Chapter 26 Amino Acid Metabolism
Amino acids are used for three major purposes:
Only 11 of the 20 amino acids can be synthesized in the human body, either from common metabolic intermediates or from other amino acids. Those that cannot be synthesized are called essential amino acids. The essential amino acids are valine, leucine, isoleucine, phenylalanine, tryptophan, methionine, lysine, histidine, and threonine.
This chapter describes the fate of the amino nitrogen during amino acid catabolism, the pathways by which amino acids are degraded to simple nitrogen-free metabolic intermediates, and the biosynthesis of the nonessential amino acids.
Amino Acids Can Be Used for Gluconeogenesis and Ketogenesis
The adult human body contains approximately 10 kg of protein in addition to small quantities of free amino acids in tissues and body fluids. The plasma concentrations of the 20 amino acids combined are only 20 to 30 mg/dl, one fourth of the blood glucose level.
Figure 26.1 shows an overview of amino acid and protein metabolism. A typical dietary protein intake is 100 g/day. Another 250 to 300 g of amino acids comes from protein breakdown, but the same amount is consumed for protein synthesis. Different proteins turn over at different rates. Most metabolic enzymes have a life expectancy of one or a few days, but most plasma proteins circulate for 1 to 3 weeks. Hemoglobin survives for 120 days, collagen lasts up to several years in some tissues, and the lens proteins last for a lifetime.
Glucogenic amino acids are degraded to intermediates of tricarboxylic acid cycle or glycolysis. They are used for gluconeogenesis during fasting. Ketogenic amino acids are degraded to acetyl-coenzyme A (acetyl-CoA). They are used for ketogenesis during fasting. Only leucine and lysine are purely ketogenic. Some of the larger amino acids, including isoleucine and the three aromatic amino acids phenylalanine, tyrosine, and tryptophan, are both. All other amino acids are glucogenic.
The Nitrogen Balance Indicates The Net Rate of Protein Synthesis
One hundred grams of dietary protein contains approximately 16 g of nitrogen. Eighty-three percent of this ingested nitrogen eventually leaves the body as urea, 7% as ammonium ion and 10% as organic waste products, including uric acid and creatinine. Ninety-five percent of the urea and a large majority of the other nitrogenous wastes are excreted in the urine, but 1 to 2 g of nitrogen from undigested protein is excreted in the stools.
The nitrogen balance is the difference between the nitrogen entering the body and that leaving it. A normal adult with adequate protein intake should be in nitrogen equilibrium (Fig. 26.2). This means that the amount of outgoing nitrogen matches exactly the amount of incoming nitrogen, and the amount of body protein remains constant.
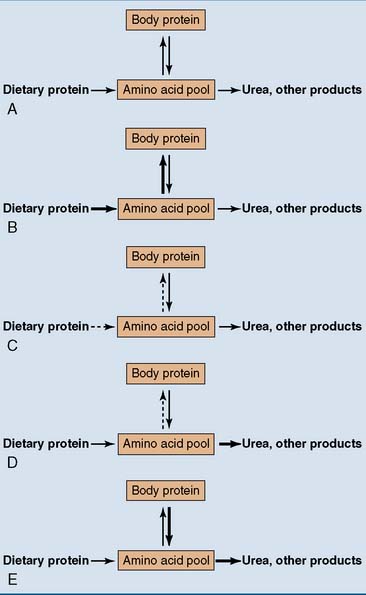
Figure 26.2 Nitrogen balance in different normal and abnormal states. A, Normal adult: nitrogen equilibrium. B, Growth, pregnancy: positive nitrogen balance. C, Protein deficiency: negative nitrogen balance. D, Essential amino acid deficiency: negative nitrogen balance. E, Wasting diseases, burns, trauma: negative nitrogen balance.
A positive nitrogen balance is observed when nitrogen intake exceeds nitrogen excretion. It implies that the amount of body protein increases. Growing children, pregnant women, bodybuilders, and patients recovering from severe illnesses have a positive nitrogen balance. Therefore they have an increased requirement for dietary protein.
A negative nitrogen balance is observed in dietary protein deficiency. Even the protein-starved body degrades 30 to 40 g of amino acids every day. This amount defines the dietary requirement. Essential amino acid deficiency has the same effect because protein synthesis is impaired even if only one of the essential amino acids is missing.
Patients with chronic infections, cancer, or other severe diseases have a negative nitrogen balance because glucocorticoids and other stress hormones favor protein degradation over protein synthesis, thereby supplying amino acids for gluconeogenesis. Some cytokines—biologically active proteins released by white blood cells in many diseases—have catabolic effects similar to those of the stress hormones.
The Amino Group of Amino Acids Is Released as Ammonia
During amino acid catabolism, some of the amino acid nitrogen is released as ammonia. The most important ammonia-forming reaction is the oxidative deamination of glutamate by glutamate dehydrogenase in liver and other tissues (Fig. 26.3, A). This reversible reaction can function in both the synthesis and the degradation of glutamate. The enzyme uses mainly nicotinamide adenine dinucleotide (NAD+) for glutamate degradation and reduced nicotinamide adenine dinucleotide phosphate (NADPH) for glutamate synthesis. The glutamate dehydrogenase reaction implies that glutamate is both nonessential and glucogenic.
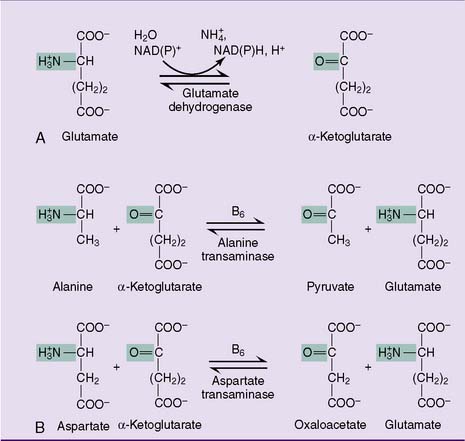
Figure 26.3 Fate of the amino group during amino acid catabolism. A, Release of ammonia from glutamate in the glutamate dehydrogenase reaction. Although reversible, this reaction functions in glutamate degradation under most conditions. NAD(P), Nicotinamide adenine dinucleotide (phosphate); NAD(P)H, reduced form of NAD(P). B, Transamination of alanine and aspartate. These reversible reactions transfer the amino group to α-ketoglutarate, forming glutamate. From glutamate, the nitrogen can be released as ammonia in the glutamate dehydrogenase reaction.
Most other amino acids do not form ammonia directly. They transfer their α-amino group to α-ketoglutarate to form glutamate. The enzymes that catalyze these reversible amino group transfers are called transaminases or aminotransferases. Examples are shown in Figure 26.3, B.
Alternatively, the glutamate that accumulates from these transaminase reactions can be used to make aspartate via aspartate transaminase operating in the reverse direction (Fig. 26.3, B). Both ammonia and aspartate are sources of nitrogen for the synthesis of urea (see section about urea cycle).
All transaminases contain pyridoxal phosphate (PLP), the coenzyme form of vitamin B6, as a prosthetic group. PLP is bound to the active site of the enzyme by electrostatic interactions and by a Schiff base (aldimine) bond with a lysine side chain of the apoprotein. PLP participates directly in the reaction as shown in Figure 26.4.
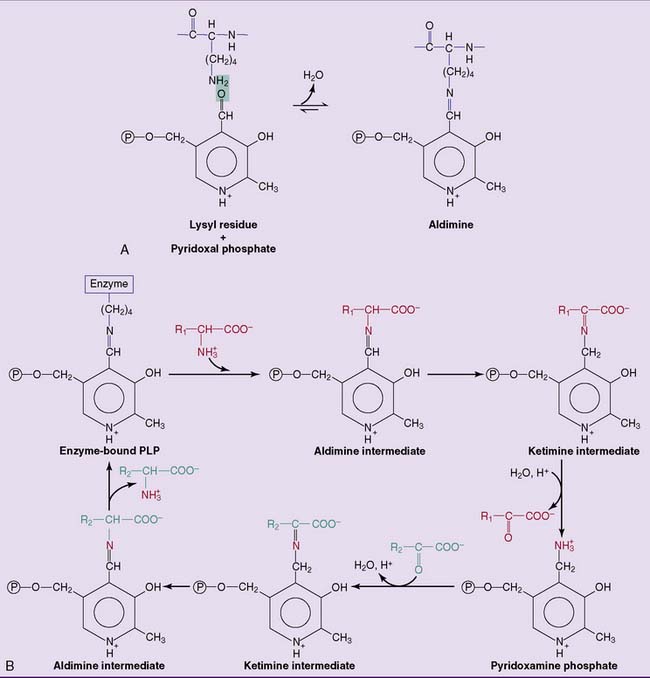
Figure 26.4 Mechanism of transamination reactions. A, Pyridoxal phosphate (PLP) is bound to a lysine side chain in the apoprotein by an aldimine (“Schiff base”) bond. B, Catalytic cycle. Transamination is a sequence of two reactions in which the prosthetic group of the enzyme participates as a reactant.
Ammonia Is Detoxified to Urea
Ammonia is a hazardous waste. It is neurotoxic even in low concentrations, so it must be disposed of quickly. Being unable to excrete ammonia fast enough, humans turn it into nontoxic, water-soluble, and therefore easily excretable urea.
Urea is the diamide of carbonic acid. This dry statement implies that urea can be cleaved into carbonic acid and ammonia. The reaction is catalyzed by the bacterial enzyme urease:
Humans do not possess urease, but some bacteria do. The bacterium Proteus mirabilis causes urinary tract infections in which the bacterial urease produces large amounts of ammonia. The ammonia alkalinizes the urine, causing the formation of large kidney stones (“staghorn calculi”) consisting of magnesium ammonium phosphate. Also, the sharp smell of latrines is ammonia formed by urease-producing bacteria.
Because urea contains 48% nitrogen by weight and proteins contain 16%, 3 g of dietary protein forms 1 g of urea—a total of 33 g urea on a diet of 100 g protein per day.
The blood urea level is measured as blood urea nitrogen (BUN). In health, the level is 8 to 20 mg/dl. The BUN rises sharply in renal failure. This condition is called uremia. Urea is not responsible for most of the clinical manifestations of uremia, but BUN is a convenient measure for the retention of nitrogenous wastes.
Urea Is Synthesized in the Urea Cycle
Most amino acid catabolism takes place in the liver, and the liver is also the only important site for the synthesis of urea in the urea cycle. Nitrogen enters the cycle in the form of carbamoyl phosphate, which is synthesized in liver mitochondria from ammonia, carbon dioxide, and ATP:
The use of two ATP molecules makes this reaction irreversible. The carbamoyl phosphate synthetase requires N-acetylglutamate as an activator. This regulatory metabolite is formed by a separate enzyme from glutamate and acetyl-CoA. N-acetylglutamate is formed when glutamate is increased and indicates that the urea cycle should be turned on to get rid of nitrogen. Carbamoyl phosphate synthetase has a very high affinity for its substrate ammonia (Km = 250 μmol/L) and therefore can maintain the ammonia concentration at a low level of 30 to 60 μmol/L at all times.
The reactions of the urea cycle proper are shown in Figure 26.5. One of the two nitrogen atoms in urea comes from ammonia via carbamoyl phosphate and the other from aspartate. Most of the aspartate nitrogen is derived from transamination reactions in the liver (Fig. 26.6).
Synthesis of one urea molecule requires four high-energy phosphate bonds. Two ATP molecules are converted to ADP in the carbamoyl phosphate synthetase reaction, and two additional phosphate bonds are consumed for the formation of argininosuccinate, when one ATP molecule is hydrolyzed to AMP and inorganic pyrophosphate. The nitrogen is committed to urea synthesis because the two reactions that introduce it into the cycle are made irreversible by ATP hydrolysis.
Failure of the urea cycle leads to ammonia toxicity and encephalopathy. Inherited urea cycle enzyme deficiencies (see Clinical Example 26.1) are rare conditions with a combined incidence of 1:8000. In addition to ammonia, glutamine is elevated because excess ammonia is diverted into glutamine synthesis. The substrate of the defective enzyme also accumulates. Death in infancy or severe disability is inevitable when a urea cycle enzyme deficiency is complete, but partial enzyme deficiencies lead to milder impairments.
A far more common cause of hyperammonemia is liver failure. Patients develop a form of encephalopathy that is caused in large part by ammonia toxicity but also is related to other aspects of impaired liver function (Clinical Example 26.2).
CLINICAL EXAMPLE 26.1: Ornithine Transcarbamoylase Deficiency
With an estimated incidence of 1:14,000, X-linked ornithine transcarbamoylase deficiency is the most common inherited urea cycle enzyme deficiency. In classic cases, affected males become lethargic and anorexic between 1 and 3 days after birth. Vomiting, hypothermia, and hyperventilation may be present, and laboratory investigations show respiratory alkalosis. Cerebral edema develops because of the osmotic activity of glutamine accumulating in astrocytes, and many patients die within weeks after birth.
The diagnosis is established by findings of elevated blood ammonia, glutamine, and ornithine and reduced blood urea nitrogen. Orotic acid appears in blood and urine because accumulating carbamoyl phosphate leaks from the mitochondria into the cytoplasm, where it is a precursor of orotic acid in the pathway of pyrimidine synthesis (see Chapter 28).
Treatment includes dietary protein restriction, combined with sufficient carbohydrate to prevent the breakdown of body protein for gluconeogenesis. Patients are fed high doses of benzoic acid and phenylacetic acid, which are excreted in the urine as conjugation products with glycine and glutamine, respectively (Fig. 26.7). Ordinarily, these reactions are detoxification reactions that convert the unwanted acids into water-soluble excretable products. In this context, however, they are exploited as alternative routes of nitrogen excretion. Arginine or, preferably, citrulline is administered because the urea cycle functions as a biosynthetic pathway for arginine. For patients with urea cycle enzyme deficiencies, arginine is an essential amino acid.
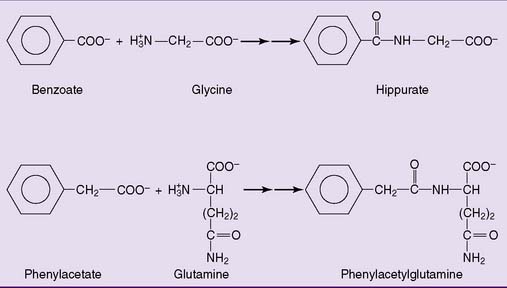
Figure 26.7 Use of benzoate and phenylacetate for treatment of hyperammonemia in liver cirrhosis and inherited urea cycle enzyme deficiencies. The amino acid conjugates of these organic acids provide alternative routes of nitrogen excretion.
Incomplete deficiencies lead to mild forms of the disease that become symptomatic in older children or adults, usually during episodes of dieting or starvation, when a large amount of body protein is degraded to provide substrate for gluconeogenesis. In addition, some carrier females develop signs of encephalopathy in these situations.
CLINICAL EXAMPLE 26.2: Hepatic Encephalopathy
Liver cirrhosis is defined by progressive loss of hepatocytes, which are replaced by fibrous connective tissue. It is an end point of many pathological processes, with alcoholism being the most common cause.
The proliferating connective tissue impairs blood flow through the liver. This leads to portal hypertension and the development of a collateral circulation. Venous channels in the lower esophagus and in the periumbilical, rectal, and retroperitoneal areas dilate, shunting blood from the portal vein to the systemic circulation. Portal hypertension is dangerous because it can result in the rupture of veins and fatal hemorrhage, especially in the lower esophagus.
The biochemical derangements in liver cirrhosis cause hepatic encephalopathy, also called portal systemic encephalopathy because the shunting of blood around the cirrhotic liver contributes to the problem. It manifests with slurring of speech, blurring of vision, motor incoordination (ataxia), a characteristic coarse flapping tremor (asterixis), and mental derangements. The condition can progress to hepatic coma and death. Although other biochemical abnormalities have been implicated as well, hyperammonemia is a major cause of the central nervous system disorder.
Foul-smelling breath is an important diagnostic sign. It is caused by volatile sulfhydryl compounds (mercaptans) that are formed from dietary cysteine and methionine by intestinal bacteria. Ordinarily, the mercaptans are oxidized to nonvolatile, odorless products in the liver. In liver cirrhosis, however, they reach the lungs and are exhaled.
A low-protein, alcohol-free diet is the best long-term treatment of liver cirrhosis. The replacement of essential amino acids by their corresponding α-keto acids is an effective (but expensive) way of reducing the total nitrogen intake without precipitating essential amino acid deficiencies. Because of the transamination reactions, most of the essential amino acids are no longer essential when their corresponding α-keto acids are present in the diet.
Intestinal bacteria are an important source of ammonia. They ferment undigested proteins under formation of ammonia, and they cleave the urea that is present in digestive secretions into carbonic acid and ammonia. Therefore hepatic encephalopathy can be treated with sterilization of the gastrointestinal tract by broad-spectrum antibiotics, although there is a danger of overgrowth by drug-resistant bacteria with resulting enterocolitis.
Some Amino Acids Are Closely Related to Common Metabolic Intermediates
Some amino acids are close relatives of intermediates in the major metabolic pathways. Alanine is related to pyruvate through the reversible alanine transaminase reaction:
Glutamate is similarly interconvertible with α-ketoglutarate, both by transamination and the glutamate dehydrogenase reaction. Glutamine is both synthesized from and degraded to glutamate:
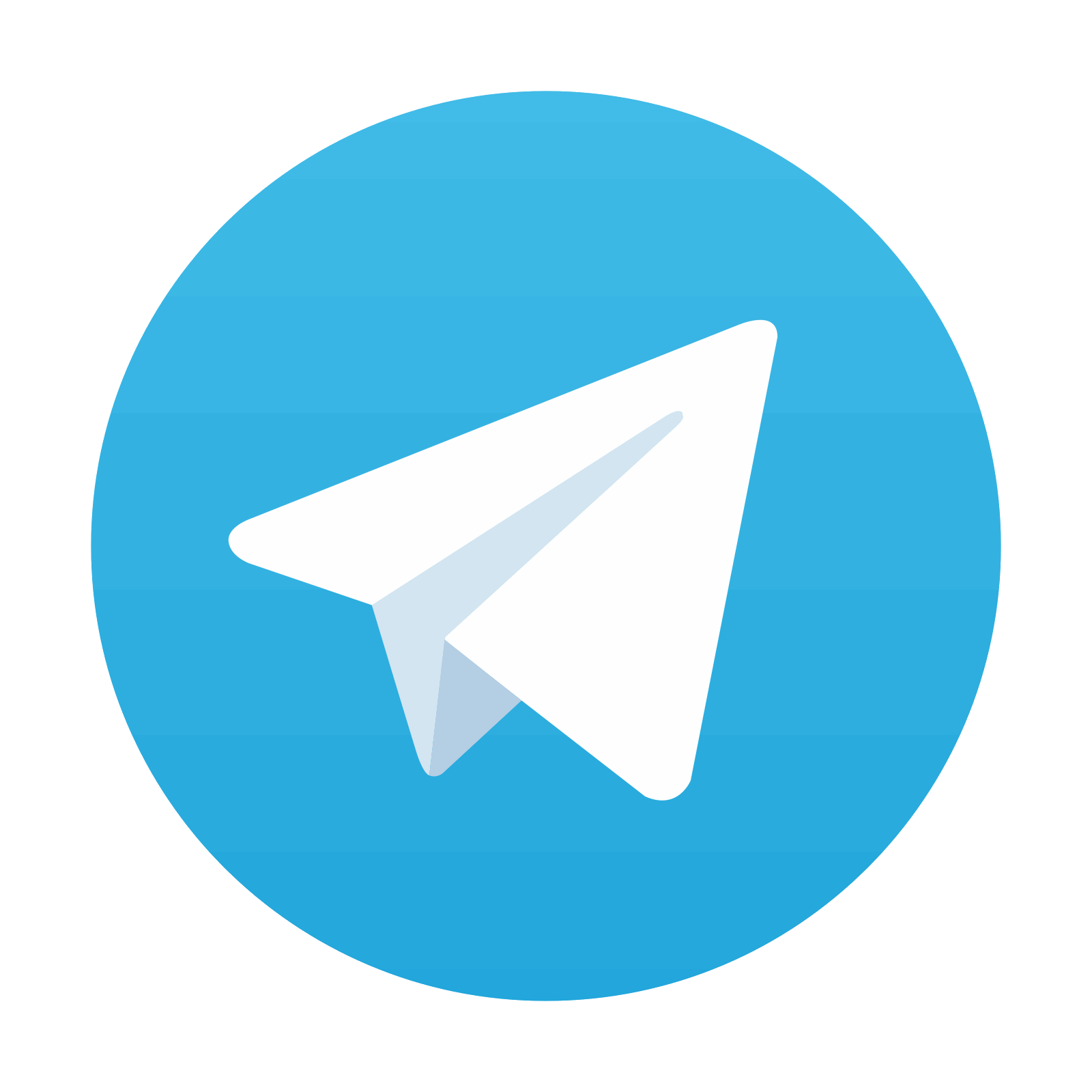
Stay updated, free articles. Join our Telegram channel

Full access? Get Clinical Tree
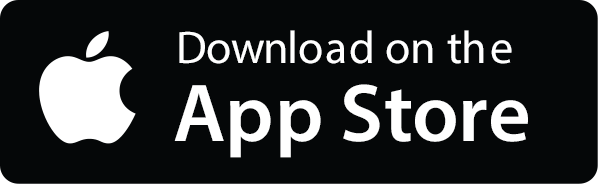
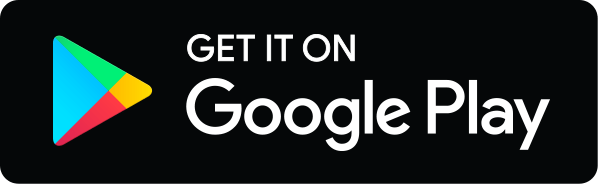