Fig. 2.1
Histotroph in the uterine lumen represents secretions from the uterine endometrial luminal (LE) and glandular (GE) epithelia, as well as molecules that are selectively transported into the uterine lumen. Histotroph includes the various classes of proteins listed in this figure, as well as nutrients such as glucose and amino acids . Amino acids, the subject of this review, are transported into the uterine lumen and then from the uterine lumen into the conceptus (embryo and its associated extra-embryonic membranes) to stimulate growth and development. Receptors for progesterone (PGR) are down-regulated in uterine LE and GE due to autoregulation by progesterone and interferon tau (IFNT) silences expression of receptors for estradiol (ESR1) and oxytocin (OXTR) to prevent oxytocin-mediated pulsatile release of prostaglandin F2a (PGF) which prevents regression of the corpus luteum. The endometrial stromal fibroblasts express PGR and secrete progestamedins, particularly fibroblast growth factor 10 and, to a lesser extent, hepatocyte growth factor (HGF) that regulates uterine epithelia cell functions. Interferon tau induces expression of interferon regulatory factor 2 (IRF2) in uterine LE and superficial GE specifically which prevents those cells from expressing classical interferon stimulated genes (ISG). This allows IFNT and progesterone, acting via progestamedins from uterine stromal cells, to stimulate expression of a unique set of genes in uterine LE and superficial GE that are in direct contact with the trophectoderm, such as those for transport of nutrients like amino acids and glucose. Those nutrients then support growth and development of the conceptus (see Bazer et al. 2009a, b, 2010; Bazer 2013)
The majority of embryonic mortality in ewes occurs before Day 18 of gestation (Nancarrow 1994). Key physiological events during early pregnancy include maternal recognition of pregnancy signaling via interferon tau (IFNT), formation of conceptus mesoderm, elongation of conceptus trophectoderm, formation of trophectoderm binucleate cells, attachment of trophectoderm to uterine LE/sGE, and secretion or selective transport of components of histotroph into the uterine lumen (Guillomot et al. 1993; Bazer et al. 2013). Elongation of ovine conceptuses is a prerequisite for central implantation and synepitheliochorial placentation which is superficial and noninvasive with increasing apposition and then adhesion between trophectoderm and uterine LE/sGE (Bazer 2013). In several prolific breeds of ewes, increases in litter size adversely affect fetal growth and lamb survival even though ewes are maintained under intensive management conditions (see Gootwine et al. 2008; Gootwine 2013). Prenatal fetal death associated with intrauterine fetal growth restriction (IUGR) and difficulties during lambing are primary causes of lamb mortality (Gootwine et al. 2007).
Embryonic mortality and the pattern of development of pig conceptuses are similar to those for sheep conceptuses (Bazer and First 1983; Geisert et al. 1982a; Geisert et al. 1982b). Spherical pig blastocysts (0.5–1 mm diameter) shed the zona pellucida between Days 6 and 7, expand to 2–6 mm diameter on Day 10, then elongate rapidly to a filamentous form by Day 16. Bovine blastocysts are spherical on Days 8–9 (.17 mm diameter), oblong or tubular by Days 12–13 (1.5–3.3 mm by 9–1.7 mm) and then filamentous between Days 13–14 (1.5 × 10 mm), 14–15 (2 × 18 ram), 16–17 (1.8 × 50 mm) and 17–18 (1.5 × 160 mm). By Days 17–18 the bovine blastocyst occupies about two-thirds of the gravid uterine horn. It then occupies the whole gravid uterine horn by Days 18–20 and extends well into the contralateral uterine horn by d 24 (Chang 1952). Sheep blastocysts are basically spherical between Days 4 (.14 mm diameter) and 10 (0.4 mm diameter) and then elongate to the filamentous form by Day 12 (1.0 × 33 mm) and 14(1.0 × 68 mm) and Day 15 (150–190 mm long x 1 mm diameter) before the trophoblast extends through the uterine body and into the contralateral uterine horn by Days 16–17 (Chang and Rowson 1965; Bindon 1971).
The dramatic changes in morphology of pig, cow and sheep conceptuses precede initial attachment of trophectoderm to uterine LE and initiation of a non-invasive “central-type” implantation (Steven 1975). It is during this period of morphological and functional transition that 30–40 % of the conceptuses die, with many failing to elongate and/or achieve extensive contact of trophectoderm with uterine LE for uptake of components of histotroph from the uterine lumen. Among the species of livestock, prolific pigs and ewes suffer the greatest prenatal losses due to a suboptimal intra-uterine environment which may include inadequate uterine secretions and sub-optimal nutrition (Vonnahme et al. 2002; Bazer et al. 2009b). The first peak of embryonic deaths occurs between Days 12 and 15 of gestation and three-fourths of prenatal losses occur in the first 25 or 30 days of gestation (Bazer and First 1983). Then, fetal losses occur between Days 30 and 75 of gestation likely as a result of inadequate uterine capacity for placentation that is primarily at the expense of those conceptuses that experience insufficient elongation of the trophectoderm during the peri-implantation period of pregnancy (Bazer et al. 1969a, b; Fenton et al. 1970; Webel and Dziuk 1974).
All mammalian uteri contain endometrial glands that produce/or selectively transport a complex array of proteins and related substances in histotroph (Bazer 2013). Among the nutrients in histotroph, amino acids play the most important roles in growth and development of the conceptus because they are essential for protein synthesis and activation of cellular functions (Kim et al. 2008; Wu et al. 2013b). This places amino acids at the forefront of animal health because fetal growth restriction has permanent negative impacts on neonatal adjustment to extra-uterine life, preweaning survival, postnatal growth, feed utilization efficiency, lifetime health, tissue composition (including protein, fat, and minerals), meat quality, reproductive function, and athletic performance (Wu et al. 2006). Based on dietary needs for nitrogen balance or growth, amino acids have been traditionally classified as nutritionally essential (indispensable) or nonessential (dispensable). Essential amino acids (EAA) are those for which carbon skeletons cannot be synthesized or those which are inadequately synthesized de novo by the body to meet metabolic needs and must be provided in the diet to meet requirements (Wu et al. 2013c). Nonessential amino acids (NEAA) are defined as those amino acids which are synthesized de novo in adequate amounts by the body to meet requirements. Conditionally essential amino acids are normally synthesized in adequate amounts by the organism, but must be provided in the diet to meet needs under conditions where rates of utilization are greater than rates of synthesis. Functional amino acids are defined as those that not only serve as building blocks of protein but also regulate key metabolic pathways to benefit health, survival, growth, development, and reproduction of animals and humans (Wu 2010a). These unique nutrients include arginine (Arg), cysteine (Cys), glutamine (Gln), leucine (Leu), proline (Pro) and tryptophan (Trp). Thus, functional amino acids can be as either EAA, NEAA, or conditionally essential amino acids (Li et al. 2009; Tan et al. 2009; Wu 2013b).
Nitric oxide (NO) and polyamines (putrescine, spermidine, and spermine) are products of Arg catabolism that are critical for placental growth (Wu et al. 2009). Arginine stimulates placental NO production by enhancing expression of GTP cyclohydrolase I (GCH1), the first and rate-controlling enzyme for synthesis of tetrahydrobiopterin (BH4, an essential cofactor for all isoforms of NO synthase). Additionally, glutathione, synthesized from glutamate, glycine and cysteine, is the major antioxidant in the conceptus (Wu 2009). Transport of amino acids requires multiple specific transporters (Grillo et al. 2008). Of particular note, intra-uterine growth restriction (IUGR) of fetuses is associated with impaired transport of basic, neutral and acidic amino acids by the placenta (Regnault et al. 2005; Wu et al. 2008). Thus, maternal protein nutrition greatly impacts embryonic/fetal survival in pigs (Pond et al. 1969; Pond et al. 1981). Along with insulin-like growth factors , vascular endothelial growth factors and other growth factors, NO and polyamines are crucial for angiogenesis, embryogenesis, placental growth, utero-placental blood flows, and transfer of nutrients from mother to fetuses, as well as fetal-placental growth and development (Wu et al. 2006; Wu and Meininger 2009).
We discovered an abundance of Arg in porcine allantoic fluid during early gestation (Wu et al. 1995, 1996a). Arginine and ornithine account for 50 and 55 % of the total alpha amino-acid nitrogen (the sum of nitrogen in α-amino acids) in porcine allantoic fluid on Days 40 and 45 of gestation, respectively. Similarly, members of the Arg family of amino acids are highly abundant in ovine allantoic fluid (e.g., 10 mM citrulline and 25 mM Gln on Day 60 of gestation) (Kwon et al. 2003). The ovine placenta expresses arginase; therefore, citrulline is abundant in allantoic fluid whereas the pig placenta does not express arginase, which allows for accumulation of arginine in allantoic fluid. These observations suggested important biological roles for Arg in growth and development of mammalian conceptuses. Accordingly, rates of NO and polyamine synthesis in both porcine and ovine placentae are highest during early gestation when placental growth is most rapid (Kwon et al. 2004a, b; Wu et al. 2005, 2012). We hypothesize that impaired placental growth (including vascular growth) or function results from reduced placental synthesis of NO and polyamines, thereby contributing to IUGR in both underfed and overfed dams (Wu et al. 2004b). Growing evidence from studies with pigs, sheep, and rats supports this hypothesis (Wu et al. 2013a, b, c, d).
2.2 Leucine, Arginine and Glutamine
Leucine, Arg and Gln are of particular interest based on their roles in conceptus development. In mice, outgrowth of trophectoderm requires Leu or Arg for expanded blastocysts to exhibit motility and outgrowth of trophectoderm essential for implantation (Gwatkin 1966, 1969; Martin and Sutherland 2001; Martin et al. 2003). Leucine and Arg initiate cell signaling via a serine-threonine kinase and MTOR to regulate protein synthesis and catabolism, and induce expression of genes for insulin-like growth factor 2 (IGF2), nitric oxide synthases (NOS) and ornithine decarboxylase (ODC1) (Nielsen et al. 1995; Kimball et al. 1999; Murakami et al. 2004). This may allow the conceptus and uterus to coordinate differentiation of trophectoderm with development of uterine epithelia receptive to implantation. There are also differential effects of Leu, Arg and Gln on hypertrophy and hyperplasia of cells important for conceptus development during the peri-implantation period of pregnancy (Kim et al. 2011b). Physiological levels of Leu, Arg and Gln stimulate activities of MTOR and ribosomal protein S6 (RPS6) kinase, and proliferation of trophectoderm cells (Kim et al. 2013). Interestingly, the actions of Gln require the presence of physiological concentrations of glucose or fructose (a precursor of fructose-6-phosphate and thus glucosamine-6-phosphate), supporting the view that hexosamine plays a cell signaling role in conceptus growth and development (Kim et al. 2012). Cellular events associated with elongation of ovine and porcine conceptuses during the peri-implantation period of pregnancy involve both cellular hyperplasia and hypertrophy, as well as cytoskeletal reorganization during the transition of spherical blastocysts to tubular and filamentous conceptuses (Albertini et al. 1987; Mattson et al. 1990; Burghardt et al. 2009).
The human placenta transports amino acids to the fetal circulation against the concentration gradient utilizing both sodium dependent and sodium independent transporters based on published research focused on System A amino acid transporters that primarily transport small and neutral amino acids (Dilworth and Sibley 2013). A range of transporters have been found to be affected in pregnancies with intra-uterine growth restriction as there is an inverse relationship between placental size and System A amino acid transporters (Desforges and Sibley 2010) and the level of activity of System A amino acid transporters is related to the severity of the intra-uterine growth restriction. These results are consistent with a current model suggesting that amino acid transporters are critical components of the nutrient sensing system and that amino acids stimulate MTORC1 which in turn enhances expression of transporters linking maternal nutrient availability and fetal growth (Jansson et al. 2012).
2.3 MechanisticTarget of Rapamycin (MTOR)
Mouse and human blastocysts express at least 14 amino acid transporters, but the Leu-selective system B0,+ controls uptake of Leu and Arg that induce MTOR signaling critical to conceptus development (Van Winkle and Campione 1987). Regulation of B0,+ in mice involves estrogen-induced increases in Na+ in uterine secretions to stimulate Na+-dependent Leu uptake and there is evidence for stimulation of system B0,+ by proteases such as chymotrypsin-like enzymes. FRAP1 (FK506 binding protein 12-rapamycin associated protein 1, also known as MTOR) is a highly conserved serine threonine protein kinase which senses and responds to changes in abundance of amino acids and energy, as well as hormones and mitogens to effect changes in metabolism and growth of cells (Dennis et al. 1996; Gingras et al. 2001; Liao et al. 2008; Wullschleger et al. 2006). The MTOR cell signaling pathway is a “nutrient sensing system” of protein kinases that effect translational events through phosphorylation and activation of ribosomal protein S6 (RPS6). Phosphorylation by MTOR of RPS6 increases translation of 5ʼTOP mRNAs that encode proteins important for translational events, including phosphorylation of eukaryotic initiation factor 4E (eIF4E) binding protein 1 that is a translational repressor via a yet unknown mechanism. The role of eIF4E in regulating differentiation of trophectoderm involves Ras transformation and, when over-expressed, leads to malignant transformation (Kimball et al. 1999; Fingar et al. 2004).
In Xenopus embryos Ras promotes mesoderm induction (Klein and Melton 1994). Thus, molecules that stimulate MTOR activity in trophectoderm likely stimulate, as described in the preceding paragraph, translationof mRNAs critical to conceptus development, including IGF2, ODC1 and NOS (Gwatkin 1966; Kaliman et al. 1999; Van Winkle and Campione 1983; Murakami et al. 2004). IGF2 expressed by tropectoderm of mice during implantation induces NO production, whereas ODC1 is the rate-limiting enzyme in the synthesis of polyamines from ornithine (a product of arginase action on Arg) which are required for DNA and protein synthesis and, therefore, conceptus development and differentiation. Homozygous mutant mice lacking MTOR die shortly after implantation due to impaired cell proliferation and hypertrophy in both the embryonic disk and trophoblast (Murakami et al. 2004). Further, deletion of the C-terminal six amino acids of MTOR which are essential for its kinase activity also inhibits hyperplasia and hypertrophy of cells of mouse blastocysts (Murakami et al. 2004).
2.4 Nitric Oxide and Trophoblast Motility
Nitric oxide generated from conversion of Arg to NO by eNOS and/or iNOS in trophoblast cells activates guanyl cyclase to produce cGMP, stimulates trophectoderm motility perhaps by modifying the extra-cellular matrix (ECM), induces vasodilation of maternal blood vessels (Guo et al. 2005), and regulates cellular energy metabolism (Dai et al. 2013). During ovine conceptus elongation and implantation, there is a significant increase in expression of SPP1 (Johnson et al. 2003) by uterine GE, and NO induces SPP1 expression that increases cell adhesion and invasion in cultured cells (Saxena et al. 2000; Cartwright et al. 2002). In addition, hepatocytegrowth factor (HGF)-induced motility of human trophoblast cells is activated by NO signaling through phosphatidylinositol bisphosphate-3 kinase (PI3K), serine/threonine kinase (AKT) and MTOR (Kwon et al. 2004a). Expression of iNOS is highest in peri-implantation mouse blastocysts (Reynolds et al. 2005). There are increases in eNOS and iNOS activities in ovine placentomes between Days 30 and 60 of gestation that are sustained to Day 140 of gestation and increases in placental NO synthesis parallel increases in placental vascular growth and utero-placental blood flows in ewes during pregnancy (Reynolds et al. 2005).
2.5 Polyamines and Trophoblast Motility
Changes in motility of trophectoderm cells may result from increases in expression of ODC1, the rate-limiting enzyme in polyamine synthesis from Arg, proline and ornithine (Mehrotra et al. 1998). Polyamines associate with DNA and nuclear proteins to produce normal chromatinrequired for gene transcription , proliferation of trophectoderm and formation of multinucleated trophectoderm cells that give rise to giant cells in the placentae of mice (Kwon et al. 2004a). Polyamine cell signaling pathways include tyrosine and mitogen activated protein kinases (MAPK) and proto-oncogenes, c-myc, c-jun, and c-fos (Kwon et al. 2004b). Polyamines also activate MTOR cell signaling to stimulate protein synthesis in porcine trophectoderm cells (Kong XF, Bazer FW, and Wu G, unpublished data). ODC1 is important for motility, integrin signaling via focal adhesion kinases, cytoskeletal organization, and invasiveness of mouse blastocysts . Additionally, polyamines stimulate trophectoderm cell motility through modification of beta-catenin phosphorylation, as well as changes in uterine epithelial cells that allow blastocysts to adhere to uterine LE and undergo superficial implantation (Martin et al. 2003).
Synthesis of polyamines is highest in ovine placentomes and endometrium between Days 30 and 60 of gestation when their growth and morphological changes are most rapid, and high levels of polyamines in ovine placental and endometrial tissues in the second half of pregnancy likely contribute to continued development of the placental vascular bed for increased uterine blood flow to support fetal growth (Kwon et al. 2004b). Similar results have been reported for porcine conceptuses (Wu et al. 2005). Knockout of the Odc1 gene in mice is not lethal until the gastrulation stage of mouse embryogenesis (Pendeville et al. 2001). There is a requirement for polyamines later in embryogenesis as Odc1 null embryos at the late morula to early blastocyst stages do not survive in vitro due to apoptotic cell loss in the inner cell mass, but this condition can be rescued by providing putrescine (a precursor of spermidine and spermine) in drinking water of the dam up to the early implantation stage, but not beyond that stage of pregnancy (Pendeville et al. 2001).
2.6 Amino Acids in Uterine Flushings of Ewes and Gilts during the Peri-Implantation Period of Pregnancy
Nutrients in uterine secretions are essential for development and survival of conceptuses; therefore, we determined amounts of amino acids in uterine fluids of cyclic and pregnant ewes (Gao et al. 2009a). As summarized in Table 2.1, there is a significant effect of day of pregnancy on amounts of amino acids in uterine flushings from ewes. Similarly, as summarized in Tables 2.2, 2.3 and 2.4, as well as Fig. 2.2, there are significant changes in amino acids in uterine flushing of pigs during the estrous cycle and pregnancy . These results indicate activation of pregnancy-associated mechanisms for transport of amino acids into the uterine lumen, and provide a framework for studies of amino acids required to activate nutrient sensing cell signaling pathways for growth, development and survival of conceptuses, as well as for optimization of culture media for in vitro studies of conceptus development.
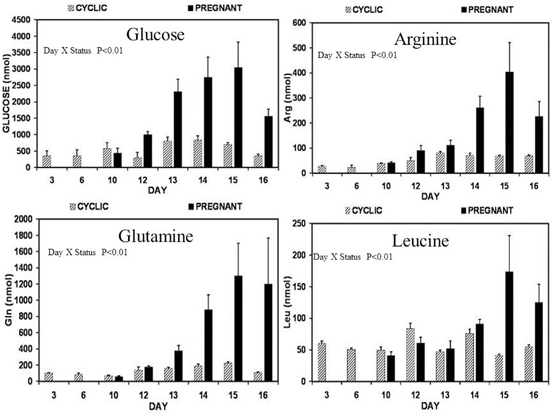
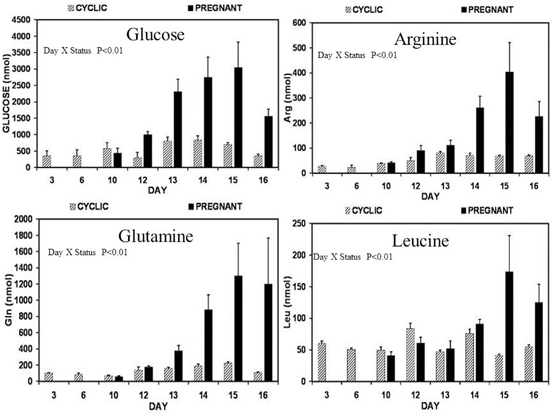
Fig. 2.2
The analysis of uterine flushings from cyclic (cross-hatched bars) and pregnant (solid black bars) for glucose, arginine, glutamine and leucine revealed that total recoverable amounts of these nutrients was not affected by day of the estrous cycle, but increased between Days 10 and 15 in pregnant ewes. Thus, a Day X Pregnancy Status interaction (P < 0.01) was detected. These results reflect effect of the interaction between progesterone and interferon tau to increase expression of nutrient transporters in uterine luminal and superficial glandular epithelia for transport of these nutrients into the uterine lumen to support growth and development of the conceptus (see Gao et al. 2009a, b, c, d)
Table 2.1
Amino acids and ions in uterine flushings from ewes during the peri-implantation period of pregnancy
Components | Days of pregnancya | |||
---|---|---|---|---|
13 (n = 10) | 14 (n = 9) | 15 (n = 8) | 16(n = 9) | |
Asp | 86 ± 21 | 182 ± 49 | 323 ± 45 | 670 ± 99 |
Glu | 501 ± 117 | 987 ± 235 | 1759 ± 203 | 1889 ± 140 |
Asn | 89 ± 18 | 193 ± 43 | 443 ± 47 | 463 ± 44 |
Ser | 891 ± 139 | 1224 ± 214 | 2172 ± 320 | 1039 ± 171 |
Gln | 310 ± 23 | 451 ± 71 | 1209 ± 94 | 1352 ± 121 |
His | 54 ± 9 | 131 ± 32 | 312 ± 33 | 430 ± 45 |
Gly | 4570 ± 610 | 4731 ± 752 | 6033 ± 1168 | 2542 ± 408 |
Thr | 237 ± 44 | 506 ± 113 | 1027 ± 160 | 683 ± 76 |
Cit | 103 ± 19 | 164 ± 43 | 394 ± 51 | 309 ± 34 |
Arg | 64 ± 7 | 121 ± 31 | 459 ± 50 | 804 ± 71 |
β-Ala | 43 ± 5 | 53 ± 8 | 93 ± 11 | 110 ± 15 |
Tau | 501 ± 61 | 565 ± 122 | 699 ± 141 | 434 ± 74 |
Ala | 402 ± 50 | 660 ± 114 | 1252 ± 72 | 1060 ± 106 |
Tyr | 41 ± 5 | 77 ± 15 | 183 ± 22 | 273 ± 23 |
Trp | 13 ± 1 | 28 ± 6 | 56 ± 8 | 69 ± 5 |
Met | 14 ± 2 | 41 ± 11 | 117 ± 19 | 212 ± 19 |
Val | 61 ± 9 | 147 ± 36 | 313 ± 41 | 348 ± 22 |
Phe | 25 ± 3 | 57 ± 13 | 153 ± 25 | 273 ± 29 |
Ile | 26 ± 4 | 55 ± 13 | 132 ± 17 | 151 ± 10 |
Leu | 40 ± 4 | 70 ± 11 | 222 ± 25 | 273 ± 21 |
Orn | 62 ± 5 | 81 ± 10 | 144 ± 14 | 172 ± 13 |
Lys | 82 ± 21 | 262 ± 85 | 780 ± 95 | 1511 ± 158 |
Cys | 39 ±6 | 86 ± 17 | 136 ± 16 | 118 ± 16 |
Ca++ | 830 ± 118 | 901 ± 85 | 1298 ± 172 | 2031 ± 252 |
Na+ | 20782 ± 3839 | 23091 ± 3881 | 38948 ± 5508 | 76951 ± 10598 |
K+ | 49194 ± 4167 | 54851 ± 7890 | 69536 ± 9355 | 50114 ± 4749 |
Table 2.2
Effects of day of the estrous cycle, day of pregnancy and interaction between day and pregnancy status on total recoverable basic and acidic amino acids in porcine uterine flushings (nmol; Means ± SEMs)
Status | Day | Basic amino acids | Acidic amino acids | |||
---|---|---|---|---|---|---|
His | Orn | Lys | Asp | Glu | ||
Estrous cycle | 5 | 154 ± 16c | 61 ± 16a | 63 ± 12b | 245 ± 59b | 5395 ± 1277 a |
9 | 237 ± 94bc | 152 ± 89a | 73 ± 14b | 2121 ± 595a | 12019 ± 4717 a | |
12 | 478 ± 33ab | 118 ± 91a | 364 ±79a | 2111 ± 348a | 13102 ± 2232 a | |
15 | 712 ± 358a | 45 ± 4a | 510 ± 272a | 1622 ± 808a | 5095 ± 2213 a | |
Pregnancy | 9 | 231 ± 59w | 160 ± 81uv | 151 ± 57w | 2356 ± 20u | 12681 ± 2103 u |
10 | 139 ± 40x | 90 ± 23v | 198 ± 42w | 1870 ± 289v | 8415 ± 1148 v | |
12 | 969 ± 206uv | 178 ± 25u | 811 ± 74uv | 2829 ± 503u | 10782 ± 2113 u | |
13 | 649 ± 201vw | 234 ± 88u | 613 ± 273v | 3909 ± 1418u | 11158 ± 2232 u | |
14 | 971 ± 268u | 214 ±57u | 1337 ± 364uv | 2421 ± 563uv | 8888 ± 1195uv | |
15 | 2672 ± 1417u | 286 ± 55u | 1873 ± 589 u | 5099 ± 1343u | 18352 ± 7393 u | |
Two-way ANOVA# | Day effect | 0.001 | 0.91 | < 0.0001 | 0.82 | 0.35 |
Status effect | 0.04 | 0.01 | 0.002 | 0.02 | 0.15 | |
Day x status interaction | 0.30 | 0.45 | 0.51 | 0.09 | 0.05 |
Table 2.3
Effects of day of the estrous cycle, day of pregnancy and interaction between day and pregnancy status on total recoverable small neutral amino acids (≤ C4) and glutathione in porcine uterine flushings (nmol; Means ± SEMs)
Day | Small neutral amino acids | |||||||||
---|---|---|---|---|---|---|---|---|---|---|
Asn | Ser | Gly | Thr | Ala | β-Ala | Tau | Cys | Glutathione | ||
Status | 5 | 90 ± 28c | 1743 ± 209d | 23376 ± 1857c | 130 ± 29b | 931 ± 71c | 587 ± 189b | 47512 ± 10543a | 244 ±51c | 28 ± 4b |
9 | 213 ± 45bc | 35065 ± 4567c | 141320 ± 20219a | 4420 ± 208a | 2618 ± 857bc | 1941 ± 729a | 82763 ± 16622a | 867 ± 127a | 301 ± 127a | |
12 | 537 ± 43a | 213324 ± 26572a | 107883 ± 3719ab | 5784 ± 745a | 3920 ± 1503b | 2056 ± 327a | 59483 ± 13016a | 535 ± 232bc | 470 ± 59a | |
15 | 549 ± 90ab | 123196 ± 32131b | 67291 ± 18009b | 4924 ± 1529a | 12265 ± 4825a | 803 ± 253ab | 50654 ± 16894a | 738 ± 215bc | 258 ± 69a | |
Pregnancy | 9 | 290 ± 45v | 109208 ± 16353u | 103568 ± 10300u | 7922 ± 1506u | 1910 ± 364wx | 1297 ± 218u | 54567 ± 2641u | 508 ± 81vw | 467 ± 56u |
10 | 1039 ± 509uv | 93387 ± 12435u | 63878 ± 6145u | 4562 ± 517uv | 1754 ± 282x | 1068 ± 117uv | 37536 ± 4558v | 401 ± 60w | 193 ± 26u | |
12 | 1974 ± 1041u | 145097 ± 49680u | 81816 ± 30896uv | 4412 ± 1301vw | 6342 ±1691uv | 1637 ± 472u | 57714 ± 17261uv | 841 ± 75v | 385 ± 164u | |
13 | 1118 ± 570uv | 129481 ± 28777u | 75596 ± 10143u | 5005 ± 687u | 8290 ± 996u | 2250 ± 334u | 92243 ± 23068u | 1150 ± 327v | 313 ± 116u | |
14 | 577 ± 136uv | 24504 ± 4351v | 9396 ± 2306w | 1920 ± 530w | 3642 ± 432vw | 226 ± 53w | 27585 ± 5764v | 4105 ± 628u | 53 ± 19v | |
15 | 1607 ± 726uv | 95282 ± 54745uv | 41409 ± 25858vw | 7163 ± 2462u | 8334 ± 2986uv | 1324 ± 738v | 39613 ± 14737v | 5363 ± 1888u | 103 ± 79v | |
Two-way ANOVA | Day effect | 0.006 | 0.09 | 0.04 | 0.64 | 0.003 | 0.17 | 0.22 | 0.009 | 0.02 |
Status effect | 0.26 | 0.73 | 0.08 | 0.7 | 0.87 | 0.55 | 0.28 | 0.02 | 0.14 | |
Day x Status interaction | 0.69 | 0.05 | 0.77 | 0.28 | 0.38 | 0.81 | 0.94 | 0.02 | 0.09 |
Table 2.4.
Effects of day of the estrous cycle, day of pregnancy and interaction between day and pregnancy status on total recoverable large neutral amino acids (≥ C5) in porcine uterine flushings (nmol; Means ± SEMs)
Day | Large neutral amino acids | ||||||||
---|---|---|---|---|---|---|---|---|---|
Cit | Tyr | Trp | Met | Val | Phe | Ile | Pro | ||
Status | 5 | 98 ± 9b | 126 ± 17c | 19 ± 4d | 45 ± 4b | 821 ± 215b | 106 ± 9b | 213 ± 15b | 542 ± 59ab |
9 | 1685 ± 855a | 7600 ± 2810a | 24267 ± 7909a | 559 ± 180a | 3098 ± 1042a | 1124 ± 522a | 844 ± 402a | 2139 ± 785a | |
12 | 1787 ± 619a | 995 ± 248b | 2504 ± 1368b | 372 ± 78a | 1348 ± 140ab
![]() Stay updated, free articles. Join our Telegram channel![]() Full access? Get Clinical Tree![]() ![]() ![]() |