic Potential of AFS Cells
In recent years different studies have been proposed to evaluate the therapeutic potential of AFS cells as an allograftable stem cell source for in vivo cellular cardiomyoplasty. Transplantation in preclinical animal models of myocardial infarction (MI) has been broadly analysed in order to validate the cardiomyogenic and cardiovascular potential of the AFS cells.
Rat mesenchymal stem cells isolated from amniotic fluid were compared to bone marrow-derived stem cells (BM-MSC) for cellular cardioplasty by intramyocardial injection in the acute necrotizing ischemic area of syngeneic and athymic rat hearts in a preclinical model of cardiac cryoinjury. AF-MSC showed lower engraftment than BM-MSC in the short term, but after 30 days both cell types were detected in similar numbers in the host tissue. AF-MSC were shown to possess a greater proliferative potential in the long term. About 34.6 % of the injected BM-MSC and 49.6 % of the transplanted AF-MSC acquired a cardiovascular phenotype in vivo with expression of cardiac troponin T, vWF or α smooth muscle actin. Notably, while BM-MSC possess a broad cardiovascular potential, giving rise to cells of myocardial, smooth muscle and endothelial lineages, forming both capillaries and small arterioles, whereas AF-MSC demonstrated a more restricted myocardial and endothelial fate in vivo [36].
Human c-kit+ AFS cells xenotransplantated into a rat model of myocardial infarction via intramyocardial injection (with or without cyclosporine treatment as well as in nude rats) were found in the host tissue as traces of the original cell inoculum after 15 and 30 days. Some AFS cells expressing both the human-specific anti-platelet nonmuscle myosin (hptMyosin) and cardiovascular antigens, such as cardiac troponin T, were found as tissue/cell fragments in the transplanted heart. These hptMyosin+ AFS cells still expressed the stem cell marker SSEA4 suggesting an incomplete acquisition of a cardiogenic phenotype, while easily forming smooth muscle and endothelial cells, as confirmed by expression of α smooth muscle actin or vWf [37]. Despite the negative expression of HLA-DR antigens in the human c-kit+ AFS cells, all the animal recipients in this study—immunocompetent, immunodeficient or immunosuppressed—showed a significant infiltration of inflammatory cells, indicating that these cells struggled to survive and engraft, due to the host xeno-immune response. In a more recent study, human AFS cells were analysed for their therapeutic potential for cardiac repair in a similar immune-suppressed rat model with MI, via intramyocardial injection into peri-infarct areas. After 4 weeks the treated animals showed attenuation of the left ventricle remodelling with higher vascular density and an overall improvement in cardiac function, when compared with the saline injected control group. Furthermore the engrafted human AFS cells showed expression of cardiac marker such as Nkx2.5, α-actinin, cardiac troponin T together with connexin 43, suggesting structural coupling with the host tissue and supporting their therapeutic potential for cellular cardiomyoplasty [40]. The in vivo potential of rat GFP+ ckit+ AFS cells for myocardial repair was further evaluated by transplantation in the heart of animals subjected to cardiac ischemia/reperfusion injury and monitored by magnetic resonance imaging (MRI), following the labelling of cells with a super paramagnetic iron oxide particles solution. Three weeks after transplantation a small proportion of rat AFS cells acquired a cardiovascular phenotype with expression of endothelial and smooth muscle antigens and, to a lesser extent, cardiomyocyte markers, including cardiac troponin I. Despite the low number of rat GFP+ ckit+ AFS cells engrafted in the heart, there was still an improvement of the ejection fraction as measured by MRI, with a trend towards physiological values [38]. In light of these results, AFS cells have been shown to possess a remarkable propensity to acquire a cardiomyogenic phenotype in vitro while preserving cardiac function and differentiating into cardiovascular lineages in vivo, although their potential seems to be limited by the poor survival and low engraftment in an allogeneic setting in the long term.
To overcome one of the major limitations in cardiac cell therapy, i.e. the significant loss of transplanted cells and their low long-term engraftment and survival, alternative approaches to the injection of a single cell suspension have also been tested. Injection of spheric cell bodies obtained with AFS cells and extracellular matrix structures has been shown to enhance cell retention and functional benefits, following myocardial infarction in a preclinical rat model; bioluminescence imaging, real-time polymerase chain reaction (PCR), echocardiography and MRI revealed that the human AFS cells “bodies” remarkably enhanced cell retention and engraftment, both in the short- and in the long-term, limiting the progression of heart failure and improving cardiac function. Furthermore, expression of HGF, bFGF and VEGF were upregulated in the ischemic area, along with direct differentiation of the transplanted human cellular bodies into angiogenic and cardiomyogenic lineages [43]. The aforementioned results demonstrate that enriching the human AFS cells injectable preparation with extracellular matrix components can support their in vivo retention, improving functional engraftment with host tissue.
Despite these encouraging preclinical results, further validation of the in vivo cardiogenic potential of the AFS cells for cellular cardiomyoplasty is still required. Indeed, animal models present several limitations and clinical trials in highly selected patients are required to fully test their therapeutic applicability. Furthermore, a better understanding of the immunomodulatory and immunogenic properties of AFS cells, along with detailed mechanisms of their cardiovascular regenerative potential, is required to translate these preliminary results into the clinical arena.
3 Moving Forward from Stem Cell-Based Therapy into Stem Cell Factor-Based Therapy: The Paracrine Hypothesis
Initially the regenerative paradigm in stem cell therapy was based on the assumption that progenitors could play a critical role in cardiac repair by means of their plasticity in giving rise to new cardiovascular cells. The results of a large number of studies have revealed that the mechanism underlying the benefit achieved by transplanting stem/progenitor cells in the heart appears to relate primarily to their paracrine modulatory effect, rather than direct replacement of lost cells through direct transdifferentiation. Indeed considering the limited long-term engraftment of the transplanted cells, together with their general low transdifferentiation propensity, while significantly improving the cardiac function, it is indeed more plausible that stem cell-derived regenerative effects may act through local release of soluble factors and their effect on host cells, rather than from differentiation of exogenous transplanted cells. Considering the stem cell populations mostly investigated thus far, such as bone marrow-derived stem cells, several studies report a temporal improvement in cardiac function and neovascularization with a decrease in infarct size, following transplantation. Since permanent engraftment and direct cardiac transdifferentiation of the injected stem cells have been refuted, current hypotheses to explain their beneficial effect include their ability to alter the local microenvironment by secreting bioactive trophic factors, acting to reduce cardiomyocyte apoptosis and ventricular remodelling and support neovascularization, thereby stimulating cardiac regeneration [5]. Thus, mounting evidence that stem cell-mediated repair relies more on the paracrine stimulation, rather than their plasticity, has led to a paradigm change in regenerative medicine, shifting the attention from the stem cell genome to the stem cell “secretome”, represented by the modulatory cytokines, chemokines and growth factors that they can release to influence the surrounding tissue. MSC have been demonstrated to secrete a wide array of cytokines and soluble factors involved in cardioprotection, neoangiogenesis and cardiac regeneration, such as FGF, bFGF, VEGF, TGFβ, IGF-1 and SDF-1 [1]. Moreover, the administration of stem cell-conditioned medium was shown to reduce apoptosis and improve cardioprotection in preclinical experimental models of myocardial infarction, reproducing beneficial effects of cell transplantation [44]. In light of these results, there has been a shift in emphasis from cell-based therapy to protein-based therapy, establishing a new therapeutic approach based on modifying stem cells, inducing them to express higher levels of specific paracrine factors. Impressive results have been reported with stem cells over-expressing pro-survival agents, such as bone marrow-derived MSC over-expressing Akt or HIF-1α, which led to reduced apoptosis and ventricular remodelling and restoration of cardiac function in vivo [1, 45].
Another mechanism accounting for the improved outcome of the injured myocardium, following stem cell transplantation, has been suggested in the synergistic crosstalk between the injected exogenous stem cells and the endogenous resident cardiac progenitor cells (CPC). Indeed, the exogenous transplanted cells may provide crucial factors via paracrine signalling that can boost the in situ activation, proliferation and mobilization of the endogenous CPC, as represented in Fig. 1.1 [46]. This “teamwork” hypothesis seems to be confirmed now by several studies. Transplantation of MSC into infarcted hearts led to the formation of cardiomyocytes co-expressing the CPC marker c-kit in a pig model, whereas endothelial progenitor cells triggered proliferation of c-kit+ CPC via secretion of VEGF, IGF-1 and SDF-1 [47, 48]. More recently, an elegant study analysed the effect of bone marrow-derived stem cell therapy on the cardiac endogenous regenerative response after myocardial infarct, confirming that c-kit+ bone marrow-derived stem cells activated endogenous cardiac progenitors, resulting in their differentiation into cardiomyocytes and a general improvement of ventricular function. This effect was suggested to be dependent on paracrine communication between the exogenous transplanted cells and the host cells [49]. Hence identifying the detailed mechanism and the key soluble factor(s) through which the transplanted stem cells “communicate” and support/recruit resident CPC for cardiac repair may facilitate progress in regenerative medicine, establishing the basis for the development of protein-based therapies and refining the current state of stem cell-based strategies.
3.1 A Role for the AFS Cell Secretome
Growing interest has been recently reported towards the analysis of the AFS cell secretome, as a source of therapeutic paracrine factors responsible for the strong angiogenic effect and regenerative properties that these cells exert in vivo. The in vitro analysis of human c-kit+ AFS cell-conditioned medium revealed the presence of cytoprotective, pro-differentiative and chemoattractive soluble mediators, such as MCP-1, IL-8, SDF-1 and VEGF, which can provide in vivo tissue regeneration in a mouse preclinical model of hind-limb ischemic injury, both by recruiting the host endothelial cells and supporting neo-arteriogenesis and also via the remodelling of pre-existing collateral arteries [50]. Similarly, the regenerative potential of the AFS cells secretome was tested using a rat model of ischemic full-thickness skin flap in the epigastric region with topical delivery of the c-kit+ AFS cell-conditioned medium, embedded in a gelatin membrane. After 7 days of treatment, the ischemic area showed a better histological profile, together with improved reperfusion and repopulation of the area by host-derived endothelial precursors, confirming the beneficial therapeutic effect of the AFS cell-derived chemokines and growth factors on the ischemic lesion [51]. Furthermore, spindle-shaped amniotic fluid-derived mesenchymal stem cells were also shown to enhance new vessel formation both in vitro and in vivo, via the modulation of migration, proliferation and vascular tubule formation of human umbilical cord blood endothelial colony forming cell, due to their paracrine potential in secreting soluble factors such as IL-8, PDGF-AB/BB and MMP9. Notably these cells also supported increased angiogenesis/vasculogenesis in an NOD-SCID murine model more effectively than bone marrow-derived stromal cells [52].
The in vivo regenerative effect of human c-kit+ AFS cells for cardiac repair has been recently evaluated in a rat model of acute myocardial infarction by ischemia/reperfusion injury, to assess whether their therapeutic potential may be mediated by secretion of critical paracrine factors. In this study, systemic administration of AFS cells during the acute phase of reperfusion provided a remarkable decrease of 14 % in the infarct size, compared to control animals, together with a reduced number of apoptotic cardiomyocytes. Thus, these data confirmed the hypothesis of the cardioprotective role of the ckit+ AFS cells in vivo [53]. Considering the reduced amount of human c-kit+ AFS cells engrafted in the cardiac tissue over the limited time of the study (2 h) and the rapidity of the therapeutic effect exerted on the host, the authors suggested that the results obtained were achieved via a local paracrine effect. The human c-kit+ AFS cell-conditioned medium was then analysed, revealing a significant enrichment for thymosin beta 4 (Tβ4), a paracrine key factor for coronary angiogenesis during development, as well as a remarkable stimulator of cardiomyocyte survival and epicardial progenitor cells reactivation and cardiac differentiation following injury, as recently demonstrated [54–58]. The cardioprotective paracrine potential of human AFS cells was subsequently confirmed by repeating the set of experiments using the cell-conditioned medium and showing similar results, in terms of reducing the necrotic infarcted area and supporting the cardiomyocyte survival within the reperfusion time. These results suggested that, in the setting of acute myocardial infarction c-kit+ AFS cells possess a significant cytoprotective regenerative potential by paracrine effect with a potential therapeutic candidate in the small peptide Tβ4, which can activate the resident endogenous CPC to provide cardiac repair.
In light of these results, AFS cells may represent an appealing source for cardiac repair with an indirect regenerative role through a paracrine mechanism of action, rather than by direct cardiomyogenic transdifferentiation. Hence, a new stem cell-based strategy for cardiac regeneration may be based on culturing stem cells, such as AFS cells, to isolate therapeutic bioactive molecules produced in their secretome. These regenerative factors could then be administrated to the injured myocardium, in order to exert a paracrine modulation of the inflammation process, activation of cardiomyocyte pro-survival pathways while supporting neo-angiogenesis and stimulating the activation of the endogenous CPC to influence cardiac repair and regeneration in the acute phase following an injury.
Despite the significant effort that has been invested in stem cell-based therapy for cardiovascular regeneration and repair, much scepticism still surrounds cell transplantation methods, due to limitations associated with the safety, immunogenicity and limited efficacy of the transplanted cells, therefore paracrine therapy is envisioned to be a more straightforward translational approach and the isolation and administration of specific stem cell-derived regenerative factors for protein therapy may represent an extremely promising strategy. This approach offers a more clinically feasible mechanism to obtain stem cell-mediated regenerative effects in vivo, without the inherent complications associated with stem cell delivery. If translated successfully, curing cardiovascular diseases and congenital defects by administrating a cocktail of proteins in the form of a pill or of an injection may soon become a clinical reality.
Furthermore, paracrine therapy offers specific advantages for the clinical read out. Cardiovascular disease patients need prompt therapeutic intervention. Therefore it would be ideal to have access to “off-the-shelf” products for simple administration. Stem cells can be utilized as “drug-stores” to produce specific soluble factors, which can ultimately be administered orally as a pill or systemically as a suspension. This strategy could replace the invasive or additional surgical procedures generally required by cell therapy or tissue engineering methods.
In this scenario the ideal stem cell source should be selected upon consideration of their paracrine potential and the feasibility of their isolation together with their in vitro self-renewal properties. AFS cells unambiguously fulfil both criteria, as previous results showed that they possess an appealing cardiomyogenic and cardiovascular potential, being at the same time an attractive reservoir of regenerative factors with angiogenic and cardioprotective effects. These features make them an attractive source of paracrine factors for protein therapy.
4 Mending a Broken Heart: The Tissue Engineering Approach
Along with stem cell-based therapy approaches, another promising therapeutic strategy that has rapidly evolved is represented by cardiac tissue engineering. Tissue engineering represents “the restoration of function through the delivery of living elements which becomes integrated into the patient”, as first reported by Vacanti in 1999 [59] and it has developed with the aim of restore and restoring and improving the diseased or damaged heart, through the application of a living graft, cardiac patch, vascular and valve constructs. New cardiac tissue engineering approaches combine the application of nanotechnology and chemical engineering with medicine, using smart biocompatible polymeric biomaterials and a broad range of cell types, including stem or stem cell-derived cardiac cells [11, 60, 61]. The main function of the biomaterial is to mimic the biological function and mechanical support of the extracellular matrix providing mechanical stimuli and an instructive scaffold for both the in vitro and/or in vivo stem cell growth, differentiation and maturation and/or a way for their delivery into the host tissue improving retention, engraftment and function. Compared to the traditional approach based on stem cell therapy, cardiac tissue engineering offers some important advantages, overcoming particular problems related with cardiac cell-based therapy and stem cell transplantation. Combining the use of bioactive matrices with stem cell biology provides a better cardiac-instructive microenvironment, via inter-cell and cell–matrix interactions, to support the in vitro and in vivo stem cells maturation, therefore improving their differentiation potential and the secretion of paracrine and trophic factors involved in cardiac regeneration [9, 10, 62]. Different biomaterials have been studied and engineered in several diverse modalities, attempting to faithfully reproduce the morphological and physiological properties of the heart. Scaffolds may be natural biomaterials, derived from matrix proteins (e.g., collagen or alginate, or synthetic polymer substances such as poly-lactic acid or poly-lactic glycolic acid), that can be produced in form of meshes, fibres, porous, solids or hydrogels [63] or, alternatively, may be the extracellular matrix produced by the cells themselves, injected as “sheets” or “patches” (also known as “scaffold-free” approach [64]. Acellular matrices, obtained by removing cellular components from specific tissues have been also used to produce in vitro functional bioartificial cardiac tissue; for example, by reseeding an acellular, perfusable vascular architecture of the mouse heart with endothelial and cardiomyocyte cells [65] or as injectable scaffold with the capacity to form a nanofibrous structure in vivo and to increase arteriole formation post-injection [66]. A more novel method has recently been developed based on innovative formulations like hydrogels used to entrap cells to improve their delivery and engraftment in the host tissue. In addition to the necessary requirements (biocompatible, biodegradable and biomimetic), hydrogels have also been shown to offer an improved alternative, as they represent a versatile biomaterial that can be injected in vivo without distorting the heart geometry and acting as a cell-carrier, delivering them directly into the infarcted wall to increase their survival, engraftment and functional integration [67, 68]. Hydrogels also provide the encapsulated stem cells and the necessary extracellular matrix signals so as to enhance their survival following engraftment. Promising results have also been achieved by establishing a tissue engineering platform for the delivery of human mesenchymal progenitor cells based on a composite matrix, obtained by applying fibrin hydrogel onto decellularized sheets of human myocardium. Once implanted into infarcted nude rat hearts, the transplanted human cells migrated to the ischemic area and supported a paracrine-mediated neovascularization response, resulting in the recovery of baseline levels of ventricular dimensions and contractility [69].
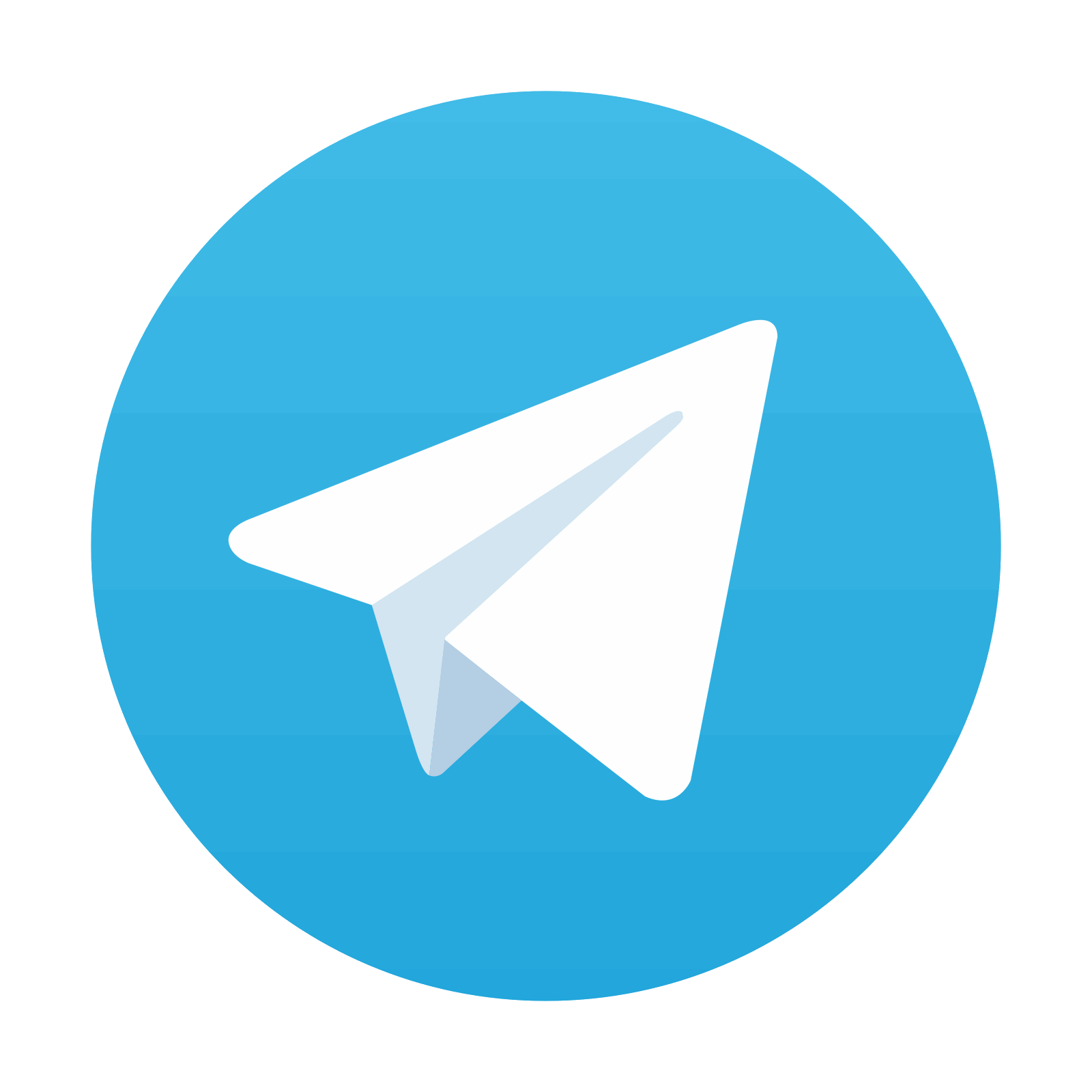
Stay updated, free articles. Join our Telegram channel

Full access? Get Clinical Tree
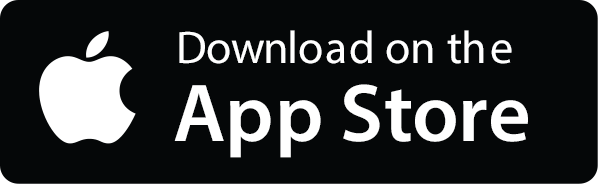
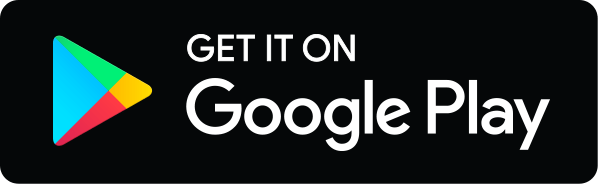