Biologic Scaffolds Composed of Extracellular Matrix as a Natural Material for Wound Healing
McGowan Institute for Regenerative Medicine, University of Pittsburgh, Pittsburgh, PA, USA
McGowan Institute for Regenerative Medicine, Department of Surgery, University of Pittsburgh, Pittsburgh, PA, USA
McGowan Institute for Regenerative Medicine, Department of Surgery, University of Pittsburgh, Pittsburgh, PA, USA
7.1 Introduction
The ideal substrate/scaffold for cells, tissues, and organs is that which exists in nature; that is, the extracellular matrix (ECM). The ECM represents the secreted product of resident cells and is a dynamic, living structure. Stated differently, the ECM is constantly changing in response to microenvironmental niche conditions such as pH, oxygen tension, nutrient availability, mechanical loading, and other external influences; a process aptly named dynamic reciprocity [1,2]. In addition, there are undoubtedly internal influences which affect the composition and ultrastructural organization of the matrix, such as the inherent genetic programming of resident cells, the influences of age, gender, and other endogenous factors. This ideal scaffold which we call the ECM is an extremely complex structure that is only partially understood. It is logical therefore, that a bio-inspired material is limited by the depth of our understanding of the composition, structure, and signaling mechanisms of the native matrix.
It is widely recognized that the ECM has a profound influence on cell differentiation, phenotype, and behavior [3–6]. Cell adhesion to matrix ligands triggers intracellular signaling pathways that regulate cell migration, cell cycle progression, and cell differentiation [7–9]. The transitional ECM in developing fetuses influences cell behavior through molecules such as tenascin-C, hyaluronic acid, and fibronectin [10]. Changes in ECM organization and mechanical properties affect cell phenotype [3]. Not only is our understanding of matrix structure–function relationships limited, but our attempts to mimic the ECM are wrought with such challenges. The technical limitations of reproducing all elements of the ECM, especially those which are dynamic and responsive to transitional external influences, are daunting.
Although a synthetic mimic of the ECM cannot be produced, individual components of the matrix such as collagen, fibronectin, laminin, and tenacin can be isolated and engineered to provide an instructive scaffold for tissue engineering and regenerative medicine applications. There currently exist many biologic scaffold materials composed of mammalian ECM (Table 7.1). Such naturally occurring materials are the subject of this chapter. An overview of these materials, which are typically regulated as surgical mesh medical devices by the Food and Drug Administration (FDA), is provided. In addition, the preparation of such materials will be briefly reviewed including the influence of various processing methods on clinical efficacy. Mechanisms by which the host responds to these materials is becoming increasingly clear and such mechanisms will be described herein.
Table 7.1 Clinically Available Products Composed of Extracellular Matrix (ECM)
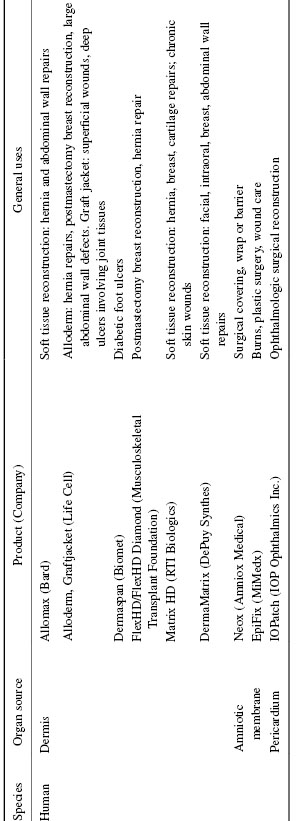
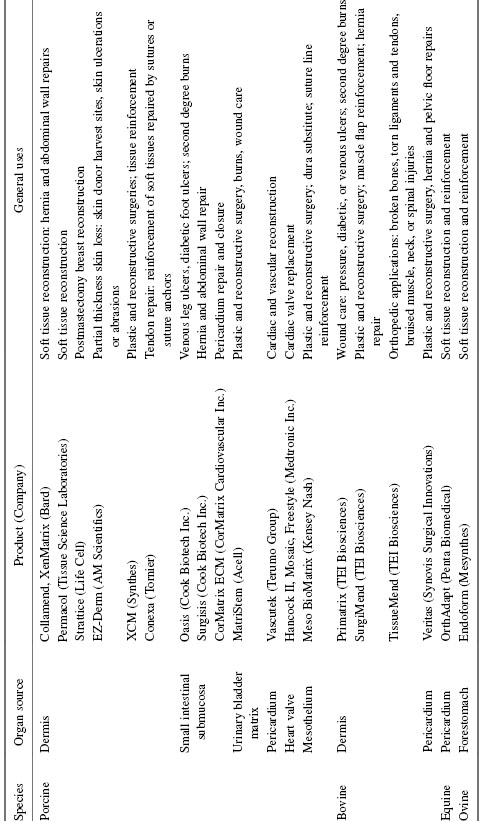
7.2 Products and Clinical Use of ECM
Scaffolds composed of biologic materials such as ECM, or components of ECM, are used in a variety of surgical applications. The advantages of biologic materials as compared with synthetic materials include a greater resistance to bacterial infection and a more constructive host healing response. However, biologic scaffold materials tend to be less strong than synthetic materials such as polypropylene, and are subject to biologic variability, which can affect mechanical and material properties to a small degree.
As can be seen in Table 7.1, the species from which biologic scaffold materials are harvested include: human, porcine, bovine, equine, and ovine. In addition, the tissues from which these materials are prepared vary widely and include small intestine, urinary bladder, dermis, pericardium, and fascia, among others. The wide variety of raw material sources for biologic scaffold materials suggests that there are favorable characteristics inherent within and conserved across all mammalian ECM. There are clear differences not only in the structure and composition of ECM derived from different species and different tissues, but more importantly, differences in the manufacturing processes can dramatically affect structure function relationships [11–13]. The manufacture of a biologic scaffold material requires decellularization of the source tissue while maintaining, as much as possible, the native structure and composition of the ECM [14–17]. Decellularization methods vary widely and are the subject of several reviews [11,14]. Chemical, enzymatic, and physical methods of decellularization are utilized to various degrees. The host response following subsequent implantation of the resulting ECM is directly related to the efficacy of decellularization of the source tissue [18,19]. Standard criteria for defining effective decellularization have been proposed [14], and include several different quantitative measures: the absence of visible nuclear material on histologic examination with H&E or DAPI staining, DNA remnants less than 50 ng/mg of dry weight, and remnant DNA fragments must be 200 base pairs or less. Commercially available products have a wide range of residual cellular material [20,21], and this cellular debris likely plays a critical role in the host response. There have been descriptions of excellent functional and constructive remodeling outcomes following the use of biologic scaffold materials in several surgical applications, including esophageal [22,23], musculoskeletal [24–28], and the lower urinary tract [29–33], among others [34–36]. However, there have also been reports of unfavorable proinflammatory reactions following the use of biologic scaffold materials [37–39]. These unfavorable reactions include serous fluid accumulation, redness and swelling, and mechanical failure [21,40,41]. It is likely that the disparate outcomes are related to processing (i.e., decellularization) methods.
The use of chemical cross-linking agents to provide strength to biologic materials is commonplace [42–45]. Although chemical cross-linking does indeed provide strength, such treatment transforms the naturally occurring, degradable material, into a nondegradable (or very slowly degradable) material, which elicits a foreign body response [19,38,46]. In addition, the inherent growth factors and bioactive cryptic peptides, which are produced during the degradation process, are substantially inhibited [47–50]. In the opinion of the authors, the use of chemically cross-linked ECM transforms an inductive biologic material into one that is similar to most nondegradable synthetic materials.
In addition to efficacy of decellularization and the use of chemical cross-linking agents, methods of terminal sterilization such as ethylene oxide, electron beam, and gamma irradiation can affect mechanical, physical, and biologic properties of these naturally occurring materials [51–56]. Lyophilization, vacuum pressing, and storage conditions can also play a notable role in remodeling outcomes [57–60]. In summary, there are numerous potential sources of variability in the preparation of biologic scaffold materials. The appropriate and optimal use of such materials for various clinical applications requires an understanding not only of the methods of preparation for each product, but also the mechanisms by which the host responds to these materials (discussed in more detail below).
7.3 Mechanisms of ECM Remodeling
Appropriately prepared biologic scaffolds composed of ECM have been shown to facilitate constructive and site-appropriate remodeling when implanted into in vivo injury sites both in preclinical animal models and human clinical applications. The new host tissue that forms is grossly, histologically, and functionally similar to the native tissue it is intended to replace, but does not perfectly recapitulate the native tissue structure and function. Site-specific remodeling has been shown in a variety of tissues, including esophagus [22,23], blood vessels [61,62], skin and body wall [25,63], urinary tract [29,30], skeletal muscle [24–28,64–67], tendon and ligaments [27,68,69], myocardium [70,71], and bone [72,73], among others. In addition to the intact two- or three-dimensional form of ECM scaffolds, such materials can be enzymatically digested, solubilized, and polymerized to form a hydrogel, a network of polymer chains in which water is the dispersion medium [74–80]. ECM hydrogels retain many components found in the native tissue [81]. Injectable, in situ polymerizing hydrogels have been used for biomedical applications such as drug delivery and/or targeted stem cell delivery. Their ability to be delivered by minimally invasive techniques, conform to an irregular shape, and maintain robust biologic activity, make hydrogels optimal for these applications [82].
The use of ECM scaffolds at least partially transforms the natural fibrotic scarring host response injury toward one of constructive remodeling. Although the mechanism(s) by which ECM scaffolds promote constructive remodeling are not completely understood, rapid proteolytic scaffold degradation with the generation of bioactive cryptic molecules, referred to as cryptic because they are hidden until released by proteases, that have potent immunomodulatory, angiogenic, chemoattractant, mitogenic, and antimicrobial properties [47–50,83–91], recruitment of multipotent progenitor cells [27,49,92,93], site-appropriate mechanical loading [94–96], and modulation of the host innate immune response toward a regulatory and constructive phenotype [38,39,97], have all been shown to be important events in this process. Each of these mechanisms will be discussed in detail.
7.3.1 Biologic Scaffold Degradation and Recruitment of Stem/Progenitor Cells
Biologic scaffolds composed of ECM are rapidly infiltrated by host polymorphonuclear (PMN) and mononuclear cells in vivo, a process which initiates scaffold degradation. Macrophages in particular have been shown to be key facilitators of this degradation process, as their depletion prevents the degradation of ECM scaffolds [98]. Quantitative studies utilizing ECM scaffolds radiolabeled with 14C labeled proline, an amino acid that is the building block for hydroxyproline which constitutes approximately 10% of each collagen molecule, were conducted to track their degradation profile in vivo. Since 14C labeled proline is integrally bound to every collagen molecule, a decrease in the radioactivity per unit weight of tissue corresponds directly to a decrease in the mass of the scaffold. These studies showed that approximately 40% of the ECM scaffold was degraded after 28 days and virtually all of the ECM was replaced with host tissue by 90 days postimplantation [87–89]; a process partially dependent on the anatomic site of placement and the tissue source of the ECM.
Since the degradation of ECM scaffolds in vivo is critical to creating an optimal microenvironment that enhances constructive remodeling [48,49,91,99,100], a number of studies have evaluated the biologic activity of ECM degradation products. Several in vitro studies have shown that low molecular weight cryptic oligopeptides (5–16 kDa) are formed through ECM degradation [49,83,91]. These peptides show potent bioactivity not present in the parent ECM proteins. For example, these cryptic peptides have antibacterial activity against both gram-negative Escherichia coli and gram-positive Staphylococcus aureus. Several preclinical and clinical studies confirm resistance to deliberate bacterial contamination in a surgical site repaired with ECM scaffold materials [84,85].
Furthermore, ECM degradation products have shown chemotactic activity for a number of stem/progenitor cells in vitro including: multipotential progenitor cells [49], Sox2+ cells [101], and multipotent perivascular progenitor cells [93,99,102,103]. Furthermore, endogenous recruitment of host multipotential progenitor cells (e.g., Sox2+, Rex1+, Sca1+, CD133+, CD34+, and perivascular stem cells [CD146+/NG2+]) to the site of ECM scaffold degradation in vivo has been demonstrated [24,27,49,92,99,101–106]. Stated differently, modifying the microenvironmental niche of the wound site by placement of an ECM scaffold induces the endogenous recruitment of perivascular stem/progenitor cells. However, the contributions of ECM degradation products to tissue remodeling encompass more than just resistance to bacterial contamination and stem cell recruitment, as cellular differentiation can also be affected [27,48,49,91,93,99,107]. For example, a cryptic peptide isolated from collagen III has been shown to accelerate osteogenesis of perivascular stem/progenitor cells in vitro and can initiate new bone formation at the site of injury [100]. Another study showed that cells isolated from the injury site of animals treated with biologic scaffold degradation products had the ability to differentiate down both mesodermal and neuroectodermal lineages in vitro, whereas cells from control animals could only differentiate to a mesodermal lineage [99]. These studies suggest that specific products of biologic scaffold degradation may direct stem cells down a specific lineage pathway and/or accelerate differentiation. In addition to cryptic peptides, growth factors such as vascular endothelial growth factor (VEGF), basic fibroblast growth factor (bFGF), and transforming growth factor beta (TGF-β) can be liberated during the degradation of the ECM scaffold and subsequently exert their biologic effects (i.e., angiogenesis, mitogenesis, and/or cellular differentiation) to aid in the remodeling process [50,81,108].
In summary, unlike synthetic materials, the degradation of ECM scaffolds is an important and requisite process with bioactive consequences (i.e., release of cryptic peptides, growth factors, and cytokines) that work in concert to facilitate a microenvironment that enhances constructive tissue remodeling [48,49,91,99,100]. It is logical therefore that inhibition of scaffold degradation by chemical cross-linking of the ECM will eliminate the beneficial effects of ECM degradation products and ultimately result in a chronic inflammatory response and encapsulation.
7.3.2 The Effect of Site-Appropriate Mechanical Loading
It is widely accepted that passive range of motion and mechanical loading can have a positive and substantial effect on the endogenous healing response. Accordingly, several in vivo and in vitro studies have demonstrated that passive range of motion and tissue-specific biomechanical loading is essential for constructive remodeling of ECM scaffold materials. For example, Hodde et al. conducted a study to investigate the effect of range of motion on constructive remodeling of ECM scaffolds when used as an Achilles tendon repair material in the rabbit. Following surgical manipulation, animals were subject to one of three groups for 4 weeks: (a) full range of motion, (b) partial range of motion (60–90° of flexion), or (c) no range of motion. Histological analysis of the groups with a partial or full range of motion showed dense collagenous connective tissue oriented along the longitudinal axis of the tendon. Spindle-shaped cells were distributed throughout the tendon and oriented along the longitudinal axis of the tendon. The ECM material in rabbits with no range of motion was associated with an increased presence of nonaligned fibroblasts, ECM, mononuclear cells, and blood vessels compared with native tendons. These findings demonstrate that applying physiologic range of motion to early postsurgically implanted ECM scaffold materials facilitates a constructive remodeling response. Additionally, a study by Boruch et al.
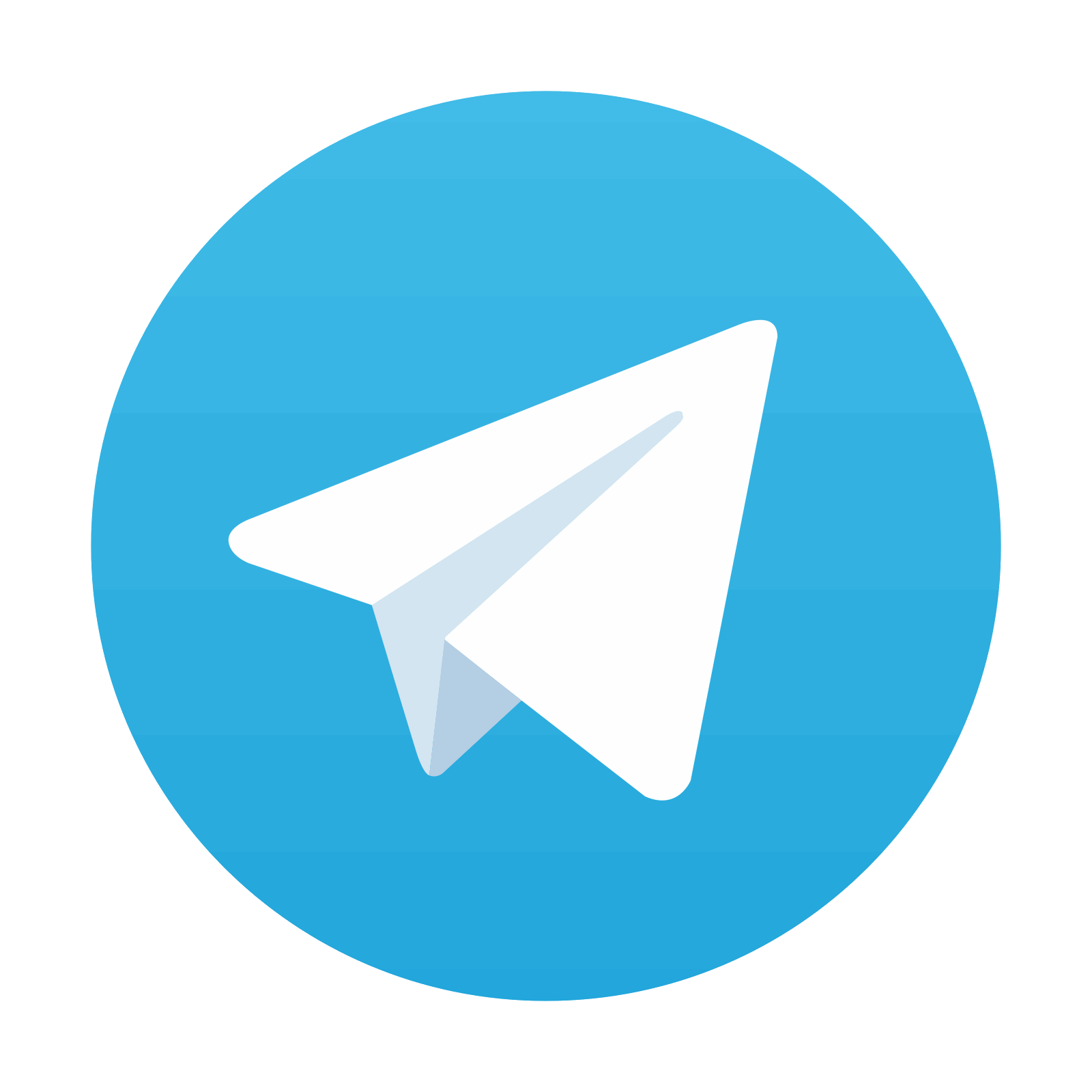
Stay updated, free articles. Join our Telegram channel

Full access? Get Clinical Tree
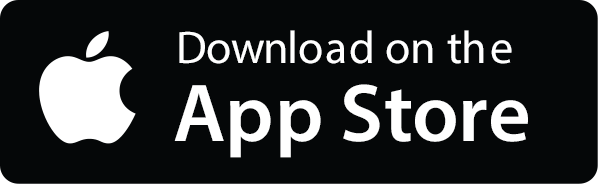
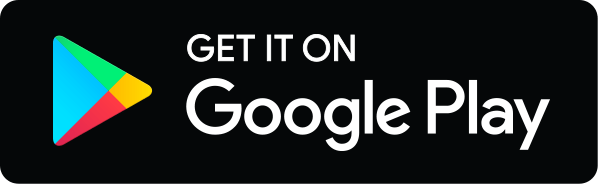