
to generate α,β-unsaturated aldehyde polymers. Progression to the higher polymeric form can also occur with increased time and pH. As the pH is raised, n increases (see Figure 23.3) in value until the polymer precipitates from solution. Loss of reactive aldehyde groups could therefore be responsible for the rapid loss of biocidal activity of alkaline solutions on storage. A study into the structure of the solid aldol condensation product showed that polymerization of glutaraldehyde under basic conditions results in the formation of water-soluble and water-insoluble polymers and that these polymers contain unconjugated aldehyde, conjugated aldehyde, hydroxyl, and carboxyl groups.19 A number of other studies investigated the several products formed in the aldol condensation of glutaraldehyde and concluded that these new oligomers were glutaraldehyde trimer, pentamer, and heptamer having a 1,3,5-trioxane skeleton.20,21,22,23,24 The glutaraldehyde trimer, 2,4,6-tris(4-oxobutyl)-1,3,5-trioxane, was named “paraglutaraldehyde,” and this was proposed as being responsible for the precipitation observed occasionally during chemosterilization with alkaline glutaraldehyde.
![]() FIGURE 23.2 Glutaraldehyde polymerization in aqueous acid media. A, Monomer. B, Cyclic hemiacetal. C, Acetal-like polymer. D, Monohydrate. E, Dihydrate. |
![]() FIGURE 23.3 Glutaraldehyde polymerization in aqueous alkaline media. Aldol-type polymer (A) progresses to a higher polymeric form (B) with time and increase pH. |
![]() FIGURE 23.4 Influence of time, temperature, and pH on the biocidal activity of acid and alkaline glutaraldehyde solutions: 1a,b,c and 2a,b relate to state of the molecule as described in Figures 23.2 and 23.3. |
glutaraldehyde with hydroxylamine, hydrazine, and methylamine and found that a characteristic change in absorbance occurred.31 From this, a spectrophotometric method was developed for the determination of low concentrations of the aldehyde in the presence of substrates absorbing in the same wavelength region. A microplate photometric method based on N-methylbenzo-thiazol-(2)-hydrazone and 4-amino-3-hydrazino-5-mercapto-1,2,4-triazole has been developed to measure various aldehydes, including glutaraldehyde, in disinfectant solutions.32 Other methods of analysis have been developed for specific reasons such as the determination of glutaraldehyde at low levels in hospital air.33 Chromatographic methods have also been described. These gas chromatographic34,35 and high-performance liquid chromatographic (HPLC) methods22 have compared favorably with UV methods. It is advised that the chromatographic methods should be used in preference to the UV-absorption (235/280 nm) or UV spectrophotometric method (hydroxylamine).23,36 A HPLC method for glutaraldehyde determination was defined and validated by derivatization using 2,4-dinitrophenylhydrazine. The method was validated for concentration ranging from 1.25 mg/L with a detection limit of 0.2 mg/L.37
TABLE 23.1 Lethal effects of aqueous 2% alkaline glutaraldehyde solutionsa | ||||||||||||||||||||||||||||||||||||
---|---|---|---|---|---|---|---|---|---|---|---|---|---|---|---|---|---|---|---|---|---|---|---|---|---|---|---|---|---|---|---|---|---|---|---|---|
|
TABLE 23.2 Comparative sporicidal activities of some aldehydes | ||||||||||||||||||||||||
---|---|---|---|---|---|---|---|---|---|---|---|---|---|---|---|---|---|---|---|---|---|---|---|---|
|
TABLE 23.3 Comparison of the sporicidal activity of formaldehyde and glutaraldehydea | ||||||||||||||||||||
---|---|---|---|---|---|---|---|---|---|---|---|---|---|---|---|---|---|---|---|---|
|
globigii in 3 hours or less.60 A similar result was obtained with a challenge of 106 spores dried onto aluminum foil. A 3-hour exposure was recommended at that time to be sufficient for practical purposes, particularly as spores are infrequent on clean medical equipment. Other work, using similar time-survivor measurements and aqueous suspensions of B subtilis spores, indicated that a 3-hour period gave approximately a 6 log drop in viable count.61,62,63,64
TABLE 23.4 Susceptibilities of nonsporing bacteria to 0.02% aqueous alkaline glutaraldehydea | |||||||||||||||||||||||||||||
---|---|---|---|---|---|---|---|---|---|---|---|---|---|---|---|---|---|---|---|---|---|---|---|---|---|---|---|---|---|
|
which produced results more closely related to M tuberculosis in the suspension test employed. Once again, a glutaraldehyde formulation was found to be rapidly mycobactericidal. Variation in resistance to glutaraldehyde was shown by different strains of mycobacteria, with strains of Mycobacterium avium and Mycobacterium intracellulare requiring over 20 and 40 minutes, respectively, to achieve a 99% kill.80 Differences in sensitivity to glutaraldehyde between laboratory strains and clinical isolates had been observed previously.81 The antimycobacterial activity of glutaraldehyde is summarized in Table 23.5. But it is interesting to note increasing reports of high-level resistance to glutaraldehyde in atypical mycobacteria strains (Mycobacterium chelonae, Mycobacterium massiliense) that have been isolated from environmental conditions (eg, water and equipment) and from patient infections.79,86,87,88,89 Some of these strains were also reported to be cross-resistant to other aldehydes (OPA) and demonstrated increased virulence in animal studies.89,90,91 The resistance mechanisms have been studied and is most likely to be due to the lack of appreciable or sensitive protein molecules at the surface of the mycobacterial cell wall in these strains, thereby limiting the activity or penetrability of glutaraldehyde.92
TABLE 23.5 Mycobacterial activity of glutaraldehyde | |||||||||||||||||||||||||||||||||||||||||||||||||||||||||||||||||||||
---|---|---|---|---|---|---|---|---|---|---|---|---|---|---|---|---|---|---|---|---|---|---|---|---|---|---|---|---|---|---|---|---|---|---|---|---|---|---|---|---|---|---|---|---|---|---|---|---|---|---|---|---|---|---|---|---|---|---|---|---|---|---|---|---|---|---|---|---|---|
|
Aspergillus fumigatus were found to be the most resistant fungi encountered in a comparative study of fungicidal activity of disinfectants.97 Sonacide® (an acid-based glutaraldehyde formulation) was effective against both fungi; however, a glutaraldehyde-phenate mixture (Sporicidin®) was not effective, even after 90 minutes of contact.
![]() FIGURE 23.7 Effect of 0.5% acid or alkaline glutaraldehyde formulation on spores of Aspergillus niger. From Gorman and Scott.96 |
necessitated a reduction in contact time for decontamination in many instances, and this has inevitably led to the reported cases. Indeed, 45 minutes of glutaraldehyde contact was necessary to achieve sterilization of heavily contaminated flexible-fiber bronchoscopes.123 It is also likely that in many of these cases, the disinfectant was not used appropriately or indeed cross-contamination following disinfection due to the use of tap water (which is often contaminated with pathogens are low levels at the point of use).
failure to replace solutions that have deteriorated on standing, or even dilution of residual glutaraldehyde solution may all modify the outcome of disinfection. Illustrating this point, the “reuse” of glutaraldehyde solutions has been investigated.136 Samples of the disinfectant were collected from baths over the recommended 14 day reuse period, analyzed, and assessed for activity against M bovis and hepatitis A virus. Glutaraldehyde levels dropped to approximately 1% (from 2.25% initial) by 14 days. Due attention should therefore be exercised in the use of glutaraldehyde, as with any disinfectant, to avoid such an occurrence especially in view of the resurgence of pathogens such as mycobacteria, viruses, and antibiotic-resistant bacteria.
TABLE 23.6 Revival of alkaline glutaraldehyde-treated Bacillus subtilis spores after coat removal and resuscitationa | |||||||||||||||||||||||||||||||||||||||||||||||||||||||||||||||||||||||||
---|---|---|---|---|---|---|---|---|---|---|---|---|---|---|---|---|---|---|---|---|---|---|---|---|---|---|---|---|---|---|---|---|---|---|---|---|---|---|---|---|---|---|---|---|---|---|---|---|---|---|---|---|---|---|---|---|---|---|---|---|---|---|---|---|---|---|---|---|---|---|---|---|---|
|
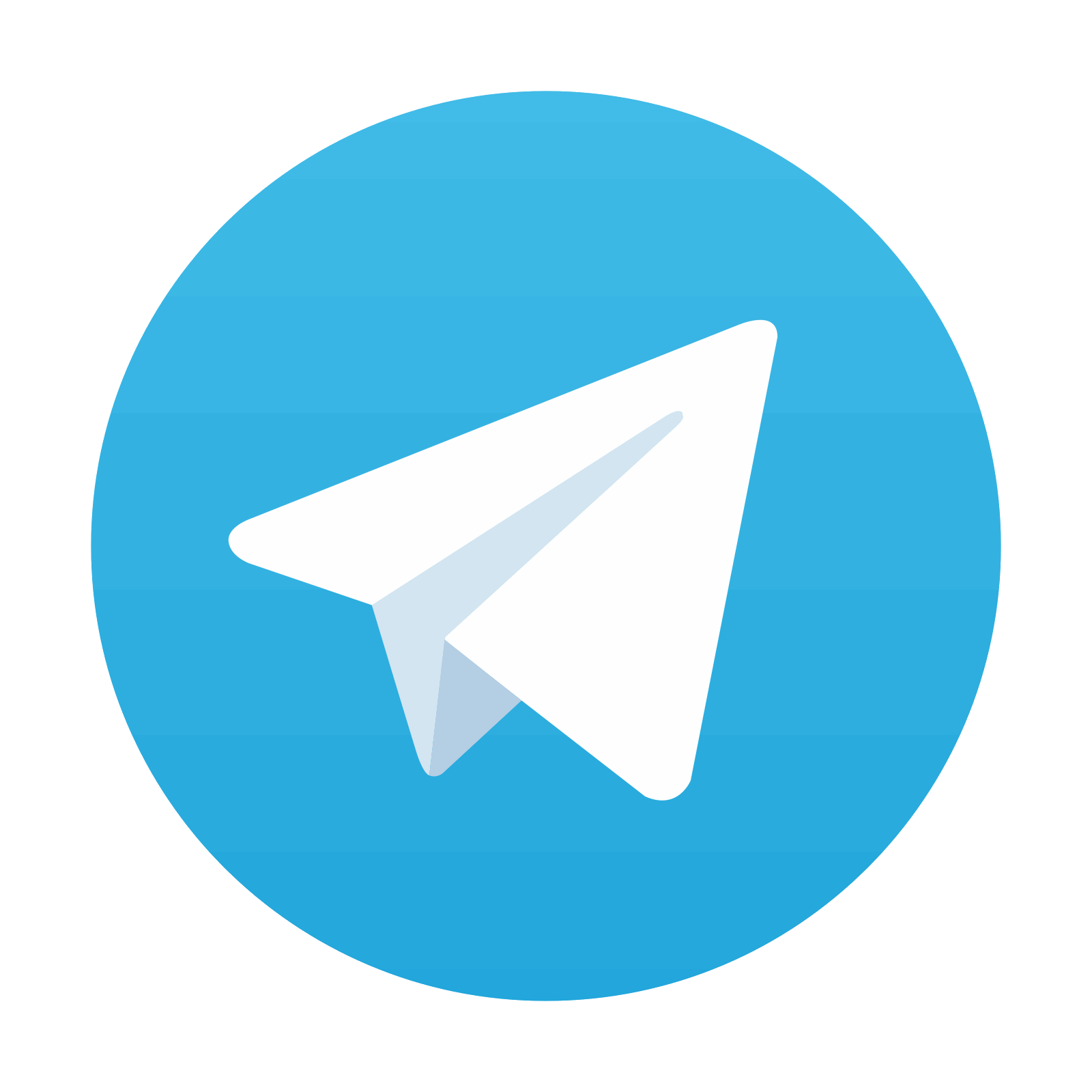
Stay updated, free articles. Join our Telegram channel

Full access? Get Clinical Tree
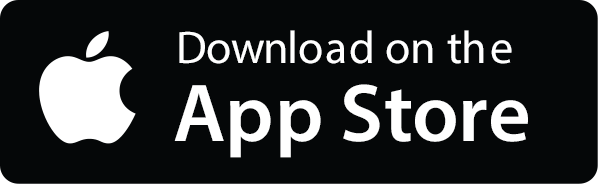
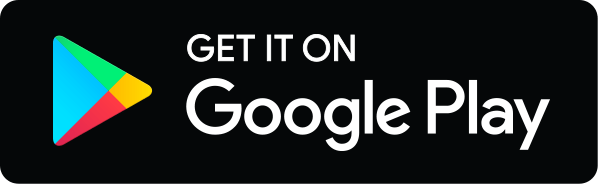
