CHAPTER 3 Laura J. Chandler1, P. Rocco LaSala2, and Susan Whittier3 1 Corporal Michael Crescenz VA Medical Center and Department of Medicine Perelman School of Medicine at the University of Pennsylvania, Philadelphia, PA, USA 2 West Virginia University Hospital, Morgantown, WV, USA 3 New York-Presbyterian/Columbia University Medical Center, New York, NY, USA Since the first edition of this book, major advances have been made for the rapid identification of clinically significant aerobic bacteria. These include methods to definitively identify bacteria directly in clinical specimens and simpler, faster identification of isolated organisms with mass spectrometry (MS). Technologies are evolving quickly, and methods such as matrix-assisted laser-desorption ionization time-of-flight (MALDI-ToF) MS and nucleic acid detection techniques are rapidly becoming commonplace in the laboratory. Mass spectrometry and certain molecular methods for detection and identification of microorganisms in clinical specimens are discussed in this chapter, and are also reviewed in detail in other chapters in this text. Although exciting new technologies for bacterial identification are emerging, many clinical microbiology laboratories still rely on biochemical (phenotype) testing for identification of the majority of isolates. However, the conventional biochemical tests will be relied upon less in the coming years as the new methods gain US Food and Drug Administration (FDA) approval and are implemented in laboratories. In this chapter, we update our review of the major systems available for identifying bacteria isolated in culture and directly in clinical specimens. Automation in the clinical microbiology laboratory has also improved in the past ten years. A significant improvement in the turnaround time for reporting organism identification has been seen for the phenotypic identification systems. Some of the automated systems can return results very quickly, with final identifications now being reported within hours for some groups of bacteria. In this edition, we have updated the lists of tests, manufacturers, and references. With the rapid pace of change in the clinical microbiology marketplace, the introduction and discontinuation of commercial kits are ongoing processes. Therefore, the omission of any tests or kits in this chapter, while likely to occur, is unintentional. Conventional methods for identification of bacteria rely on phenotypic characteristics and are largely based on biochemical reactions such as carbohydrate utilization and enzymatic modification of substrates. A panel of “positive” and “negative” values is then translated into a probability score based on Bayes theorem modeling [9, 84] using assumptions that (i) an unknown isolate has equal probability of belonging to any taxon, and (ii) each test is independent of another. These methods are very adaptable to different formats; conventional systems are commercially available in manual or automated formats (107). These systems perform optimally for identification of non-fastidious bacteria that grow well aerobically, although there are panels available for some groups of fastidious bacteria such as the Haemophilus/Actinobacillus/Cardiobacterium/Eikenella/Kingella (“HACEK”) group of organisms and anaerobic organisms. A number of different molecular methods are used for identification of bacteria, including direct probe hybridization, various iterations of nucleic acid amplification and detection, DNA microarrays, and DNA sequencing. Several methods have been FDA cleared and are available commercially. Some of the methods are discussed in this chapter, while others are discussed in the molecular diagnostics and other chapters. Fatty acid methyl ester analysis by gas chromatography (GC) has been used successfully for several groups of organisms including aerobic bacteria. Bacterial fatty acids are unique to each species. The procedure is relatively simple: fatty acids are extracted from pure isolates obtained in culture, then esterified to form fatty acid methyl esters (FAME). Since the fatty acid profile of bacteria is dependent on the media used and the temperature of incubation, standardization of the growth conditions prior to testing on the system is necessary. The FAMEs are extracted, then analyzed on a gas chromatograph. The Sherlock system (MIDI, Inc., Newark, DE) automates the preparation and analysis. Its databases for aerobic bacteria include about 165 species in addition to a bioterrorism library containing 21 species in seven genera. The Sherlock system is not currently (at the time of this writing) FDA cleared for diagnostic use; laboratories interested in using the MIDI for aerobic bacterial identification must validate the method in their own laboratory. Matrix-assisted laser-desorption ionization time-of-flight mass spectrometry (MALDI-ToF/MS) is an emerging technology for identification that is rapid, accurate, and inexpensive. The system is based on mass spectrometry. Proteins extracted from bacteria using a matrix of specialized molecules are ionized with a laser. The ionized particles are separated according to their mass-to-charge ratio, and detected using a specialized detection device (131). The characteristic patterns produced by this method can be used to classify and identify bacteria. Two major systems are currently available. The MALDI-ToF system has significant potential for use in the clinical laboratory, as it is rapid, accurate, and (after the initial outlay for the equipment) inexpensive to perform the testing in terms of reagents and labor [27, 42, 136]. Automated instruments for identification of aerobic bacteria use miniaturized biochemical panels that are incubated in the instrument and read at specified intervals. Software programs interpret the data collected from the results of the phenotypic reactions and generate a biochemical profile. The profiles are compared to databases to determine the identity of the organism. Results are highly dependent on appropriate inoculum preparation, incubation conditions, and the version of the software and database used. The automated phenotypic systems available with FDA clearance include Vitek 2 (bioMérieux), MicroScan (Siemens Healthcare Diagnostics), Phoenix (BD Diagnostic Systems), and Aris2X (Trek Sensititre, ThermoFisher Scientific). The Biolog OmniLog System (Biolog, Hayward, CA) uses automated biochemical testing for identification but is not FDA cleared at the time of this writing. A discussion by Petti, Carroll and Weinstein of manual and automated systems for detection and identification of microorganisms was recently reported [116]. The reader is referred to this report, as well as the first edition of this manual, for reviews of criteria for selecting a system, methods for evaluating an instrument or system, and other general considerations. Additional information can be found in other authoritative references that are mentioned in this chapter. The MicroScan system (Beckman Coulter, Brea, CA) (Figure 3.1) includes identification panels for rapid or conventional overnight identification; panels are available for identification only, or combined with antimicrobial susceptibility testing. Panels are 96-well microdilution trays that contain biochemical substrates. Each well in the panel is essentially a small test tube that holds about 115 μL of liquid. Conventional (overnight) panels contain chromogenic substrates, and require overnight growth of the organism in the plate, which is incubated at 35°C for 18–24 h; after adequate growth, the plates can be read visually (manually) or with an automated (WalkAway or autoSCAN-4) instrument system. Identification in conventional panels is based on the detection of pH changes, substrate utilization, and growth in the presence of antimicrobial agents. Rapid panels detect preformed enzyme using fluorogenic tests, and are incubated for 2 h at 35°C. Identification in the rapid fluorogenic panels is based on the detection of hydrolysis of fluorogenic substrates, pH changes following substrate utilization, production of specific metabolites, and the rate of production of specific metabolic byproducts after 2 h of incubation at 35°C. Reading of the Rapid Gram-negative panels must be performed on a WalkAway instrument, with the initial instrument reading at 40 min, and subsequent reads taken at regular intervals up to 7 h of incubation. Figure 3.1 MicroScan System. (© 2015 Beckman Coulter, Inc. All rights reserved. Used with permission.) The Phoenix Automated Microbiology System (Becton Dickinson, Sparks, MD) was launched in US markets in 2004 (Figure 3.2). This instrument houses up to 100 identification (ID) and/or combination ID–antimicrobial susceptibility testing (AST) trays simultaneously with complete random-access flexibility. Onboard incubation is coupled with a combination of detectors for spectrophotometric, colorimetric, and fluorometric analyses, with well interrogation performed roughly every 20 min. As with other systems, preparation of organism suspension, adjustment to standardized turbidity, transfer to appropriate identification tray, and inoculation of purity check cultures are all requirements for the Phoenix system. Somewhat distinct from other instruments, however, is an option for lower inoculum testing (0.25 vs. 0.5 McFarland) using standard Gram-negative and Gram-positive identification trays, potentially enabling a user to set up automated identifications with fewer isolated colonies [18, 37]. To expedite setup, the Phoenix AP automated processor was introduced in 2008. This instrument automatically adjusts the organism suspension to the appropriate turbidity and, coupled with a label printer and the Epicenter data management software (BD Diagnostics Systems, Sparks, MD), significantly reduces manual “hands-on” time relative to the standard preparation process [73]. In addition to linking the Phoenix Automated Microbiology System with the Phoenix AP, the Epicenter Plus module also offers connectivity to other BD instruments and provides both user and laboratory information system interfaces as well as a variety of customizable rules and data query/reporting functions. Figure 3.2 Phoenix System. (Courtesy and © Becton, Dickinson and Company.) Reagents for phenotype-based identifications on the Phoenix system include a panel for Gram-negative aerobic and facultative organisms (NID) and another for Gram-positive organisms (PID), both of which may be purchased as ID-only kits or as combination ID/AST trays (NMIC/ID and PMIC/ID panels, respectively). An additional combination panel (SMIC/ID) has also been introduced for ID/AST of Streptococcus spp. There are two instruments available for reading identification panels: the AutoReader and the Aris2X (Figure 3.3). The biochemical panels contain fluorometric reagents. Standard identification plates are available for Gram-negative (GNID) and Gram-positive (GPID) organisms. Figure 3.3 Thermo ScientificTM SensititreTM ARIS 2X ID/AST system. (Photos courtesy of ThermoFisher Scientific, copying is prohibited). Trek Diagnostics Systems (now a division of ThermoFisher Scientific) released an automated microbial identification system in the early 1980s. This included a 96-well plate format with four replicates of a 24-reaction test panel, an automated plate inoculator (now known as the Trek AIM), a mirror box for visual interpretations and a microcomputer for data entry and taxa calculations [149]. The semi-automated system required manual preparation of organism suspension, mineral oil overlay, attachment of adhesive plate cover with puncturing of specified wells, transfer to and from incubator, and post-incubation addition of some reagents in addition to visual endpoint determination and keystroke recording of positive and negative reactions. Performance of the Sensititre system relative to other manual phenotypic kits for identification of enteric Gram-negative bacilli was good [150]. Soon thereafter, the company released a modified broth microdilution methodology for bacterial susceptibility testing that incorporated a fluorogenic substrate for endpoint determination of growth. Use of this technology allowed for automated instrument interpretation, performed by the Trek Autoreader [149], and ultimately led to development and marketing of the AP80 and AP90 trays for Gram-negative and Gram-positive identification, respectively. Cleared by the FDA in 1989, the AP80 tray performed well in a multicenter study, correctly identifying 95% of enteric and nonfermenting organisms to the genus level [151]. The AP90 tray received US FDA clearance in 1998. The latest Sensititre identification trays marketed by Trek Diagnostics retain the automated fluorescent endpoint detection technology. These include both the GNID tray and the GPID tray, each of which may be read using the Autoreader, the updated Optiread automated system, or the fully automated ARIS instrument. Both trays employ a series of 32 enzymatic reactions such that three organisms may be tested simultaneously per 96-well tray; and manual mineral oil overlay is still required for some wells. Both systems utilize the SWIN software, with 15 genera (42 species, subspecies, or groups) present in the Gram-positive database and 51 genera (127 species, subspecies, or groups) plus one unnamed taxon in the Gram-negative database. Published studies of performance evaluations for either of these trays are not currently available at the time of this writing. The Vitek system (originally known as the Automicrobic System [AMS]; Figure 3.4) was developed by McDonnell Douglas in cooperation with NASA. The instrument system was released in the late 1970s and was intended for use in the direct [noncultivation dependent] detection of urinary pathogens by astronauts during space travel [141, 145]. This modular unit included individual stations for specimen dilution, inoculation and sealing of disposable plastic cards, incubation, and spectrophotometric reading of cards, as well as probability calculations and display of results; and it marked an innovative and radical departure from practice standards of the time. Improvements in the system allowed for expanded use for various microbial isolates until 1989, when the company and technology was purchased by bioMérieux (Marcy l’Etoile, France). Figure 3.4 Vitek System. (Courtesy of bioMérieux.) The bioMérieux Vitek instrument was engineered largely around the design and principles of the AMS, requiring the user to inoculate identification cards in a separate workstation prior to “onboard” incubation and reading phases. The system successively measured colorimetric or turbidometric changes within individual card wells to determine positive and negative endpoints. Several updates to the organism database (primarily reflective of shifts in prokaryotic taxonomy) as well as adjustments to the biochemical test batteries in newer card versions continued in an effort to improve system performance. As a result, the Vitek was adopted by many clinical laboratories throughout the 1990s due to its efficiency relative to traditional phenotypic testing, in addition to its accuracy compared with other manual and automated platforms [124]. In 1998, bioMérieux launched its Vitek 2 instrument, which reduced the amount of hands-on technologist time necessary by automating steps for organism suspension dilution (for antimicrobial susceptibility test cards), card-inoculation/sealing, and waste disposal. Inclusion of a card carrier and “smart” docking station provided a more efficient workflow, allowing users to scan identification (and susceptibility) cards and accession labels at multiple benches, thereby linking patient demographics, lot numbers, and organism information to the Vitek software centrally and providing immediate recognition by the instrument upon loading of the card carrier. Further, the Gram-negative and Gram-positive identification cards (i.e. ID-GNB and ID-GPC, respectively) incorporated a fluorogenic indicator that reduced the time to final identification relative to the older, colorimetric cards. These were subsequently phased out of production, however, in favor of a return to colorimetric cards (i.e. GN and GP) in the mid-2000s to improve identification accuracy. A smaller version of the instrument, the Vitek 2 Compact, offers 15, 30 and 60-card capacities but is somewhat less automated than its full-size sibling. By contrast, the Vitek 2 XL doubles the capacity of the Vitek instrument (120 vs. 60 cards) while maintaining near-full automation. Each of the instruments includes similar software, data storage, and retrieval systems and interfacing capabilities. Other similarities between the original Vitek instrument and Vitek 2 models include requirements for: (i) manually preparing saline suspensions of well-isolated colonies to a specified McFarland turbidity range; (ii) inoculating purity plates; (iii) performing off-line testing (e.g., oxidase, indole, motility, pigmentation, hemolysis) for some isolates when automated discrimination values are low. Because the large majority of studies published over the past 15 years evaluating the Vitek system focus on the Vitek 2, and because the original Vitek instrument is no longer manufactured, this chapter concentrates exclusively on performance characteristics of Vitek 2. The Sherlock system (MIDI, Inc., Newark, Delaware; Figure 3.5) is based on “fingerprints” or profiles, of bacterial fatty acids, which are first extracted from bacteria, then converted to FAMEs. High-resolution gas chromatography is used to analyze the fatty acids, generating a pattern that is unique for each species of bacteria. The patterns are analyzed with software and compared to the patterns in the database to generate the identification. As of the time of this writing the Sherlock system is not currently FDA cleared. A rapid version of the FAME, called Q-FAME, is also available. Figure 3.5 Sherlock MIDI System. (Courtesy of MIDI, Inc.) Table 3.1 is an overview of currently available commercial systems that can be used for the identification of Gram-positive cocci. There are significant variations in the organism databases and time to results. When considering system implementation, each laboratory should take into account what best meets its needs with respect to commonly encountered organisms, space requirements, ability to interface to a laboratory information system, amount of disposables, turn-around-time to identification, and cost. Table 3.1 Commercial identification systems for Gram-positive cocci The BBL Crystal Gram-Pos ID System (BD Biosciences, Sparks, MD) identifies 13 different genera of aerobic Gram-positive bacteria. The panel consists of 29 biochemical and enzymatic substrates that are reconstituted with a suspension of the organism. After overnight incubation, the panels are read using the BBL Crystal Panel Viewer (Figure 3.6), which utilizes a combination of white and UV light sources to interpret reactions and obtain a profile number. Figure 3.6 BBL Crystal and Autoreader. (Courtesy and © Becton, Dickinson and Company.) The BBL Crystal Rapid Gram-Pos ID System identifies nine different genera of aerobic Gram-positive bacteria. The panel consists of 29 biochemicals and enzymatic substrates that are reconstituted with a suspension of the organism. After 4 h incubation, the panels are read using the BBL Crystal Panel Viewer, which utilizes a combination of white and UV light sources to interpret reactions and obtain a profile number. The Phoenix Gram Pos Panels (PMIC/ID and PID), developed for the BD Phoenix Automated Microbiology System, identify 30 different genera (140 species and subspecies) of aerobic Gram-positive bacteria. The identification panel incorporates wells for hydrolysis (n = 29) and utilization (n = 16) of various substrates with colorimetric and fluorometric indicators, two wells for polymyxin resistance, and one for nitrocefin degradation, with two wells serving as growth controls. The instrument reads inoculated panels every 20 min for up to 16 h. Time to results generally requires only 3–4 h for identification-only panels [38, 87]. The panel is sealed and self-inoculating and the Phoenix instrument can accommodate up to 100 tests. Studies published on the performance of the Phoenix system for Gram-positive identification focus almost exclusively on Gram-positive cocci despite the fact that the Gram-positive database includes 14 genera of commonly encountered (e.g., Corynebacterium spp., Bacillus spp.) and/or medically relevant (e.g., Listeria monocytogenes, Rhodococcus equi, Erysipelothrix rhusiopathiae) bacilli. Concordance to the species level for streptococci, staphylococci and enterococci is generally high (>90%) relative to other phenotypic methods, but appears somewhat lower relative to MALDI-ToF/MS and/or sequence ID. In a 2006 study comparing Phoenix Gram Positive panels to conventional biochemical and MIDI Sherlock, the Phoenix Gram Positive panels had good agreement with the identification to the genus level of 99.7% for staphylococci and enterococci, with 100% of Staphylococcus aureus and 98% of coagulase-negative staphylococci identified correctly [23]. A study in 2008 showed that correct identification of coagulase-negative Staphylococcus spp. by Phoenix was 90.5% [18]. Investigations designed to assess the low-inoculum (0.25 McFarland) protocol have demonstrated comparable levels of performance [19, 26, 37]. The Vitek ID-GPC card, developed for the Vitek 2 System but no longer manufactured, identified 22 different genera of aerobic Gram-positive bacteria. The ID-GPC card contained a 46 fluorescent test battery, which included tests for 22 enzymes, 16 fermentation reactions, two decarboxylases and six ancillary tests, with final results available in approximately 8 h or less. Fifty-two taxa were represented in its database. In contrast to published evaluations of the ID-GNB, studies of the ID-GPC generally focused on single or related groups of Gram-positive cocci, with correct identification to the species level reported to be approximately 80–85% for streptococci/enterococci and approximately 85–90% for staphylococci relative to phenotypic comparator assays [2, 11, 26, 36, 40, 54, 79, 90, 97, 146]. Forty-six total biochemical tests are contained within the GP card, with 115 taxonomic groups defined in the Gram-positive database. Each of the cards is automatically inoculated and incubated onboard the instrument, and reaction wells are interrogated every 15 min. Mean time to organism identification is up to 8 h [49]. Studies that directly compared GP and ID-GPC cards for identification of staphylococci and pneumococci have demonstrated similar to slightly better performance for the GP card [2, 87]. Additional studies of GP card performance using various groups of Gram-positive organisms have reported correct taxonomic assignment for approximately 85–95% of all strains tested [54]. In a 2005 study comparing the Vitek 2 Gram-positive panels to the Phoenix system panels, Vitek correctly identified 100% of the 51 Enterococcus isolates that were tested [41]. Several other studies reported accuracies of 93% [90], and 92% [44] for Enterococcus spp. The MicroScan Pos ID Panels, developed for the WalkAway System, identify ten different genera of aerobic Gram-positive bacteria. A variety of 96-well microtiter plate formats are available for identification alone or in combination with susceptibility testing. Identification is based on a total of 27 different conventional and chromogenic tests. After 18–24 h of incubation, the WalkAway System interprets the reactions and provides an identification. In an evaluation of the MicroScan WalkAway with Positive Combo panels, the system identified 94.6% of staphylococci to the species level [113]. The Rapid Gram-Pos ID Panel, also developed for the WalkAway System, identifies seven different genera of aerobic Gram-positive bacteria. The 96-well microtiter plate incorporates 36 different fluorometric substrates or fluorometric indicators and relies on the detection of preformed enzymes for identification. After 2 h of incubation, the WalkAway System interprets the reactions and provides a definitive identification. A study comparing MicroScan, Vitek 2 and Phoenix showed no incorrect identification of Staphylococcus spp., Enterococcus spp., and other Gram-positive cocci [69]. Two types of panels are available from MicroScan (Beckman Coulter, Brea, CA) for identification of Gram-positive organisms, including Staphylococcus spp. Conventional overnight panels contain biochemical substrates used for bacterial identification after 18–24 h of incubation. Identification in conventional panels is based on the detection of pH changes, and substrate utilization. Rapid Fluorogenic panels provide a result after 2 h of incubation. The biochemical tests on these panels are designed to detect preformed enzymes. The Rapid Fluorogenic panels are based on the detection of hydrolysis of fluorogenic substrates, pH changes following substrate utilization, production of specific metabolic substrates, and the rate of production of specific metabolic byproducts. Conventional panels can be read manually or with automated instrumentation; rapid panels must be read with the WalkAway instrument. Gram Positive ID Panels that can be used to identify Staphylococcus spp. include Positive ID Type 3 (conventional overnight panel) and Rapid Pos ID Type 2 (Rapid panel). Two other studies to evaluate the Microscan Gram-positive identification panel indicated that supplemental off-line testing may be necessary for accurate identification of Enterococcus faecium [31, 67]. Enterococci and related organisms are difficult to identify with automated systems. Though designated specifically for classification of Streptococcus spp., the ID portion of the SMIC/ID trays is configured similar to the PMIC/ID and NMIC/ID trays, containing 45 individual wells for assessment of substrate utilization (n = 14) and enzymatic hydrolysis (n = 31) in addition to two growth control wells [74]. Unlike the other panels, however, the 0.25 McFarland protocol is not available for the streptococci ID panel. The most current taxonomic database includes 27 species, subspecies, and biotypes of β-hemolytic and viridans group Streptococcus spp. There are no published studies reporting a direct comparison of Phoenix SMIC/ID to PID or PMIC/ID trays. However, results of four investigations, each including more than 100 streptococcal isolates, demonstrated 88–97% accuracy for species-level identifications when compared to standard phenotypic ID. Identification of Streptococcus pneumoniae with automated systems has also been studied recently and shown to have good accuracy. In a study comparing the Phoenix and Vitek 2 cards, both systems were comparable in their performance (96.8% for Phoenix, 95.2% for Vitek), and both compared favorably to manual identification methods. Each system returned identifications more rapidly than conventional testing [97]. The Phoenix system has been evaluated in several large studies for the identification of Enterococcus spp., including studies that incorporate direct inoculation of panels from positive blood culture vials [18, 23, 41]. In all studies, the Phoenix system performed well for identification of E. faecium, and E. faecalis. The Biolog GEN III MicroPlate and the GP2 MicroPlate panel (GEN II for Gram-positive bacteria) are available for identification of Gram-positive organisms. No recent studies describing the performance characteristics of the Biolog plates for identification of clinically significant human pathogens have been published as of the time of this writing. The Biolog system is shown in Figure 3.7. Figure 3.7 Biolog System. (Courtesy of Biolog.) A complete description of the Sensititre system (Trek Diagnostics, ThermoFisher Scientific) can be found earlier in this chapter. Staphylococci are Gram-positive, catalase positive organisms that appear microscopically as cocci in clusters. The genus Staphylococcus is comprised of approximately 39 species, which include 21 subspecies. While the isolation of Staphylococcus species, particularly non-S. aureus, may represent commensal microbiota, in the appropriate patient and/or clinical setting all species have the potential to be true pathogens. Staphylococcus aureus is the most common species associated with disease and the spectrum of clinical presentations is broad. Staphylococcus aureus is associated with skin infections (impetigo, furuncles, folliculitis), abscesses, pneumonia, osteomyelitis, endocarditis, sepsis, and many other infectious processes [94]. The increasing use of indwelling medical devices has contributed to the recognition of coagulase-negative staphylococci as pathogens as well. This group of organisms, in particular S. epidermidis, has been associated with bacteremia, endocarditis, surgical wound infections, and ventricular shunt infections [28, 52, 126, 127]. The ability of S. aureus to clot plasma is one of the key differentiators of this organism when compared to other species within the genera. One of the earliest methods used to detect coagulase production was to mix a suspension of organism with plasma, incubate for 4 h at 37°C and observe for clot formation. Due to inherent issues with both false-positive and false-negative results, other methods have been developed. Clumping factor A can also be used as a diagnostic tool. This cell-wall associated protein leads to aggregation of S. aureus in the presence of plasma. This reaction must be read within 10 s in order to reduce the chance of false-positive reactions. Staphylococcus lugdunensis and Staphylococcus schleiferi are also positive for clumping factor A, which can impact the specificity of this reaction. Numerous commercial kits are available for the detection of clumping factor, protein A, and capsular polysaccharides. Table 3.2 summarizes selected products available in the United States for the rapid identification of S. aureus from isolated culture colonies. The predominant methodology is latex agglutination, although hemagglutination and DNA probe technologies are also available. Performance of some assays may vary depending on whether the isolate is methicillin susceptible or resistant; the performance characteristics of these kits range from 93 to 100% sensitivity and specificity [32]. Some strains of methicillin-resistant S. aureus (MRSA) are thought to block clumping factor and protein A with capsular antigens. Therefore, the incorporation of capsular antibodies can improve the sensitivity for MRSA [114]. A recent publication [6] examined the performance of a “fourth generation” latex agglutination assay for the identification of S. aureus. It incorporated the detection of coagulase, protein A, and capsular polysaccharides, similar to third generation assays, and also a blue carboxylated microparticle. It was found to be equal to current assays and is currently not available in the United States at the time of this writing. Table 3.2 Rapid assays for the identification of Staphylococcus aureus Tables 3.3 and 3.4 are listings of selected methods available for the rapid identification of MRSA from culture and for the identification of S. aureus direct from specimens. The performance characteristics of these methods are > 90% sensitivity and specificity [31, 85, 128, 153, 163]. Table 3.3 Rapid identification of MRSA from culture Table 3.4 Rapid identification of Staphylococcus aureus direct from specimens The API RAPIDEC Staph kit (bioMérieux) identifies S. aureus, S. epidermidis, and S. saprophyticus. A plastic strip with six cupules is inoculated with a suspension of organism and after 2 h results are available. The test includes control and test cupules for fluorogenic detection of coagulase and chromogenic substrates for alkaline phosphatase and β-galactosidase. A UV lamp is required to read the fluorescent coagulase substrate. One publication demonstrated that RAPIDEC Staph correctly identified 100% of 130 S. aureus strains, 70.3% of 74 S. epidermidis strains, and 81.3% of 32 S. saprophyticus strains [68]. The API STAPH kit (bioMérieux) (Figure 3.8) identifies Staphylococcus, Micrococcus, and Kocuria spp. A plastic strip with 20 microtubes is inoculated with a suspension of organism and after overnight incubation color changes associated with each reaction are read and interpreted. The identification can be determined by locating the numerical profile in the manual database or by using APIweb identification software. Figure 3.8 API Staph. (Courtesy of bioMérieux.) The Microgen Staph ID (distributed in the United States by Hardy Diagnostics) identifies Staphylococcus, Micrococcus, Kocuria, and Kytococcus spp. Twelve biochemical substrates in a microwell strip are reconstituted with a suspension of the organism. After overnight incubation, a numerical profile is determined based on visual inspection of biochemical reactions, and then interpreted by the Microgen Identification System software (MID-60). The RapID STAPH PLUS system (Remel Inc., ThermoFisher Scientific, Lenexa, KS) (see Figure 3.9 for example of RapID kit) identifies Staphylococcus, Micrococcus, Kocuria and Rothia spp. Each panel consists of 18 reaction cavities that are reconstituted with a suspension of the organism. After 4–6 h incubation the reaction cavities are visually examined for color change. Identification is obtained by comparison of reactions to a differential chart or by the Electronic RapID Compendium (ERIC) software (Remel, Inc). Figure 3.9 RapID Kits. (Thermo ScientificTM RapIDTM NH system. Photos courtesy of ThermoFisher Scientific, copying is prohibited; also see Table 3.13) Streptococci are Gram-positive, catalase-negative organisms that appear microscopically as cocci in chains and pairs. Streptococci and related organisms comprise 17 different genera. Historically, the presence or absence of β-hemolysis formed the foundation for classifying streptococcal species. Currently, the term “pyogenic” is used to describe the β-hemolytic streptococci, as well as additional species such as S. dysgalactiae subsp. dysgalactiae. Characterization of species by Lancefield grouping is also becoming less definitive. A list of the commercial kits for grouping of β-hemolytic streptococci isolated in culture is found in Table 3.5. As with the staphylococci, the clinical significance of isolating Streptococcus and Streptococcus-like organisms in culture will depend on the clinical presentation and the immune status of the host. Infectious processes vary widely and can include pharyngitis, skin and soft tissue infections, neonatal infections, pneumonia, endocarditis, and septicemia. The “nonpyogenic” species include the α- and nonhemolytic organisms such as S. pneumoniae [43]. Table 3.5 Kits for grouping β-hemolytic Streptococcus isolated in culture Streptococcus pneumoniae is a major cause of community-acquired pneumonia. Rapid diagnosis of invasive disease caused by S. pneumoniae is of importance clinically. The Binax NOW S. pneumoniae test (Alere, Inc., Waltham, MA), shown in Figure 3.10, is a rapid immunochromatographic test that detects pneumococcal antigen in urine and cerebrospinal fluid (CSF) specimens, and was approved by the FDA in 2003. In a prospective clinical evaluation of the Binax NOW test, the sensitivity of the test was 82%, and the specificity was 97% [139]. A more recent study showed the sensitivity of the Binax test to be 88%, with a specificity of 96% [140]. A second method is the Trinity Biotech UNI-Gold S. pneumoniae immunoassay which is currently Conformité Européenne/European Conformity (CE) cleared but not yet cleared by the US FDA at the time of this writing. Figure 3.10 Binax NOW Streptococcus pneumoniae antigen test kit. (Courtesy of Alere, Inc.) Rapid antigen tests for the direct detection of group A streptococcal antigen in throat swabs have been used for many years. Current practice guidelines recommend use of rapid detection methods for Group A streptococcal pharyngitis [138]. A number of rapid tests are available for the direct detection of Group A streptococcal antigen from throat swabs [62, 118, 158]. Compared to culture, the direct detection methods range in sensitivity from 75 to 85%. Negative test results should be followed with culture. An increase in self-contained direct detection tests using an immunochromatographic test format has occurred during the past 10–15 years. Many of these newer tests have been granted waived test status under the Clinical Laboratory Improvement Amendments of 1988 (CLIA 88) and they are used in physician’s offices, clinics and emergency departments. These tests have important utility in point-of-care testing, with results available in less than 10 min. Early detection of Group A streptococcal pharyngitis allows the appropriate and timely use of antimicrobial agents [55, 89]. It is not uncommon for a single manufacturer of a rapid waived test to enter into marketing agreements with a few different distributors, resulting in the test being sold under different names. Accurate test results will depend on both sampling technique and the amount of organism present in the specimen [55]. A list of the currently available rapid Group A Streptococcus tests is found in Table 3.6. Table 3.6 Kits for the direct detection of Group A Streptococcus antigen in throat specimens Three molecular assays for the direct detection of Group A Streptococcus in pharyngeal specimens are currently commercially available (Table 3.7). Two of these are FDA cleared at the time of this writing, while one is available as a laboratory-developed assay. In two studies comparing the Illumigene (Meridian Bioscience, Cincinnati, OH) Group A Streptococcus DNA assay to culture, the sensitivity and specificity were > 99.0% [5, 63]. Table 3.7 Molecular tests for the direct detection of Group A Streptococcus in throat specimens Molecular methods are currently a recommended method for the rapid identification of Group B Streptococcus directly in vaginal/rectal specimens or in broth-enriched specimens because of their superior sensitivities and specificities [133]. Five FDA-cleared systems (Table 3.8) are commercially available: BD GeneOhm Strep B Assay, BD Max GBS Assay (Figure 3.11), Cepheid Smart GBS, Cepheid Xpert GBS, and Illumigene GBS (Figure 3.12) from Meridian. Sensitivities range from 78 to 99% and specificities range from 90 to 97%. In a multicenter study comparing the Smart Group B Streptococcus assay with an enrichment protocol to the BD GeneOhm StrepB direct, the Smart assay had a sensitivity of 98.6% and specificity of 90% [70]. Sensitivity of the molecular assays is increased when the test is performed on enriched samples (e.g., incubated in selected broth preparations prior to performing the assay). In an evaluation of the BD MAX GBS assay performed on broth-enriched samples, the sensitivity and specificity were 100% compared to culture [132]. Table 3.8 lists the commercial methods available for rapid identification of Group B Streptococcus directly from vaginal/rectal specimens. Table 3.8 Rapid identification of Group B Streptococcus direct from vaginal/rectal specimens Figure 3.11 BD Max System. (Courtesy and © Becton, Dickinson and Company.) Figure 3.12 Meridian Bioscience Illumigene System. (Courtesy of Meridian Bioscience, Inc.) Enterococci are catalase-negative, Gram-positive cocci that are normally found in the intestines of humans and animals, but are pathogenic in certain circumstances. Identification of Enterococcus spp. is important because these organisms are intrinsically resistant to many antimicrobial agents, and they may acquire resistance to vancomycin [46]. It is also necessary to differentiate between enterococci and the related Gram-positive cocci such as Lactococcus, Leuconostoc, Pediococcus, and Vagococcus. Commercial systems for the identification of Enterococcus spp. are listed in Table 3.1. In a study comparing the BBL Crystal to API Rapid ID 32 Strep, the BBL Crystal system correctly identified 96% of enterococci [59]. The API 20 Strep kit (bioMérieux) identifies Streptococcus spp. and seven other genera (Table 3.1). A plastic strip with 20 microtubes, which determines enzymatic activity or fermentation of sugars, is inoculated with a suspension of organism. Reactions are first read at 4 h after the addition of reagents. If a definitive identification is not obtained, the strip is incubated for up to an additional 20 h. The identification can be determined by locating the numerical profile in the manual database or by using APIweb identification software.
Rapid Devices and Instruments for the Identification of Aerobic Bacteria
3.1 Introduction
3.2 Major methods currently available
3.2.1 Conventional identification methods: phenotypic testing with biochemicals
3.2.2 Molecular identification methods
3.2.3 Fatty acid analysis with gas chromatography
3.2.4 Protein mass spectrometry
3.2.5 Automated systems for the phenotypic identification of aerobic bacteria
3.2.5.1 MicroScan
3.2.5.2 Phoenix
3.2.5.3 Sensititre
3.2.5.4 Vitek
3.2.5.5 The Sherlock system
3.3 Commercial systems for identification of Gram-positive organisms
3.3.1 Commercial identification systems for aerobic Gram-positive cocci
3.3.1.1 BBL Crystal Gram-Pos ID System
3.3.1.2 BBL Crystal Rapid Gram-Pos ID System
3.3.1.3 Phoenix Gram Pos Panel
3.3.1.4 Vitek ID-GPC
3.3.1.5 Vitek GP
3.3.1.6 MicroScan Pos ID Panels/Rapid Gram-Pos ID Panels
3.3.1.7 Phoenix SMIC/ID Panels
3.3.1.8 Biolog MicroPlate systems
3.3.1.9 Sensititre
3.3.1.10 Staphylococcus species
Manufacturer
Assay
Method
Arlington Scientific
Staphslide
Latex agglutination
Becton Dickinson
BBL Staphyloslide
Hemagglutination
bioMérieux
Slidex Staph-Plus
Latex agglutination
Hologic
Accuprobe
DNA probe
MKL Diagnostics
Phadebact Staph aureus
Latex agglutination
Oxoid
Dryspot Staphytect Plus
Latex agglutination
Oxoid
Staphytect Plus
Latex agglutination
Oxoid
Staphylase
Hemagglutination
Pro-Lab Diagnostics
Prolex-Xtra Staph Latex
Latex agglutination
Remel
Staphaurex
Latex agglutination
Remel
Staphaurex Plus
Latex agglutination
Manufacturer
Assay
Method
Time to results (min)
Alere
Alere PBP2a
Immunochromatography
6
Denka Seiken and Oxoid
MRSA Latex Test for PBP2’
Latex agglutination
15
Specimen
Manufacturer
Assay
Organism
Method
Time to results (min)
Nares Swab
BD
Geneohm MRSA ACP Assay
MRSA
PCR
120
Cepheid
GeneXpert SA nasal Complete (MRSA SA)
MRSA
MSSA
PCR
60
Roche
LightCycler MRSA Advanced Test
MRSA
PCR
120
Skin and soft tissue
Cepheid
GeneXpert SA Wound Complete (MRSA SA)
MRSA
MSSA
PCR
60
Positive blood culture bottle
AdvanDx
Staphylococcus QuickFISH Blood Culture Kit
SA
Nucleic acid hybridization
60
AdvanDx
S. aureus/CNS PNA FISH
SA
Nucleic acid hybridization
20
Alere
Binax NOW Staphylococcus aureus test
SA
Immunochromatography
30
BD
Geneohm StaphSR
MRSA
MSSA
PCR
75
Nanosphere
Verigene Gram-positive Blood Culture
MRSA
MSSA
Microarray
150
Biofire
FilmArray BCID Panel
MRSA
MSSA
PCR
60
3.3.1.10.1 API RAPIDEC Staph
3.3.1.10.2 API STAPH
3.3.1.10.3 Microgen Staph ID
3.3.1.10.4 RapID STAPH PLUS
3.3.1.11 Streptococcus species, Enterococcus species, and related genera
Kit name
Manufacturer/supplier
Streppro Grouping Kit
Hardy Diagnostics
Streptex
ThermoFisher/Remel
Prolex Streptococcal Grouping Kit
Pro-Lab Diagnostics
PathoDX Latex Agglutination Strep Grouping Kit
Diagnostic Products Corp/Thermo Scientific
Oxoid Streptococcal Grouping Kit
Oxoid
PathoDXTra Streptococcal Grouping Kit
Oxoid
Slidex StreptoPlus
bioMérieux
Streptocard
Becton Dickinson
Meritec Strep
Meridian Diagnostics
Phadebact Streptococcus
MKL Diagnostics
3.3.1.11.1 Direct detection of Streptococcus pneumoniae antigen in urine specimens
3.3.1.11.2 Rapid systems for identification of Group A Streptococcus in clinical specimens
Kit name
Method
Supplier
BD Chek Group A Strep
Immunochromatographic
BD
Binax NOW Strep A
Lateral flow immunochromatographic
Alere
ICON DS StrepA Test
Dipstick immunochromatographic
Beckman-Coulter
BioStar Acceava Strep A Test
Lateral flow immunochromatographic
Inverness Medical Professional Diagnostics
Clearview Strep A Exact
Dipstick immunochromatographic
Alere
Genzyme OSOM StrepA Test
Lateral flow immunochromatographic
Sekisui Diagnostics
QuickVue Strep A Test
Dipstick immunochromatographic
Quidel
Immunocard STAT Strep A
Dipstick immunochromatographic
Meridian
SureVue Signature Strep A Test
Immunochromatographic
Fisher Healthcare
McKesson Strep A Test Cassette
Immunochromatographic
McKesson
Polymedco Poly Stat Strep A
Immunochromatographic
Polymedco
BD Directigen EZ Group A Strep Test
Rapid chromatographic immunoassay
BD
3.3.1.11.3 Molecular assay systems for identification of Group A Streptococcus in clinical specimens
Test name
Method
Manufacturer
Performance characteristics (%)
Reference
Illumigene Group A Streptococcus
LAMP DNA amplification
Meridian
Sensitivity 99
Specificity 99.6
5
Hologic Gen-Probe GASDirect Test
DNA probe assay
Hologic-GenProbe
Sensitivity 85.7
Specificity 97.8
115
Laboratory-Developed Light Cycler Assay
Real-time PCR
Roche
Sensitivity 93
Specificity 98
154
3.3.1.11.4 Rapid molecular assay systems for identification of Group B Streptococcus in clinical specimens
Manufacturer
Assay
Method
Time to results (min)
Sensitivity
Specificity
References
BD
BD GeneOhm Strep B
RT-PCR
70
94
96
129
BD
BD Max GBS
RT-PCR
60–120
95
97
128
Cepheid
Smart GBS
RT-PCR
75
78–99
90–97
68
Cepheid
Xpert GBS
RT-PCR
55
92–99
92–96
17
Meridian
Illumigene GBS
LAMP technology
<60
97
92
Data from manufacturer
3.3.1.11.5 Enterococcus spp.
3.3.1.11.6 Manual systems
BBL Crystal Gram-positive ID system
API 20 Strep
Microgen Strep ID
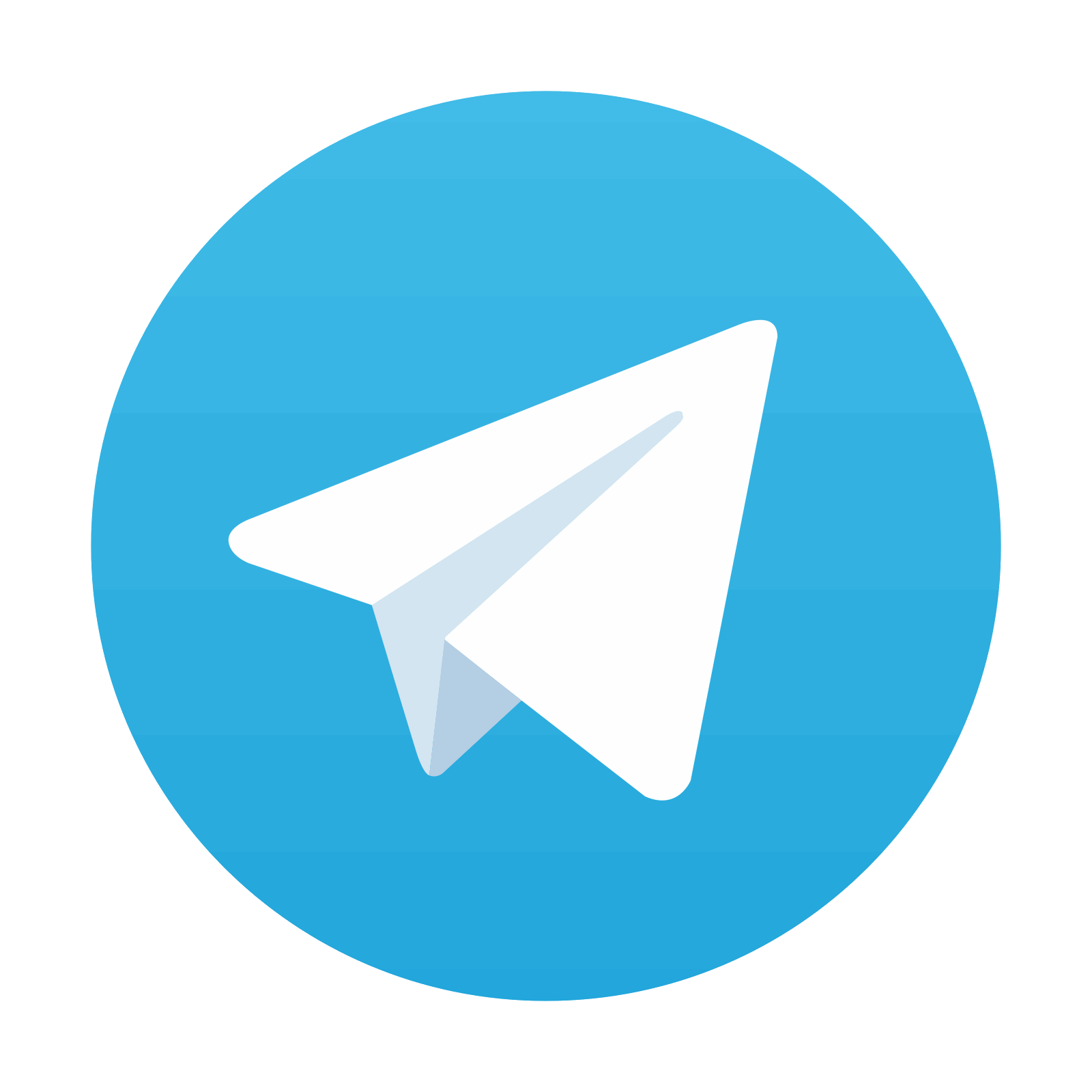
Stay updated, free articles. Join our Telegram channel

Full access? Get Clinical Tree
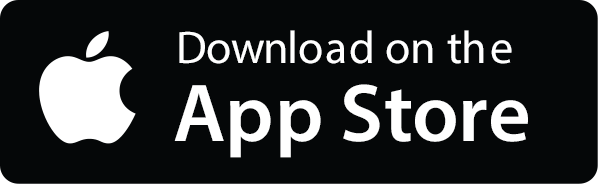
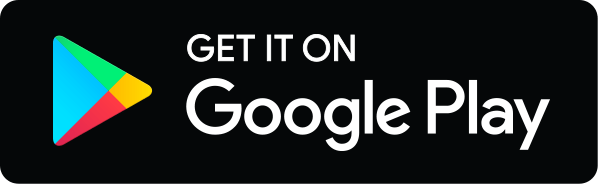