Sampling technique
Site(s)
accessible
Staging procedure
Real-time imaging and sampling
Complication risk
Specimen(s) attainable
Wang
Central
Yes
No
+
FNA and biopsy
Radial EBUS
Central and peripheral
Yesa
No
+
FNA
Convex EBUS
Central
Yes
Yes
+
FNA
CT
Central and peripheral
No
+/–
++
FNA and biopsy
Navigational
Central and peripheral
No
+/–
+
FNA and biopsy
The sampling targets in the chest can be roughly divided into central lesions and peripheral lesions. Over 70 % of patients with non-small cell lung cancer (NSCLC) will have nodal involvement at the time of their initial presentation [1]; therefore, many patients will have both peripheral and central targets to choose from. The various nonsurgical techniques for sampling available can be broadly divided into bronchoscopic and radiologic techniques, although some modalities combine both bronchoscopic and radiologic approaches to obtain pathologic specimens. This chapter addresses three bronchoscopic techniques, including endobronchial ultrasound-guided transbronchial needle aspiration (EBUS-TBNA) using convex probe technology (CP-EBUS), endobronchial ultrasound-guided sampling of peripheral nodules using radial probe technology (RP-EBUS), and electromagnetic navigational bronchoscopy (ENB). It also discusses CT-guided techniques for the sampling of parenchymal lung nodules, including CT-guided needle aspiration (CTNA) and CT-guided biopsy (CTNB).
In this chapter, “sampling” refers to the group of procedures available to clinicians for tissue diagnosis in suspected thoracic malignancy. Physicians and patients alike use the word “biopsy” when referring to these procedures. While the latter term may be useful in discussing various options with patients, it is incorrectly applied to brushings and fine-needle aspirates which result in a cytologic sample and not a histologic one. The distinction is an important one for the clinician; it is important to understand the kind of information obtainable from the samples derived from the different procedures. An excellent example would be the case of pulmonary lymphoma. Cytologic sampling with EBUS-TBNA combined with flow cytometry may establish a diagnosis of lymphoma, but the added information obtained from histologic sample, which has greater preservation of nodal architecture than a cytologic sample, may be useful for guiding treatment decisions. Knowledge of these differences may guide procedural choices in certain clinical circumstances.
General Principles of Lung Cancer Management Pertaining to Pathologic Sampling
Multiple guidelines exist to aid in clinical decision-making for the care of a patient with suspected lung cancer. For instance, the American College of Radiology recently proposed the Lung Imaging Reporting and Data System to standardize management of lung nodules identified on screening based on the size of the nodule, presence of a solid component, and growth characteristics [2]. Once the decision to sample a target has been made, the optimal modality for sampling has to be determined. Characteristics of the lesion and clinical features of the case must be factored into an analysis of the risks and benefits of each potential approach. Some techniques are more suitable for lesions with particular characteristics, and many factors, including the location, size, and differential diagnosis, must be taken into account when choosing a sampling modality. It is important for the clinician to understand the sensitivity and the limitations of the available sampling techniques, particularly when choosing between them.
Multiple approaches may yield the correct pathologic diagnosis, but clinicians must look at the broad context of each individual’s current and future care needs with respect to diagnosis, staging, and future treatment. The American College of Chest Physicians (ACCP) recently updated its guidelines for clinicians who deal with lung cancer (Diagnosis and Management of Lung Cancer, 3rd ed: American College of Chest Physicians Evidence-Based Clinical Practice Guidelines). In the article entitled “Establishing the Diagnosis of Lung Cancer,” Rivera and colleagues [3] outline the basic principles that should guide clinicians in their decision-making with respect to the selection of various diagnostic interventions. The clinician should strive to establish a pathologic diagnosis in the safest and least invasive way possible. Suspected solitary metastases should be confirmed pathologically. In patients with radiographic evidence for multiple metastatic foci in which pathologic sampling of extrathoracic disease would be problematic or difficult, sampling of the primary focus of intrathoracic disease is appropriate. Suspected malignant effusions should be confirmed pathologically. For patients with suspected mediastinal involvement and no evidence on imaging for extrathoracic disease, the guideline emphasizes that the diagnosis should be established by the “least invasive and safest method,” and bronchoscopy with TBNA, EBUS-TBNA, esophageal ultrasound-guided TBNA (EUS-TBNA), and transthoracic needle aspiration (TTNA) are listed as options.
An important consideration in undiagnosed patients is minimizing the number of procedures they undergo during the course of their management, seeking wherever possible to perform staging and diagnosis in a single step. It may be tempting to perform a CT-guided biopsy of a large peripheral mass, but if this patient is later found to have suspicious N3 nodes on a subsequent PET and requires a subsequent EBUS-TBNA to pathologically establish nodal involvement, the patient may have undergone an unnecessary intervention. This principle requires that full radiographic staging be undertaken before deciding which intervention is optimal. Patients are often very anxious and want a definitive diagnosis as soon as possible, but in the majority of cases, it is far better to have the right information than to do something right away.
An important distinction between the diagnosis and staging of NSCLC and small cell lung cancer (SCLC) should be mentioned. In NSCLC, radiographic staging should guide pathologic sampling, so that the patient’s true stage is established definitively to inform prognosis and guide therapy. In SCLC, which can often be suspected radiographically in a finding of bulky, central mediastinal tumor on imaging, the diagnosis can be established by sampling whichever site using whatever intervention is easiest and safest; staging of the disease is then determined radiographically [3]. In simplest terms, in NSCLC, radiology dictates pathologic sampling to establish a stage, whereas in SCLC, staging is usually radiographic.
Sampling Central Lesions
In patients with central targets, either masses or adenopathy in the mediastinum or parenchymal masses adjacent to the central airways from the trachea to the hila, bronchoscopic sampling is a consideration. “Blind” techniques for transbronchial needle aspiration (TBNA) of mediastinal lymph nodes were developed by Wang and colleagues [4, 5] at John Hopkins over 30 years ago. Yield and safety of this technique are limited by the blind nature of the procedure, but in expert hands, it is a safe and effective tool. Wang reported an overall diagnostic yield for malignancy of 85 %. Sensitivities for the procedure have been reported ranging from 39 to 89 % [6]. Prior to the development of EBUS-TBNA, the technique was usually employed only for large subcarinal (station 7) and precarinal (station 4R) lymph nodes and was, for the most part, performed routinely only by small percentage of pulmonologists [7]. Although newer technologies have largely supplanted blind TBNA, Wang and others [4, 5] strongly advocate that this procedure still has a role to play in the diagnosis and staging of lung cancer, citing its benefits as minimal discomfort, decreased cost, reduced risk, and widespread availability.
Radial and Convex Probe EBUS
Radial ultrasound was first developed for the field of gastroenterology in the 1980s and was adapted to bronchoscopy as more miniaturized components were developed in the 1990s. Modern radial probe endobronchial ultrasound (RP-EBUS) (Olympus) utilizes an ultrasound processor and a reusable 20 MHz ultrasound probe that is inserted through the working channel of a standard bronchoscope with a minimum working channel diameter of 2.0 mm. The radial probe uses mechanical radial scanning from a rotating transducer which, when placed in contact with the airway wall, generates a 360° B-Mode Doppler image of the layers of the bronchial wall and deeper structures adjacent to the airway. The image produced is one perpendicular to the long axis of the probe. The 20 MHz frequency allows for high resolution of the separate layers of the airway wall while providing visualization to a depth of approximately 5 cm [8]. For larger, more central airways, the probe can be covered in a sheath which has water-filled balloon at the distal tip. This can be used to facilitate circumferential contact with the airway wall for 360° imaging, allowing for imaging of the central airways and airway wall as well as central peribronchial structures such as mediastinal lymph nodes and central parenchymal lesions. The probe’s small diameter (1.4 mm at the distal tip) allows for probe access to areas of the lung periphery beyond the wedge position of the bronchoscope. It is this feature which allows it to be of use in localizing peripheral lung nodules for pathologic sampling, as will be discussed later.
Early reports of performance characteristics of this tool as a means of guiding conventional TBNA of mediastinal lymph nodes suggested that in expert hands, RP-EBUS guidance improved the overall yield of TBNA over the conventional techniques. Diagnostic yield for TBNA with RP-EBUS guidance has been shown to be superior to blind TBNA, although this comparison is colored by the fact that many studies of blind TBNA did not employ rapid on-site evaluation (ROSE) [6]. In a prospective randomized trial in 200 patients, Herth and colleagues [9] reported an overall yield of 80 % for RP-EBUS-guided TBNA versus 71 % with standard TBNA (p < 0.05). The superiority of RP-EBUS was derived from improved yield in nodal stations other than the subcarinal station 7; at station 7, no significant difference was seen [9]. The main disadvantage of the radial probe EBUS is that real-time pathologic sampling is not possible. The probe must be withdrawn from the working channel of the bronchoscope in order to allow for the insertion of a brush, needle, or forceps. This technique has the same final limitation of blind TBNA: after identification of a target lesion, the probe is removed, and the subsequent needle stick remains a blind procedure. The probe can be deployed inside a 2.0 mm diameter guide sheath, which can be left in place after ultrasound localization of a target, facilitating placement of a sampling tool and allowing for multiple passes at an identified target. Other disadvantages of the radial probe EBUS are its lack of color Doppler visualization of blood flow for the identification of vessels, as is possible with convex probe technology, and mechanical frailty of the probes. Although reusable, the delicate radial EBUS probes have a limited lifetime of up to 75 uses when handled with care [10].
Convex Probe EBUS
Despite its demonstrated superiority over conventional, blind TBNA for sampling central targets, RP-EBUS did not gain widespread use because of the development and introduction of curvilinear or convex probe ultrasound (CP-EBUS) technology. CP-EBUS represents an advance over radial probe technology in that it permits real-time pathologic sampling during ultrasound imaging. The convex probe technology uses a series of ultrasound transducers arranged in a curvilinear pattern at the distal end of a dedicated CP-EBUS bronchoscope. Multiple manufacturers of bronchoscopic systems currently offer a CP-EBUS platform. On the Olympus EBUS bronchoscope, the most widely used system, the curvilinear array provides a 60° field of view which is parallel to the long axis of the bronchoscope. The convex EBUS probe uses multiple quad-frequencies of 5, 7.5, 10, and 12 MHz, allowing for a depth of penetration of the ultrasound image to 5 cm, although the resolution is insufficient to differentiate the layers of the airway wall. The EBUS convex probe scope has a working channel with a diameter of 2.2 mm which terminates just proximal to the ultrasound probe head and which is angulated distally 35°, allowing the TBNA needle to pass over the probe in the plane of the ultrasound image. This permits real-time TBNA, with visualization of the needle in the target structure, representing a significant advantage over RP-EBUS. Other advantages include the optional color Doppler visualization of blood flow for the identification of vessels.
There are several disadvantages of the EBUS convex probe bronchoscope. The ultrasound transducer in the tip of the bronchoscope is distal to the light source and viewing port, and the direction of view is angulated 35° forward, parallel to the vector of the needle as it exits the scope, in contrast to the head-on field of view of standard bronchoscopy. This obliquely angulated view, which does not include the ultrasound transducer in the field, can make learning to use the EBUS convex probe scope difficult and perhaps raises the risks of traumatic injury to airway structures during bronchoscopy. Passage of the scope through the vocal cords can be particularly difficult and should be done with extreme care, as vocal cord injuries have been reported [11].
The EBUS convex probe bronchoscope is also larger than standard bronchoscopes, with an outer diameter of 6.9 mm. For this reason, EBUS convex probe bronchoscopy is done via the oral route, and airways smaller than 6.9 mm cannot be accessed. The size of the scope typically permits EBUS-TBNA of hilar nodal stations 11 and 12, however, as noted below [8]. The scope has a maximum flexion range (angulation up) of 120° and a maximum extension range (angulation down) of 90°. With the needle in the working channel, some degree of flexion is lost, and directing the scope into apically directed segments can be challenging. Airway wall contact can be facilitated by using a water-fillable disposable balloon which fits over the transducer head. This can be particularly useful in the trachea and the mainstem bronchi, where contact with the wall can be made difficult by limits in scope flexion, particularly with the needle and sheath in place, and by the irregular contours of the tracheal rings.
Another disadvantage of the EBUS convex probe bronchoscope is its lack of a video processor. The apparatus of the ultrasound transducer occupies space in the distal end of the bronchoscope which normally houses the video processor in a modern video bronchoscope. An EBUS scope utilizes older fiberoptic technology which has lesser image quality. The size of the scope, which limits access to more distal airways, along with the fact that the field of view is both obliquely angled and of lesser quality than a standard scope, mandates the need for a full inspection with a standard scope, before or after the EBUS bronchoscopy, in those patients with suspected NSCLC who are yet unstaged, to look for endobronchial disease or other foci of neoplasm. With the convex probe EBUS bronchoscope, sampling can be done of airway-adjacent parenchymal lesions and upper paratracheal (2 L and 2R), lower paratracheal (4 L and 4R), subcarinal (7), hilar (10 L and 10R), interlobar (11 L and 11R), and lobar (12 L and 12R) nodal stations. The prevascular/retrotracheal (3), subaortic (5), para-aortic (6), paraesophageal (8), and pulmonary ligament (9) nodal stations are not accessible via the tracheobronchial tree with the CP-EBUS [8]. Studies have shown that EBUS-TBNA using convex probe technology can be used in combination with endoscopic esophageal ultrasonography (EUS) to access the additional stations at 8 and 9, and some authors have used the CP-EBUS itself in the esophagus for this purpose [12].
The convex probe EBUS bronchoscope uses disposable needles available from multiple manufacturers. The needles are designed to be anchored to the bronchoscope and have an inner stylet, a movable sheath, and a stopping mechanism that limits needle travel to a fixed distance. The inner stylet serves to minimize the chances that a plug of bronchial wall will occlude the distal lumen of the needle. The needles have a movable sheath which can be deployed into the visual field of view prior to needle deployment to ensure that the needle leaves the working channel at the 35° angulation which will allow it to clear the ultrasound transducer and minimize the chance of a costly deployment into the transducer itself. The needles have a dimpled tip that serves to make them more echogenic, enhancing visualization in the ultrasound image. 21- and 22-gauge needles are now available. The larger inner diameter of the 21-gauge needle can provide larger samples at the expense of increased stiffness; this may allow for easier needle penetration but can limit scope mobility. Studies comparing the yield and complication rates of the 21- and 22-gauge needles have shown similar yields with slightly bloodier specimens obtained with the larger gauge [13, 14]. Use of the 21-gauge needle has been shown to result in fewer needle passes per aspirated node, and some have suggested that it may be superior with regard to better preservation of histologic architecture as well as quantity of tissue obtained [14]. The latter may have important ramifications when it comes to having adequate tissue for molecular testing in NSCLC, although data on this point is lacking.
A thorough ultrasonographic survey of the mediastinum at the time of CP-EBUS-TBNA is essential. Multiple studies of the performance characteristics of CP-EBUS-TBNA have identified PET-negative lymph nodes which were found to have malignant invasion on TBNA. Herth and colleagues showed that CP-EBUS can identify micrometastasis in patients with lung cancer and a radiographically normal mediastinum [15]. Although the percentage of cases in which CP-EBUS-TBNA upstages patients from their radiographic staging is small, they do occur, and these cases highlight the need for a full ultrasound assessment of accessible nodes and a full sampling from N3 to N2 to N1 nodes, irrespective of PET status, whenever possible in unstaged patients. In certain circumstances, however, targeted sampling is sufficient. In patients with radiographic stage 4 disease and difficult to access metastases, for example, a targeted EBUS-TBNA to establish a diagnosis may be wholly appropriate [3].
Target identification should begin before the procedure, with a careful analysis of the imaging. During the actual procedure, target identification with ultrasound should be done before the needle is inserted into the bronchoscope. The needle/sheath apparatus is then inserted and locked into place, and the sheath is deployed into the field of view and locked into place, ensuring that the needle will leave the bronchoscope at the proper angle, missing the transducer. After re-identifying the target lesion, the distance to the target (i.e., the distance of desired needle deployment) must be estimated or calculated using x and y axis centimeter markings on the view screen and the Pythagorean theorem. The stop mechanism on the needle handle is then set to this distance. Color Doppler visualization can be used to determine if blood vessels lie in the needle path. A variety of needle deployment techniques and sample collection techniques have been described. Firm deployment of the needle with the inner stylet in place can facilitate smooth needle penetration through the bronchial wall. Pitfalls at this stage often involve obstructing tracheal or bronchial rings, which can necessitate scope repositioning. After needle deployment, agitation of the stylet, ensuring that it is pushed as far distally as possible, can help to discharge a bronchial plug if one has been collected. Some operators use a suction technique, with suction ranging up to negative 20 cc, while others use no suction. A locking suction syringe is included with the Olympus EBUS needle package. The needle is then pushed in and pulled out across the span of the target, with care taken not to pull the tip of the needle out of the tissue, which could result in aspiration of the specimen into the suction syringe. Some operators use as many as 10–15 passes across a target lesion. Suction, if used, is then turned off, the needle is pulled fully into the sheath, the sheath is retracted, and the needle is removed from the bronchoscope.
It is important for the bronchoscopist to spend a moment to focus on the patient immediately after the TBNA. The puncture site should be visually observed for hemostasis. The puncture site should also be inspected for its location with respect to the desired target determined by careful examination of the imaging; was the intended target truly sampled? Precision in this regard is critical to prevent inadvertent upstaging or downstaging, as could be the case if a 10R hilar node was sampled instead of an intended nearby mediastinal 4R lymph node.
Although institutional protocol or physician preference may drive decisions regarding the type of sedation used for EBUS-TBNA, the procedure can be performed with either general anesthesia (GA), anesthesia-monitored deep sedation, or with topical anesthetic agents and moderate sedation. Results from the American College of Chest Physicians Quality Improvement Registry, Education, and Evaluation (AQuIRE) Registry, a 6-center, prospective study involving over 1,200 patients published in 2013, showed that using GA or deep sedation for EBUS-TBNA has the potential to improve patient comfort and results in the sampling of a greater number of nodal stations, possibly allowing for (or reflective of) a more systematic or thorough sampling of thoracic nodes [16, 17]. Some studies have shown that use of moderate sedation resulted in lower overall procedural yield [16]. In the AQuIRE Registry data set, the use of GA did not affect complication rates for the procedure, but it increased procedure time and the likelihood of a need for escalation of care post procedure [18]. EBUS-TBNA done with moderate sedation has the advantages of not requiring an operating room or anesthesiologist, which could lower overall procedural cost.
Performance Characteristics of EBUS-TBNA
Until CP-EBUS-TBNA became widely available, surgical mediastinoscopy was the procedure of choice for the sampling of mediastinal lymph nodes to establish a diagnosis and/or to stage the disease in patients with suspected stage II or stage III NSCLC. Multiple studies throughout the early years of EBUS-TBNA have reported similar or superior sensitivity and specificity to surgical mediastinoscopy. Adams and colleagues performed a meta-analysis of the CP-EBUS-TBNA studies published through 2008, finding a pooled specificity of 1.00 (95 % CI 0.92 to 1.00) and a pooled sensitivity of 0.88 (95 % CI 0.79 to 0.94) [19]. Gu and colleagues performed a meta-analysis which included a total of 11 studies with 1,299 patients, finding that EBUS-TBNA had a pooled sensitivity of 0.93 (95 % CI, 0.91–0.94) and a pooled specificity of 1.00 (95 % CI, 0.99–1.00) [20]. Yasufuku and colleagues published results of a prospective trial in which 159 patients underwent CP-EBUS-TBNA followed by mediastinoscopy under GA. They showed CP-EBUS-TBNA to have a sensitivity, negative predictive value, and accuracy of 81 %, 91 %, and 93 % respectively, compared to 79 %, 90 %, and 93 % for surgical mediastinoscopy [21]. The potential advantages of CP-EBUS over mediastinoscopy go beyond issues of sensitivity and negative predictive value. Whereas mediastinoscopy requires general anesthesia, EBUS can be performed with either general anesthesia, anesthesia-monitored deep sedation, or with topical anesthetic agents and moderate sedation. Lung cancer staging and diagnosis using EBUS-TBNA results in lower overall procedural risk compared to mediastinoscopy [22] and can result in lower overall healthcare costs [23, 24]. As noted above, EBUS-TBNA has a greater range of nodal stations that can be sampled compared to mediastinoscopy.
Expert interventional pulmonologists and thoracic surgeons performed the majority of published studies on the sensitivity of EBUS-TBNA for lung cancer, and the majority of the studies were conducted exclusively in patients under GA. Even in expert hands, procedural yield can vary greatly from center to center. In the AQuIRE registry, which included 6 hospitals, diagnostic yield (with yield defined as TBNA providing a specific diagnosis) ranged from 37 % to 54 % [17]. The performance characteristics of EBUS as a tool in widespread clinical use are not well known, and some operators may struggle to replicate the results seen in clinical trials.
Complications can occur with CP-EBUS-TBNA as with any bronchoscopy. As noted, there are multiple features of the CP-EBUS bronchoscope and the CP-EBUS-TBNA technique which make it more challenging to master than standard bronchoscopy. A significant majority of the published studies examining the performance characteristics of CP-EBUS-TBNA report no complications with the procedure. However, vocal cord injury [25], pneumothorax [26], pneumomediastinum [6], mediastinitis [27, 28], empyema [29], nonfatal hemorrhage [30], and fatal hemorrhage [31] have been published or presented. Despite these, the overall complication rate for CP-EBUS-TBNA is indeed low. The AQuIRE Registry examined complication rates in 1,317 cases performed in 6 centers by 12 clinicians over a 19-month period. They found an overall complication rate of 1.44 % (95 % confidence interval, 0.87–2.24 %). Sustained hypoxia (0.3 %), pneumothorax (0.2 % if no concurrent transbronchial biopsy was performed), bleeding (0.2 %), and respiratory failure (0.2 %) were all seen. One death occurred in the cohort, resulting from bleeding following an endobronchial biopsy done during the procedure and not from the TBNA [18].
The complication rates for EBUS-TBNA in widespread clinical use are not well known but are also likely low. Asano and colleagues published the results of a nationwide survey of EBUS-TBNA in Japan, where the procedure is widely available, pooling data from 210 centers that did 7,345 cases over an 18-month period. They reported a complication rate of 1.23 % (95 % confidence interval, 0.97–1.48 %), one procedure-related death (from a cerebrovascular hemorrhage), and procedure-related infection rate of 0.19 %, including cases of mediastinitis, pneumonia, pericarditis, cyst infection, and sepsis [32]. The reported procedure-related infection in the Japanese study, which was not seen in the AQuIRE Registry data, was likely a result of the fact that the latter study limited complications to events occurring within 24 h of the procedure.
The possibility of inappropriate or inadequate staging by CP-EBUS when done by an inexperienced operator is another concern, however. The potential for erroneously upstaging or downstaging a patient with NSCLC has been cited by some as a reason to recommend that a minimum of 50 cases be proctored before a bronchoscopist is considered to have reached an appropriate level of competency with the procedure [33].
There are several major limitations to EBUS-TBNA which have critical clinical implications and important implications for the cytopathologist. Nondiagnostic aspirations are common and can occur for multiple reasons. Most studies of EBUS-TBNA classify a lymph node aspirate as nondiagnostic if it contains neither malignant cells nor lymphocytes. Rates of nondiagnostic EBUS-TBNA range from 4 % to 23 % across published series. These rates describe the final result of a patient’s complete procedure, which most often involves multiple aspirations of a target lesion. When rapid on-site evaluation (ROSE) is employed, multiple aspirations of a target are often necessary before a diagnostic specimen is obtained; therefore, the nondiagnostic rate of individual aspiration attempts is lower. For this reason, when ROSE is not available, it is suggested that 3 aspirations be performed at each target to optimize yield [34, 35].
The transbronchial approach results in contamination of the cytologic specimen with bronchial cells; in one study of CP-EBUS-TBNA, bronchial cells were seen in up to 80 % of specimens [36]. A finding of only bronchial cells in the aspirated specimen is not uncommon with EBUS-TBNA. This occurs when the hollow bore needle picks up a bronchial plug as it passes through the airway wall, despite the presence of the stylet. The bronchial plug can occlude the needle tip, preventing successful aspiration of the target. Even if it is discharged by pushing out the stylet, it can be aspirated back into the needle after suction is applied. Certain characteristics of the tumor itself can contribute to a nondiagnostic finding. Necrotic tumors may contain few cells, for example.
One key to optimizing yield and to optimizing the efficiency of EBUS-TBNA lies with ROSE of the aspirated specimens. In a large meta-analysis, ROSE was shown to increase the sensitivity of EBUS-TBNA from 80 % to 88 % while decreasing procedure times [37]. Most (but not all) studies showing improved sensitivity and negative predictive values for EBUS-TBNA over mediastinoscopy used ROSE. Resource limitations are a reality in clinical medicine, and ROSE is not available in every institution, but the bronchoscopist who performs EBUS-TBNA should strongly advocate for it in order to optimize the benefits to patients.
CP-EBUS-TBNA as Part of a Multidisciplinary, Multimodality Approach to Lung Cancer
With or without ROSE, the cytopathologist plays a critical role in the performance of the operator, providing feedback on the quality and quantity of samples obtained. Whether done by the bronchoscopist, bronchoscopy nurse, or cytopathologist on site, proper handling, triage, and processing of the aspirated sample is crucial to optimize the yield for immunohistochemical staining and full molecular analysis in the case of adenocarcinoma.
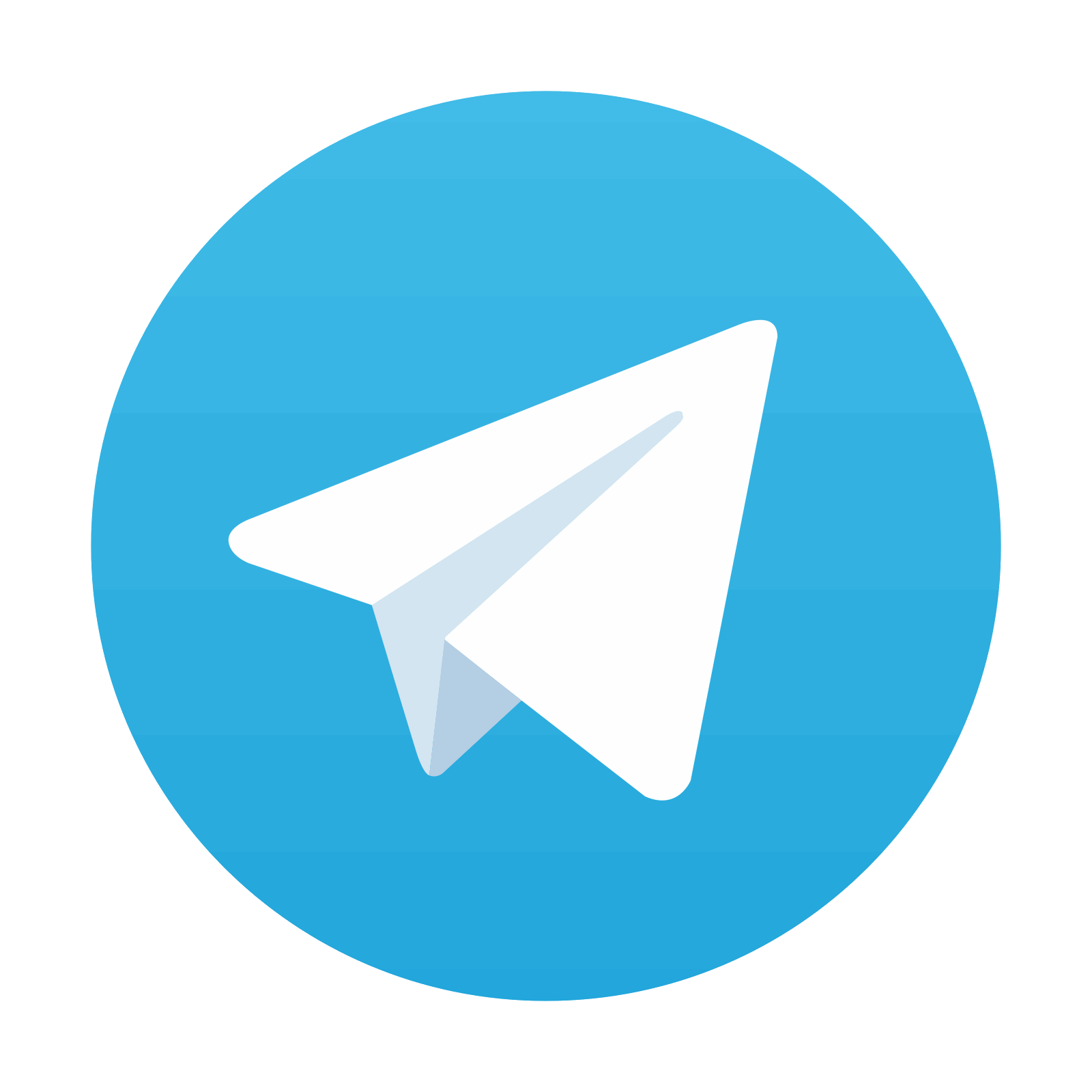
Stay updated, free articles. Join our Telegram channel

Full access? Get Clinical Tree
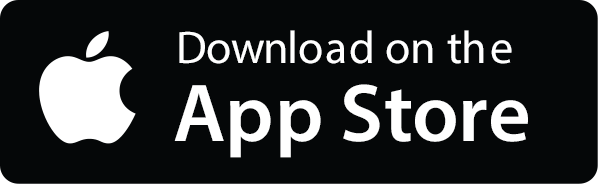
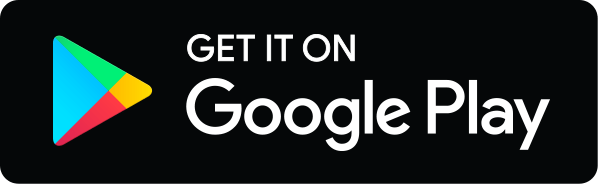