INTRODUCTION
The non–spore-forming gram-positive bacilli are a diverse group of aerobic and anaerobic bacteria. This chapter focuses on the aerobic members of this group. The anaerobic, non–spore-forming gram-positive bacilli such as Propionibacterium species and Actinomyces species are discussed in Chapter 21 on anaerobic infections. Specific genera of both groups, namely, Corynebacterium species and Propionibacterium species, are members of the normal microbiota of skin and mucous membranes of humans and, as such, are frequently contaminants of clinical specimens submitted for diagnostic evaluation. However, among the aerobic gram-positive bacilli are significant pathogens such as Corynebacterium diphtheriae, an organism that produces a powerful exotoxin that causes diphtheria in humans, and Mycobacterium tuberculosis (see Chapter 23), the causative agent of tuberculosis. Listeria monocytogenes and Erysipelothrix rhusiopathiae are primarily found in animals and occasionally cause severe disease in humans. Nocardia and Rhodococcus species are found in the soil and are significant pathogens among immunocompromised patients.
Corynebacterium species and related bacteria tend to be clubbed or irregularly shaped; although not all isolates have the irregular shapes, the terms “coryneforms” or “diphtheroid bacteria” are convenient ones for denoting this broad group. These bacteria have a high guanosine plus cytosine content and include the genera Corynebacterium, Arcanobacterium, Mycobacterium, and others (Table 12-1). Actinomyces and Propionibacterium are classified as anaerobes, but some isolates grow well aerobically (aerotolerant) and must be differentiated from the aerobic coryneform bacteria. Other non–spore-forming gram-positive bacilli have more regular shapes and a lower guanosine plus cytosine content. The genera include Listeria and Erysipelothrix; these bacteria are more closely related to the anaerobic Lactobacillus species, which sometimes grow well in air, to the spore-forming Bacillus and Clostridium species—and to the gram-positive cocci of the Staphylococcus and Streptococcus genera—than they are to the coryneform bacteria. The medically important genera of aerobic gram-positive bacilli are listed in Table 12-1. Anaerobic bacteria are discussed in Chapter 21.
Organism | General Characteristics | Disease Associations |
---|---|---|
Corynebacterium | ||
Corynebacterium diphtheriae Corynebacterium ulcerans Corynebacterium pseudotuberculosis Corynebacterium striatum Corynebacterium urealyticum Corynebacterium jeikeium | Club-shaped rods that form metachromatic granules; black colonies on tellurite media Grayish white colonies; urease positive Yellowish white colonies; urease positive Produces urea; multidrug resistant Grayish white colonies; multidrug resistant | Toxigenic strains—diphtheria Nontoxigenic strains—bacteremia, endocarditis Toxigenic strains may cause diphtheria Toxigenic strains may cause diphtheria Hospital-acquired infections, esp. lower respiratory Encrusted cystitis and pyelitis Bacteremia and other infections in immunocompromised hosts |
Arcanobacterium hemolyticum | Catalase negative coccobacilli; β-hemolytic | Pharyngitis; wound infections; septicemia |
Rothia | ||
Rothia dentocariosa Rothia mucilaginosa | Branching filaments Coccoid morphology; whitish colonies | Abscesses; endocarditis Bacteremia, endocarditis in injection drug users and immunocompromised patients |
Listeria monocytogenes | Short, thin, gram-positive rods; catalase-positive motile, non–spore-forming; exhibit β-hemolysis | Foodborne gastroenteritis in immunocompetent hosts; neonatal sepsis and meningitis; postpartum infections; meningoencephalitis, bacteremia, septicemia in immunocompromised patients |
Erysipelothrix rhusiopathiae | Appears singly, as short chains or branching filaments; α-hemolytic on blood agar; produces H2S | Erysipeloid; bacteremia; endocarditis |
Nocardia | ||
Nocardia brasiliensis Nocardia abscessus Nocardia nova complex Nocardia transvalensis complex Nocardia farcinica Nocardia asteroides Nocardia cyriacigeorgica | Thin, branching, and beaded modified acid-fast positive rods; yellowish wrinkled colonies Thin, branching, and beaded modified acid-fast positive rods Thin, branching, and beaded modified acid-fast positive rods Thin, branching, and beaded modified acid-fast positive rods Thin, branching, and beaded modified acid-fast positive rods; smooth colonies that turn orange Thin, branching, and beaded modified acid-fast positive rods; chalky white colonies Thin, branching, and beaded modified acid-fast positive rods; powdery colonies with aerial hyphae | Cutaneous lesions associated with trauma including mycetoma Pulmonary and brain abscesses Several species in this complex associated with a broad range of clinical syndromes Pulmonary and brain abscesses Among the most resistant Nocardia sp., this pathogen causes disseminated disease esp. in immunocompromised patients Infrequent cause of infections Most common Nocardia sp. in clinical material; respiratory infections, wounds, brain abscess |
Rhodococcus equi | Coccoid organisms that are modified acid-fast positive; salmon pink smooth colonies | Pneumonia, often with cavity formation in immunocompromised patients |
Actinomadura | ||
Actinomadura madurae Actinomadura pelletieri | Thin, short, branching filaments; appear as grains in tissue; colonies are wrinkled and may have color Thin short branching filaments; appear as grains in tissue; colonies are wrinkled and may have color | Mycetoma (Madura foot) Mycetoma |
Streptomyces somaliensis | Acid-fast negative; colonies appear wrinkled with aerial hyphae | Mycetoma |
There is no unifying method for identification of the gram-positive bacilli. Few laboratories are equipped to measure guanosine plus cytosine content. Growth only under anaerobic conditions implies that the isolate is an anaerobe, but many isolates of Lactobacillus, Actinomyces, and Propionibacterium species and others are aerotolerant. Most isolates of Mycobacterium, Nocardia, and Rhodococcus species are acid fast and are therefore readily distinguished from the coryneform bacteria. Many, but not all, genera of Bacillus and Clostridium produce spores, and the presence of spores readily distinguishes the isolate from the coryneform bacteria when present. Determination that an isolate is a Lactobacillus (or Propionibacterium) may require gas–liquid chromatography to measure lactic acid (or propionic acid) metabolic products, but this is generally not practical. Other tests that are used to help identify an isolate of non–spore-forming gram-positive bacilli as a member of a genus or species include catalase production, indole production, nitrate reduction, and fermentation of carbohydrates, among others. Many clinical laboratories have developed sequencing technologies targeting the 16S rRNA gene or other gene targets for identification of many of these organisms, but especially for Mycobacterium and Nocardia species recovered from clinical specimens. A relatively new technology recently introduced into microbiology laboratories involves the use of matrix-assisted laser desorption/ionization time-of-flight mass spectroscopy (MALDI-TOF MS) that allows for assessment of ribosomal proteins whose spectral patterns are unique enough to identify organisms to the species level. This technology works well for identification of a broad range of bacteria including corynebacteria and anaerobes, although less data are available on more complex bacteria such as Mycobacterium species. This technology is discussed in more detail in Chapter 47.
CORYNEBACTERIUM DIPHTHERIAE
Corynebacteria are 0.5–1 μm in diameter and several micrometers long. Characteristically, they possess irregular swellings at one end that give them the “club-shaped” appearance (Figure 12-1). Irregularly distributed within the rod (often near the poles) are granules staining deeply with aniline dyes (metachromatic granules) that give the rod a beaded appearance. Individual corynebacteria in stained smears tend to lie parallel or at acute angles to one another. True branching is rarely observed in cultures.
On blood agar, the C diphtheriae colonies are small, granular, and gray with irregular edges and may have small zones of hemolysis. On agar containing potassium tellurite, the colonies are brown to black with a brown-black halo because the tellurite is reduced intracellularly (staphylococci and streptococci can also produce black colonies). Four biotypes of C diphtheriae have been widely recognized and each of them produces the potent exotoxin: gravis, mitis, intermedius, and belfanti. These variants have been classified on the basis of growth characteristics such as colony morphology, biochemical reactions, and severity of disease produced by infection. Very few reference laboratories are equipped with methods to provide reliable biotype characterization. The incidence of diphtheria has greatly decreased and the association of severity of disease with biovar is no longer important to clinical or public health management of cases or outbreaks. If necessary, in the setting of an outbreak, immunochemical and preferably molecular methods such as ribotyping can be used to type the C diphtheriae isolates.
C diphtheriae and other corynebacteria grow aerobically on most ordinary laboratory media. On Löffler serum medium, corynebacteria grow much more readily than other respiratory organisms, and the morphology of organisms is typical in smears made from these colonies.
Corynebacteria tend to pleomorphism in microscopic and colonial morphology. When some nontoxigenic diphtheria organisms are infected with bacteriophage from certain toxigenic diphtheria bacilli, the offspring of the exposed bacteria are lysogenic and toxigenic, and this trait is subsequently hereditary. When toxigenic diphtheria bacilli are serially subcultured in specific antiserum against the temperate phage that they carry, they tend to become nontoxigenic. Thus, acquisition of phage leads to toxigenicity (lysogenic conversion). The actual production of toxin occurs perhaps only when the prophage of the lysogenic C diphtheriae becomes induced and lyses the cell. Whereas toxigenicity is under the control of the phage gene, invasiveness is under the control of bacterial genes.
The principal human pathogen of the genus Corynebacterium is C diphtheriae, the causative agent of respiratory or cutaneous diphtheria. In nature, C diphtheriae occurs in the respiratory tract, in wounds, or on the skin of infected persons or normal carriers. It is spread by droplets or by contact to susceptible individuals; the bacilli then grow on mucous membranes or in skin abrasions, and those that are toxigenic start producing toxin.
All toxigenic C diphtheriae are capable of elaborating the same disease-producing exotoxin. In vitro production of this toxin depends largely on the concentration of iron. Toxin production is optimal at 0.14 μg of iron per milliliter of medium but is virtually suppressed at 0.5 μg/mL. Other factors influencing the yield of toxin in vitro are osmotic pressure, amino acid concentration, pH, and availability of suitable carbon and nitrogen sources. The factors that control toxin production in vivo are not well understood.
Diphtheria toxin is a heat-labile, single-chain, three-domain polypeptide (62 kDa) that can be lethal in a dose of 0.1 μg/kg body weight. If disulfide bonds are broken, the molecule can be split into two fragments. Fragment B (38 kDa), which has no independent activity, is functionally divided into a receptor domain and a translocation domain. The binding of the receptor domain to host cell membrane proteins CD-9 and heparin-binding epidermal growth factor (HB-EGF) triggers the entry of the toxin into the cell through receptor-mediated endocytosis. Acidification of the translocation domain within a developing endosome leads to creation of a protein channel that facilitates movement of Fragment A into the host cell cytoplasm. Fragment A inhibits polypeptide chain elongation—provided nicotinamide adenine dinucleotide (NAD) is present—by inactivating the elongation factor EF-2. This factor is required for translocation of polypeptidyl-transfer RNA from the acceptor to the donor site on the eukaryotic ribosome. Toxin Fragment A inactivates EF-2 by catalyzing a reaction that yields free nicotinamide plus an inactive adenosine diphosphate-ribose-EF-2 complex (ADP-ribosylation). It is assumed that the abrupt arrest of protein synthesis is responsible for the necrotizing and neurotoxic effects of diphtheria toxin. An exotoxin with a similar mode of action can be produced by strains of Pseudomonas aeruginosa.
Diphtheria toxin is absorbed into the mucous membranes and causes destruction of epithelium and a superficial inflammatory response. The necrotic epithelium becomes embedded in exuding fibrin and red and white cells, so that a grayish “pseudomembrane” is formed—commonly over the tonsils, pharynx, or larynx. Any attempt to remove the pseudomembrane exposes and tears the capillaries and thus results in bleeding. The regional lymph nodes in the neck enlarge, and there may be marked edema of the entire neck, with distortion of the airway, often referred to as “bull neck” clinically. The diphtheria bacilli within the membrane continue to produce toxin actively. This is absorbed and results in distant toxic damage, particularly parenchymatous degeneration, fatty infiltration, and necrosis in heart muscle (myocarditis), liver, kidneys (tubular necrosis), and adrenal glands, sometimes accompanied by gross hemorrhage. The toxin also produces nerve damage (demyelination), often resulting in paralysis of the soft palate, eye muscles, or extremities.
Wound or skin diphtheria occurs chiefly in the tropics, although cases have also been described in temperate climates among alcoholic, homeless individuals, and other impoverished groups. A membrane may form on an infected wound that fails to heal. However, absorption of toxin is usually slight and the systemic effects negligible. The small amount of toxin that is absorbed during skin infection promotes development of antitoxin antibodies. The “virulence” of diphtheria bacilli is attributable to their capacity for establishing infection, growing rapidly, and then quickly elaborating toxin that is effectively absorbed. C diphtheriae does not need to be toxigenic to establish localized infection—in the nasopharynx or skin, for example—but nontoxigenic strains do not yield the localized or systemic toxic effects. C diphtheriae does not typically invade deep tissues and practically never enters the bloodstream. However, notably over the last two decades, reports of invasive infections such as endocarditis and septicemia due to nontoxigenic C diphtheriae have increased.
When diphtheritic inflammation begins in the respiratory tract, sore throat and low-grade fever usually develop. Prostration and dyspnea soon follow because of the obstruction caused by the membrane. This obstruction may even cause suffocation if not promptly relieved by intubation or tracheostomy. Irregularities of cardiac rhythm indicate damage to the heart. Later, there may be difficulties with vision, speech, swallowing, or movement of the arms or legs. All of these manifestations tend to subside spontaneously.
In general, var gravis tends to produce more severe disease than var mitis, but similar illness can be produced by all types.
These serve to confirm the clinical impression and are of epidemiologic significance. Note: Specific treatment must never be delayed for laboratory reports if the clinical picture is strongly suggestive of diphtheria. Physicians should notify the clinical laboratory before collecting or submitting samples for culture.
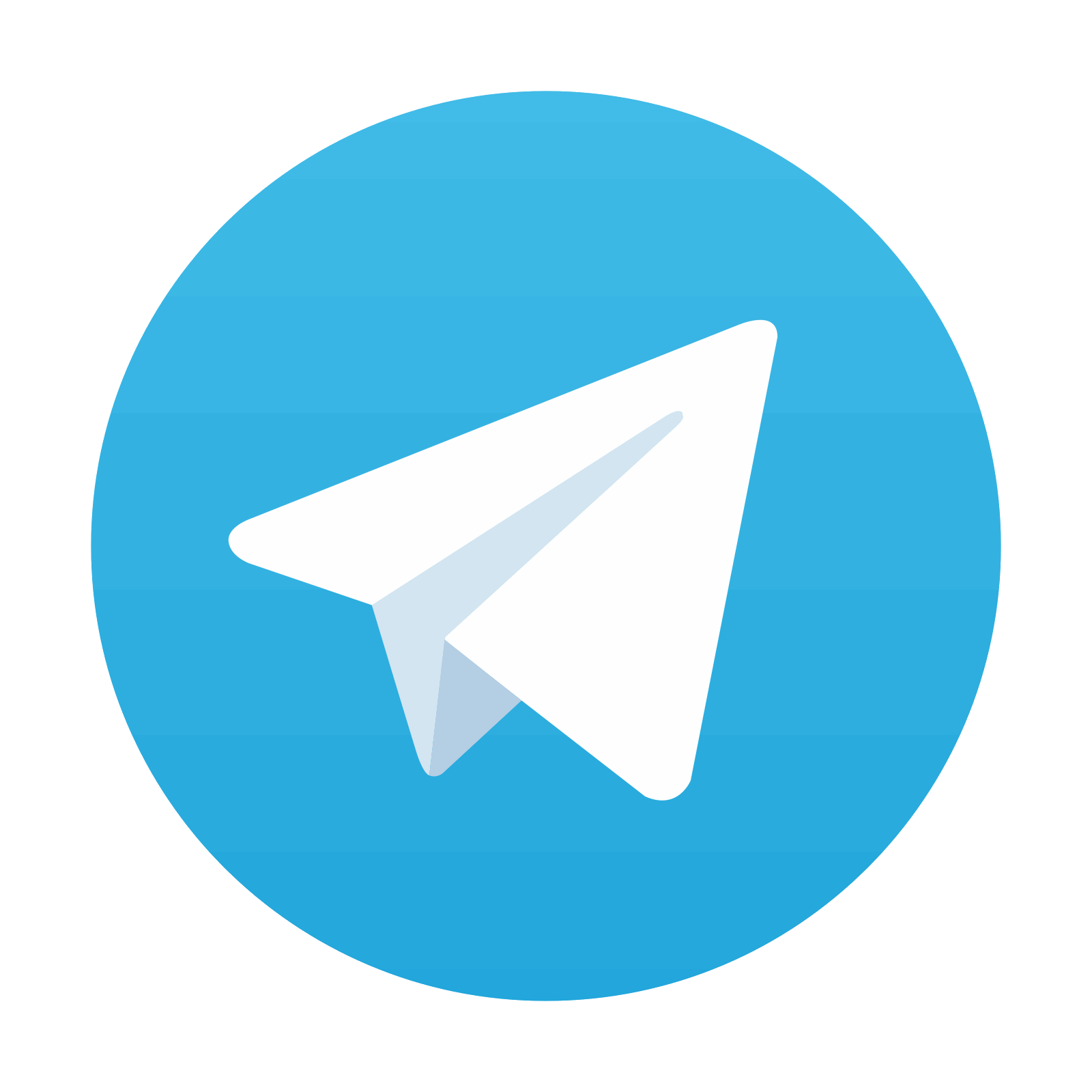
Stay updated, free articles. Join our Telegram channel

Full access? Get Clinical Tree
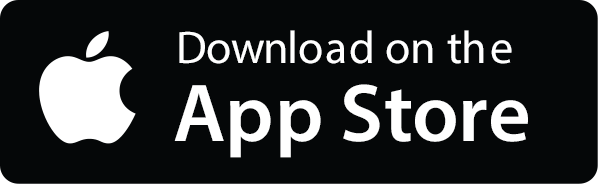
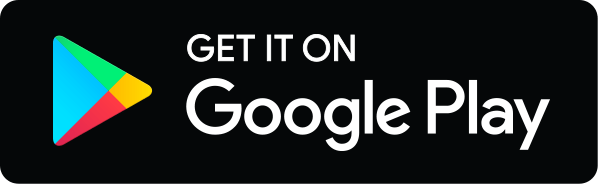