30.3.6.3 Acyclic and Cyclic S,S-Acetal S-Oxides and S,S′-Dioxides (Update 2016)
A. Ishii
General Introduction
Aldehyde and ketone S, S-acetal oxides and ketene S, S-acetal oxides are recognized as masked aldehydes or ketones, and ketenes, respectively, and they have been synthesized from various starting materials by nucleophilic substitution at the α-position of a sulfanyl group, by the reaction of carbanions stabilized by sulfanyl, sulfinyl, and sulfonyl groups with electrophiles, or by the oxidation of S, S-acetals. They are relatively easily hydrolyzed to the corresponding carbonyl compounds. Recent progress has been seen in reactions utilizing reactive sulfonium intermediates under Pummerer conditions as well as conjugate additions of nucleophiles or radicals to ketene S, S-acetals.
This chapter provides an update to the earlier Science of Synthesis publication on S, S-acetal oxides by K. Ogura (Section 30.3.6), and cross-references to sections in Ogura’s review are provided where appropriate. In addition, material on the synthesis of S, S-acetal S,S′-dioxides, which were not covered in the earlier work, is also presented.
30.3.6.3.1 Synthesis of Acyclic and Cyclic S,S-Acetal S-Oxides and S,S′-Dioxides
30.3.6.3.1.1 Method 1: Reactions of α-Sulfanyl α-Sulfinyl Carbanions
30.3.6.3.1.1.1 Variation 1: Monoalkylation with Alkyl or Hetaryl Halides, Epoxides, or Enones
The reaction of the carbanion 2 of methyl (methylsulfanyl)methyl sulfoxide (1) with electrophiles such as alkyl halides, 2-bromopyridines, epoxides, α,β-unsaturated carbonyl compounds, and allylic halides provides the corresponding monosubstituted S, S-acetal S-oxides 3 (▶ Scheme 1; see Section 30.3.6.1.8.1).[1–8]
This procedure has been employed to prepare the key synthetic intermediates for the synthesis of disaccharides (▶ Scheme 2).[9] The reaction of 1 with δ-triphenylmethoxy iodide 4 in the presence of sodium hydride as the base in tetrahydrofuran at 75 °C yields hydroxy S,S-acetal S-oxides 5 after deprotection of the triphenylmethyl group with camphorsulfonic acid. The sulfinyl group in 5 is activated by trifluoromethanesulfonic anhydride, leading to an intramolecular cyclization, which is followed by reaction with a glycosyl acceptor 6 to yield disaccharide 7. A reaction mechanism involving sulfenium and oxonium intermediates 8 and 9, respectively, has been proposed.
Scheme 2 Alkylation of an S, S-Acetal S-Oxide Followed by Sequential Intramolecular Cyclization and Glycosidation Activated by Trifluoromethanesulfonic Anhydride[9]

R1 | R2 | Yield (%) of 5 | Ratio (α/β)of 7 | Yield (%) of 7 | Ref |
OBn | H | 70 | 2:1 | 92 | [9] |
H | OBn | 80 | 3.4:1 | 88 | [9] |

30.3.6.3.1.1.2 Variation 2: Condensation with Carbonyl Compounds
As discussed in Section 30.3.6.1.8.3, methyl (methylsulfanyl)methyl sulfoxide (1) condenses with carbonyl compounds under basic conditions in a Knoevenagel-type condensation to yield (E)-ketene S, S-acetal S-oxides stereoselectively (E/Z >99:1). Triton B (benzyltrimethylammonium hydroxide) and alkali metal hydroxides (NaOH, KOH) are usually used as the base in tetrahydrofuran or without solvent at 60–70 °C.[10]
The syntheses of new examples of these products have been reported (▶ Scheme 3).[11,12] The 1-naphthyl derivative (E)-10 (Ar1 = 1-naphthyl) is synthesized in a good yield, but anthracene-9-carbaldehyde and 4-(trifluoromethyl)benzaldehyde give the corresponding ketene S, S-acetal S-oxides in low yields. Pentafluorobenzaldehyde does not yield the corresponding S, S-acetal S-oxide (E)-10 (Ar1 = C6F5).
Ar1 | Base | Conditions | Yield (%) | Ref |
Ph | Triton B | THF, reflux, 4 h | 91 | [10] |
Ph | NaOH | no solvent, 70 °C, 1 h | 84 | [10] |
Ph | Triton B | THF,60 °C, 6 h | 91 | [11,12] |
4-ClC6H4 | NaOH | no solvent, 70 °C, 1 h | 70 | [10] |
4-ClC6H4 | Triton B | THF, 60 °C, 6 h | 89 | [11,12] |
4-MeOC6H4 | Triton B | THF, reflux, 53 h | 82 | [10] |
4-MeOC6H4 | Triton B | THF, 60 °C, 6 h | 79 | [11,12] |
3-thienyl | Triton B | THF, 60 °C, 6 h | 94 | [11,12] |
1-naphthyl | Triton B | THF, 60 °C, 6 h | 72 | [11,12] |
9-anthryl | Triton B | THF, 60 °C, 6 h | 21 | [11,12] |
4-F3CC6H4 | Triton B | THF, 60 °C, 6 h | 25 | [11,12] |
C6F5 | Triton B | THF, 60 °C, 6 h | 0 | [11,12] |
A related, alternative method to synthesize ketene S, S-acetal S-oxides is dehydration of alcohols prepared by the reaction of the anion of 1 with aromatic aldehydes. The resulting alcohols 11 are acetylated with acetic anhydride, giving acetoxy derivatives 12; this is followed by treatment with triethylamine to provide ketene S, S-acetal S-oxides 13 (▶ Scheme 4; see also Section 30.3.6.1.8.3).[13]
Aliphatic aldehydes, as well as aromatic aldehydes having electron-withdrawing trifluoromethyl or fluoro substituents, have been used successfully in this method, albeit with low or no stereoselectivity and in low to moderate yields (▶ Scheme 5).[11,12] Alkoxide intermediates formed in the first step are acetylated sequentially with acetic anhydride, and the resulting acetoxy derivatives 14 are treated with potassium tert-butoxide to yield the corresponding ketene S, S-acetals S-oxides 15.
R1 | Ratio (E/Z) | Yield (%) of 15 from 1 | Ref |
9-anthryl | >99:1 | 15 | [11] |
4-F3CC6H4 | 3:1 | 42 | [11] |
4-TBDMSOC6H4 | 4:1 | 70 | [11] |
Me | 9:1 | 69 | [11] |
Cy | 1.5:1 | 29 | [11] |
C6F5 | 1:1 | 38 | [11] |
30.3.6.3.1.2 Method 2: Oxidation Reactions
30.3.6.3.1.2.1 Variation 1: Oxidation of S,S-Acetals
S,S-Acetal S-oxides can be prepared by the oxidation of S, S-acetals with an equimolar amount of common oxidants such as hydrogen peroxide with additives, sodium periodate, 3-chloroperoxybenzoic acid, tert-butyl hydroperoxide/titanocene dichloride, and oxygen/flavin as well as photosensitized oxidation (see Section 30.3.6.1.10.1).
Chiral S, S-acetal S-oxides have synthetic utility, and asymmetric oxidation of S, S-acetals has been investigated extensively since the study using sodium periodate together with a catalytic amount of bovine serum albumin described in Section 30.3.6.1.10.1. That earlier review also discusses the asymmetric oxidation of cyclic S, S-acetals performed using Sharpless or modified Sharpless conditions and, in particular, S, S-acetals having directing groups such as ester, acyl, hydroxy, and alkoxy groups lead to high asymmetric induction. In addition, asymmetric oxidations employing chiral oxidants, enzymes, and whole-cells have been reported.
More recently, a detailed study on the regioselectivity and stereoselectivity of the oxidation of bis(S,S-acetal) 16 with 3-chloroperoxybenzoic acid has been reported (▶ Scheme 6).[14] The oxidation with 1 equivalent of 3-chloroperoxybenzoic acid in dichloromethane gives monoxide 17 in 88% yield. The second oxidation of 17 yields mainly meta-isomers (cis–meta–18 and trans–meta–18) over para-isomers (cis–para–18 and trans–para–18) and ortho-isomers; the1H NMR analysis revealed that the ratios of meta–18/para–18 were >9:1 for both cis– and trans-isomers. The meta/para(ortho) selectively was explained in terms of the electron-withdrawing effect of the first sulfinyl group decreasing the electron density on the ortho– and para-sulfur atoms. Overall yields of 19% of the cis-isomers (cis–meta- 18 + cis–para–18) and 25% of the trans-isomers (trans–meta–18 + trans–para–18) were obtained.
A highly asymmetric oxidation of the parent and mono- and disubstituted 1,3-dithianes 19 has been achieved with the combination of chiral salalen–aluminum complex 20 (2 mol%) and 30% hydrogen peroxide in ethyl acetate to provide (S)-1,3-dithiane 1-oxides 21 (▶ Scheme 7).[15] Even in the case of the parent 1,3-dithiane, (S)-1,3-dithiane 1-oxide (19, R1 = R2 = H) is obtained in 70% yield with 95% ee, together with the corresponding (1S,3S)-trans-1,3-dioxide (15% yield, 99% ee). This asymmetric oxidation is also applicable to five-and seven-membered cyclic dithioacetals to provide (1S,2S)-trans-2-phenyl- and (1S,2S)-trans-2-tert-butyl-1,3-dithiolane 1-oxides and 2-phenyl-1,3-dithiepane 1-oxide with >99% ee. In the case of an acyclic S, S-acetal, bis(phenylsulfanyl)methane (PhSCH2SPh), the oxidation is slow and poorly enantioselective giving the corresponding mono-S-oxide [PhS(O)CH2SPh] in 8% yield with 64% ee.
Scheme 7 Asymmetric Oxidation of 1,3-Dithianes with Hydrogen Peroxide Catalyzed by a Chiral Salalen–Aluminum Complex[15]

R1 | R2 | ee (%) | Yield (%) | Ref |
Ph | H | 99 | 93 | [15] |
CH=CHPh | H | 99 | 95 | [15] |
C=CPh | H | >99 | 72 | [15] |
Bn | H | 99 | 92 | [15] |
(CH2)2Ph | H | 99 | 94 | [15] |
iPr | H | >99 | 98 | [15] |
cyclopropyl | H | 99 | 95 | [15] |
Cy | H | 99 | 98 | [15] |
t-Bu | H | >99 | 73 | [15] |
TMS | H | 99 | 91 | [15] |
Me | Me | 99 | 68 | [15] |
Ph | Me | >99 | 79 | [15] |
H | H | 95 | 70 | [15] |
(S)-1,3-Dithiane 1-Oxides 21; General Procedure:[15]
To a soln of salalen–aluminum complex 20 (3.6 mg, 2 mol%) in EtOAc (1.0 mL) was added 1,3-dithiane 19 (0.2 mmol). After addition of 0.067 M phosphate buffer (pH 7.4), the mixture was cooled to 10 °C and then 30% aq H2O2 (24.9 μL) was added. After being stirred for an appropriate time, the mixture was concentrated under reduced pressure and the residue was purified by chromatography (silica gel, hexane/EtOAc) to give the corresponding 1-oxide 21 as a diastereomeric mixture. The diastereomers were isolated by preparative TLC and the enantiomeric excesses were determined by chiral HPLC analysis.
30.3.6.3.1.2.2 Variation 2: Oxidation of Ketene S,S-Acetals
Monooxidation of ketene S, S-acetals (Section 30.3.6.1.10.3) is a direct method to synthesize ketene S, S-acetal S-oxides (see also Section 30.3.6.1.8.3).
The enantioselective dioxidation of ketene S, S-acetals 22 has been performed by Sharpless-type asymmetric oxidation employing (+)-diethyl tartrate [(+)-DET) (2 equiv)], titanium(IV) isopropoxide (0.5 equiv), and cumene hydroperoxide (4 equiv) to provide ketene S, S-acetal (R,R)-S,S′-dioxides 23 in good yields with very high enantiomeric excesses (▶ Scheme 8).[16] In the asymmetric oxidation, while the formation of monoxide 24 [(R/S) 93:7] was observed, meso-dioxide 25 was never isolated despite several attempts, which was explained in terms of the formation of a tight complex with titanium that made it difficult to isolate 25 even if it formed. Ketene S, S-acetal S-oxides and S, S′-dioxides can act as 2π components in [3 + 2] and [4 + 2] cycloadditions under high diastereocontrol (see ▶ Section 30.3.6.3.2.5).
Scheme 8 Asymmetric Oxidation of Ketene S, S-Acetals with Cumene Hydroperoxide Catalyzed by Titanium(IV) Isopropoxide/(+)-Diethyl Tartrate[16]

R1 | R2 | Time (h) | ee (%) | Yield (%) | Ref |
H | Bu | 5.5 | 98 | 65 | [16] |
H | Cy | 6 | >99 | 62 | [16] |
(CH2)5 | 22 | >99 | 75 | [16] | |
H | Ph | 24 | >99 | 73 | [16] |
H | 4-MeOC6H4 | 21 | >99 | 71 | [16] |
Me | Ph | 18 | >99 | 60 | [16] |

The stereoelectronic effects of sulfinyl groups in 2-benzylidene-1,3-dithiane oxides 27 – 30 on C—H coupling constants (JC–H) in neighboring CH2 groups (the Perlin effect) have been investigated.[17] Monoxide 27 is prepared in 81% yield by oxidation of 26 with 30% hydrogen peroxide in fresh molten phenol (▶ Scheme 9).

(1R,3R)-2-Cyclohexylidene-1,3-dithiane 1,3-Dioxide [23, R1, R2 =(CH2)5]; Typical Procedure:[16]
(+)-Diethyltartrate (0.34mL, 2.01 mmol) and Ti(OiPr)4 (0.15 mL, 0.50 mmol) were dissolved in CH2Cl2 (5 mL) at rt under N2 and stirred for 20 min. A soln of 2-cyclohexylidene-1,3-dithiane [22, R1, R2 = (CH2)5; 1 mmol] in CH2Cl2 (1.0 mL) was added to the mixture, which was then cooled to –40 °C and stirred for 1 h. Cumene hydroperoxide (80%; 0.76 mL, 4.01 mmol) was added, and the mixture was stirred at –40 °C for 10 min and then allowed to stand at –20 °C for 22 h. Distilled H2O (0.36 mL) was added and the mixture was allowed to warm to rt with stirring for 1 h. The mixture was filtered through a pad of Celite, which was washed well with CH2Cl2, and the solvent was removed under reduced pressure. Purification by column chromatography (silica gel, hexane/Et2O/EtOH 1:1:0, 0:1:0, and then 0:1:1) afforded a colorless solid; yield: 173 mg (75%); mp 159.5–161 °C (EtOH/hexane); [α]D22 –21.5 (c 1.0, CHCl3).
30.3.6.3.1.2.3 Variation 3: Oxidation of α-Sulfanyl Vinyl Sulfenates
The synthesis of ketene S, S-acetals 31 by a sequence of thioenolization and S-methylation of dithioesters has been reported (▶ Scheme 10).[18] This procedure was modified to synthesize ketene S, S-acetal S-oxides (▶ Scheme 11).[19] Dithioesters 32 are deprotonated with methyllithium in tetrahydrofuran at –78 °C and the resulting enethiolates are oxidized with N-sulfonyloxaziridine 33. Methyllithium is the optimal base since the use of amides such as lithium diisopropylamide leads to complex mixtures. The treatment of the resulting enesulfenate 34 with alkyl halides provides ketene S, S-acetal S-oxides 35. The Z/E ratio of 35 is dependent on the conditions for alkylation of enesulfenate 34, because Z-enesulfenate (Z)-34, which is favorably formed, reflecting the configuration of the enethiolates at –78 °C, comes to be in equilibrium with (E)-34 at higher temperatures. Thus, alkylation at –78 °C leads to the preferential formation of (Z)-35, and that at –15 °C results in the preferential formation of (E)-35.
R1 | R2 | X | Alkylation Conditions | Ratio (Z/E) of 35 | Yielda (%) of 35 | Ref |
H | Me | I | R2X (1.1 equiv), slow warming from –78 °C to rt | – | 79 | [19] |
H | Bn | Br | R2X (1.1 equiv), slow warming from –78 °C to rt | – | 56 | [19] |
Me | Me | I | R2X (5 equiv), –78 °C, 5 h | 76:24 | 61 | [19] |
Me | Me | I | slow warming from –78 to –15 °C, then R2X (1.1–2 equiv) | 13:87 | 80 | [19] |
Me | Me | I | R2X (1 equiv), rapid warming from –78 °C to rt | 54:46 | 76 | [19] |
Me | Bn | Br | slow warming from –78 to –15 °C, then R2X (1.1–2 equiv) | 22:78 | 66 | [19] |
Me | Et | I | slow warming from –78 to –15 °C, then R2X (1.1–2 equiv) | 15:85 | 76 | [19] |
Pr | Me | I | R2X (5 equiv), –78 °C, 5 h | 74:26 | 58 | [19] |
(CH2)7Me | Me | I | R2X (5 equiv), –78 °C, 5 h | 74:26 | 85 | [19] |
(CH2)7Me | Me | I | slow warming from –78 to –15 °C, then R2X (1.1–2 equiv) | 42:58 | 62 | [19] |
Ph | Me | I | R2X (5 equiv), –78 °C, 5 h | 58:42 | 26 | [19] |
a Yield from 32. |
Ketene S,S-Acetal S-Oxides 35; General Procedure:[19]
A soln of dithioester 32 (1.00 mmol) in anhyd THF (5 mL) was cooled to –78 °C and 1.6 M MeLi in Et2O (0.69 mL, 1.1 mmol) was added dropwise. Discoloration of the orange mixture was immediately observed. After the mixture had been stirred at –78 °C for 15 min, a soln of oxaziridine 33 (267 mg, 1.05 mmol) in anhyd THF (2 mL) was slowly added dropwise (exothermic reaction). The mixture was stirred at this temperature for 20 min and treated with the alkyl halide (1–5 equiv). The soln was stirred at –78 °C for 5 h and then hydrolyzed at this temperature with sat. aq NaCl (3 mL). The product was extracted with Et2O (3 ×) and the combined organic extracts were dried (MgSO4), and concentrated to dryness. Pentane/CH2Cl2 (7:1) was added to precipitate the benzenesulfonamide byproduct from the crude mixture. After filtration and concentration under reduced pressure, the resulting yellow oil was purified by column chromatography (silica gel, petroleum ether/EtOAc).
30.3.6.3.1.3 Method 3: Addition of S, S-Acetal S, S′-Dioxides to Carbonyl Compounds
The reaction of the anion generated from trans-1,3-dithiane 1,3-dioxide with aldehydes provides the corresponding alcohols 36 (▶ Scheme 12).[20–23] The adducts 36, after protection of the hydroxy group with dihydropyran, are converted into the corresponding thioesters (see ▶ Scheme 25, ▶ Section 30.3.6.3.2.2).
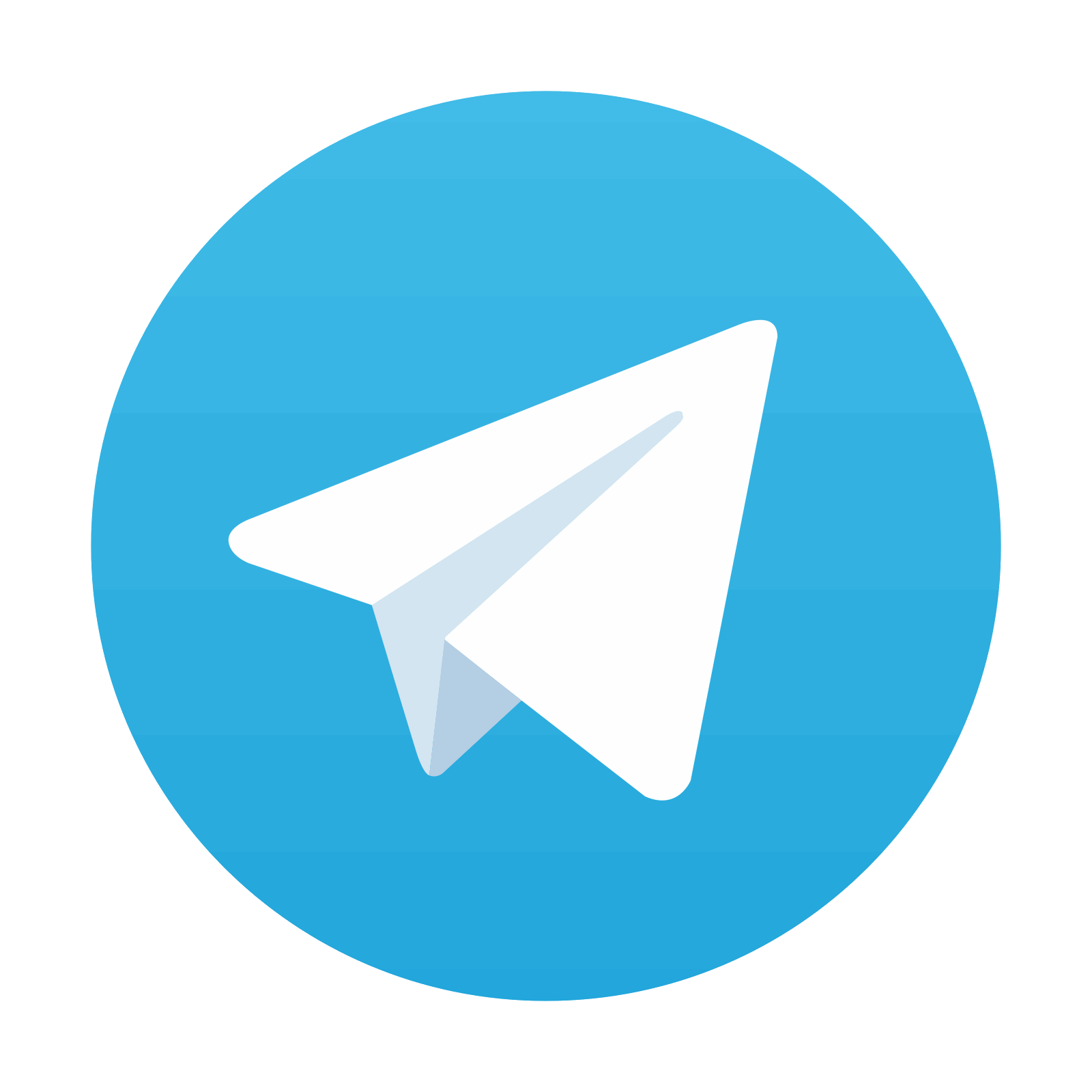
Stay updated, free articles. Join our Telegram channel

Full access? Get Clinical Tree
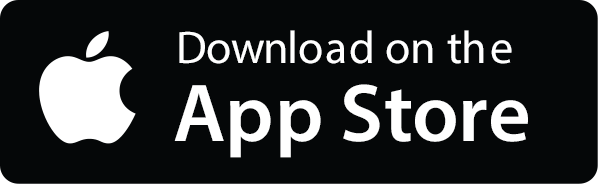
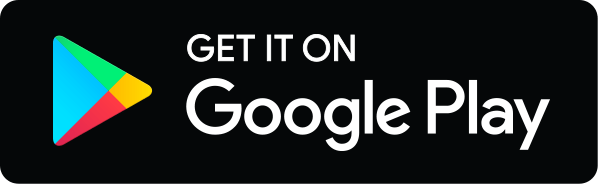