CHAPTER 21 Brett Laurence, Julie Collins, Carolyn Fernandes, Rafik Samuel, and Byungse Suh Temple University Hospital and Lewis Katz School of Medicine, Philadelphia, PA, USA Over the past 40 years the field of infectious diseases has undergone significant changes. We have witnessed the emergence of new infections such as Legionella, HIV, Hantavirus, and severe acute respiratory syndrome (SARS). In some cases, significant technological strides in diagnostics and molecular amplification have allowed us to identify new pathogens. In addition, with the development of resistance to antimicrobial therapy, diseases caused by previously well-characterized pathogens have presented new challenges to clinicians, as is the case with extremely drug resistant (XDR) tuberculosis. Thus, humans are constantly experiencing newly recognized infectious diseases and encountering previously known infections with new characteristics. Recently, emerging diseases have been defined as “Diseases of infectious origin whose incidence in humans has increased within the past 2 decades or threatens to increase in the near future”, by the Institute of Medicine. Although the definition may appear too restrictive, it gives us sound guidance in describing emerging infections. In the previous edition, we discussed ehrlichiosis, bartonellosis, bovine spongiform encephalopathy, HHV-8, West Nile Virus (WNV) encephalitis, and Escherichia coli O157:H7 among others [249]. In this chapter we review some of the most important organisms that have been identified as new human pathogens in the past 10 years as well as some previously known pathogens that have undergone significant changes in the same period. Four species of Plasmodium (falciparum, vivax, ovale, and malariae) have long been known to cause malaria in humans. Plasmodium knowlesi was discovered as a distinct malaria species in long-tail macaques in 1931. The following year, researchers demonstrated that the parasite could infect humans. However, in 2004, 120 cases of malaria due to P. knowlesi were reported in patients from Malaysia [245]. Since then, this pathogen has been increasingly recognized as a human malaria parasite in Southeast Asia. Infection with P. knowlesi has been studied extensively in primates, including in rhesus monkeys that develop fulminant disease from the parasite. The asexual cycle lasts 24 h, which is the shortest cycle among all types of primate malaria. Similar to P. falciparum, it can infect any age red blood cell [126] and there is no evidence of a latent stage in the liver [137]. In Malaysia, the primary natural vector in macaques is Anopheles hackeri, although many other species of Anopheles have been shown to transmit P. knowlesi as well. In 1965, the first natural infection of a human with P. knowlesi was reported in a traveler returning from what is now Malaysia, with the diagnosis being made on the basis of the quotidian fever pattern and the ability of the isolated parasite to cause overwhelming infection in rhesus monkeys [30]. In 1971, another suspected P. knowlesi infection was described in a patient returning from fieldwork in the jungle in Malaysia. The diagnosis was based on the morphology of the parasite on blood smear and convalescent serum for antibody titers [73]. Thus P. knowlesi was viewed as a malaria parasite of primates that was rarely documented to cause human disease until the report by Singh et al. in 2004 [245]. Subsequently, P. knowlesi infections have been reported throughout Southeast Asia [19,42,46,61,70,104,118,122,124,127,151,154,162,188,196,216,250,272]. Furthermore, archived blood samples from patients in Malaysia diagnosed with P. malariae infection in 1996 were reexamined and found to contain P. knowlesi DNA in 97% of the samples [153]. Since the growing recognition of P. knowlesi as a cause of human malaria, one case has been reported in the United States in a traveler returning from the Philippines in 2008 [39]. The clinical presentation of P. knowlesi infection is similar to that of the other human malarias, with fever, chills, headache, and malaise being the predominant symptoms. Gastrointestinal symptoms including nausea, vomiting, abdominal pain, and diarrhea were reported in 30–50% of patients in one case series [46] but were less common in another series, occurring in 5–15% of patients [245]. Disease can be severe, with 11 deaths reported in the literature to date [41,42,281]. The level of parasitemia with P. knowlesi infection can be quite high. In the first series reported by Singh et al. [245], approximately one-third of patients presented with parasitemia of over 5000 parasites/μL in blood with a maximum level of 117,600 parasites/μL in blood. All fatal cases reported have been associated with high levels of parasitemia, including one patient with 764,720 parasites/μL in blood [41,42,281]. Laboratory findings are notable for thrombocytopenia while anemia is uncommon [46]. Reinfection with different strains of P. knowlesi has been reported [148]. Transmission of P. knowlesi infection appears to occur primarily from primate to humans via the Anopheles mosquito. Human to human transmission has been documented under experimental conditions [30]. The mainstay of diagnosis of malaria in the community continues to be through the examination of thick and thin peripheral blood smears and this is the case for P. knowlesi as well. However, distinguishing P. knowlesi from other types of malaria by blood smear alone is very difficult. It is often mistaken as P. falciparum or P. malariae. The morphology of P. knowlesi malaria has been described in 10 patients in whom mono-infection with this species was confirmed by nested polymerase chain reaction (PCR) [154]. In nine of the ten patients, the infection was asynchronous at the time of presentation. Similar to P. falciparum and P. malariae, erythrocytes infected with P. knowlesi were not enlarged. Faint irregular dots, known as Sinton and Mulligan’s stippling, which is distinct from Schuffner’s dots sometimes seen in P. vivax infection, were observed in some infected erythrocytes. This appearance is similar to that of Ziemen’s stippling sometimes observed in P. malariae infection. The early trophozoite form of P. knowlesi closely resembles P. falciparum due to the presence of double chromatin dots, multiple parasites in a single erythrocyte, and appliqué forms. From the late trophozoite stage onward, there were no morphologic characteristics of P. knowlesi infection that distinguished it from P. malariae. (Table 21.1 and Figure 21.1) [154]. Table 21.1 Morphologic description of P. knowlesi compared to P. malariae Source: Adapted from Lee et al. [154]. Figure 21.1 Giemsa-stained thin blood films of different stages of P. knowlesi infection in human red blood cells (RBC). Early trophozoite (a), early trophozoite with double chromatin dots (b), single RBC with multiple early trophozoites (c), appliqué forms of early trophozoites (d), late trophozoites (e), mature trophozoites (f), schizonts (g), and gametocytes (h). (Adapted from Lee et al. [154], http://www.ncbi.nlm.nih.gov/pmc/articles/pmc2676309/.used under cc-BY 2.0; http://creativecommons.org/licenses/by/2.0/.) Given the difficulty in distinguishing P. knowlesi infection from other types of human malaria by microscopy, molecular methods for the detection of this infection have been pursued. PCR primers for diagnosis of malaria typically use small subunit rRNA genes that are distinct to each species of Plasmodium [277]. Primers with 100% specificity for P. knowlesi and the ability to detect as few as ten copies of P. knowlesi DNA/μL in blood have been developed [4,52,110,193]. Another molecular diagnostic method, loop-mediated isothermal amplification (LAMP), requires a less specialized laboratory set-up and therefore may be a better option to implement in the field. Two LAMP assays, one targeting β -tubulin genes [112] and one targeting the apical membrane antigen-1 gene [149] have been developed to detect P. knowlesi infection. The specificity and sensitivity of this method is reported to be equivalent to nested-PCR assays [112,149]. Another approach to the diagnosis of malaria is the use of rapid diagnostic tests (RDT), which check for Plasmodium antigens in human blood samples. There is limited, conflicting published experience with the use of RDTs in cases of proven P. knowlesi infection: tests have been reported as negative for all malaria species [19], positive for P. falciparum only [196], positive for non-falciparum malaria only, or positive for both P. falciparum and non-falciparum malaria [104,196]. Thus the currently available RDTs for human malaria are not reliable for use in the diagnosis of P. knowlesi infection. While access to molecular diagnostics remains limited and rapid diagnostic tests remain unreliable, the diagnosis of P. knowlesi infection should be considered in patients living in or returning from Southeast Asia with symptoms consistent with malaria. Markers that strongly suggest a diagnosis of P. knowlesi in such patients are exposure to the forest or forest fringe, and morphologic evidence of P. falciparum in the early trophozoite stage or of P. malariae by microscopy combined with a higher level of parasitemia or more severe disease than expected with P. malariae infection. There is limited data about the treatment of P. knowlesi infections. There are numerous case reports and small case series of different antimalarial agents successfully used for the treatment of P. knowlesi infection, albeit with varying degrees of follow-up: chloroquine [104,124,151,188,281], mefloquine [19], quinine with or without doxycycline [127,148,151,281], atovoquone/proguanil [39,70], sulfadoxine-pyrimethamine [245], and artemisinin derivatives [107,281]. In the first reported series by Singh et al., information about treatment was available for 94 patients with P. knowlesi infection. Two patients were treated with quinine and 92 were treated with chloroquine and primaquine. There was no sign of early treatment failure as evidenced by clearance of parasitemia in all patients and there were no deaths reported [245]. In another series of 87 patients, most were treated with chloroquine and primaquine with no deaths reported [46]. In Sabah, Malaysia, there were six deaths reported out of a group of 56 patients with P. knowlesi infection. All patients who died met World Health Organization (WHO) criteria for severe malaria. Five of these patients were treated with quinine and one was treated with artesunate. An additional 22 patients received quinine and 13 received an artemisinin derivative, all of whom survived [281]. One prospective observational study has investigated the response of P. knowlesi infection to oral chloroquine and primaquine in 60 patients with 28 days of follow-up. Only patients with uncomplicated infection were included (parasitemia of ≤ 100,000/μL). All patients received four doses of chloroquine and, if normal glucose-6-phosphate dehydrogenase levels, two doses of primaquine. All patients responded to treatment. Follow-up blood samples at 28 days found no evidence of P. knowlesi infection by nested PCR, suggesting that there was no resistance to chloroquine or primaquine and that there were no episodes of reinfection or recrudescence [45]. However, the use of primaquine is probably unnecessary given the lack of evidence for a latent stage of P. knowlesi infection in the liver [137]. There are no studies investigating the role of chemoprophylaxis for the prevention of P. knowlesi malaria. Given the response of infection to chloroquine, doxycycline, mefloquine, and atovaquone/proguanil, these agents may be effective for chemoprophylaxis as well. Clostridium difficile, a toxigenic Gram-positive spore-forming organism, was identified as the primary cause of antibiotic-associated diarrhea and colitis in 1977 [9]. During the past decade, a startling increase in rates of C. difficile infection (CDI) was noticed in the United States and Canada, and has spread worldwide, with increased morbidity and mortality [80,185,208]. This was associated with the emergence of a so-called “hypervirulent” strain. Three distinct features of this strain have led to a long and somewhat cumbersome description: (i) belonging to restriction endonuclease type BI; (ii) having North American pulsed-field type 1; and (iii) exhibiting PCR-ribotype 027. Hence this strain is called BI/NAP1/027 [159,175]. The reported incidence of CDI in the mid to late 1990s in acute care hospitals in the U.S. was stable at 30-40 cases per 100,000 population which rose to 50 cases in 2001 and further still, to 84 per 100,000 in 2005 [176,185]. In Canada, a retrospective study of all cases of CDI over a 13-year period in Quebec reported an increase in incidence from 35.6 cases per 100,000 population in 1991 to 156.3 cases in 2003 [208]. The clinical presentation was also more complicated, with increased 30-day mortality. This increase has been attributed largely to the spread of the BI/NAP1/027 strain, which prior to the year 2000 had accounted for fewer than 1% of US C. difficile isolates [175]. In England, a large outbreak caused by this strain occurred between 2003 and 2004 and again, in 2004 and 2005, at a single hospital, which resulted in a total of 334 CDI cases and 38 deaths [80]. Since then, cases of CDI caused by BI/NAP1/027 have been documented in multiple states in the USA, all provinces of Canada and many European nations, both causing outbreaks and sporadic disease [80]. Today, rates of CDI continue to rise, though not all are due to BI/NAP1/027 [10,28]. While healthcare-facility-associated CDI is well known, community-associated disease from the NAP1 strain is also recognized [10]. The virulence of C. difficile is due to the production of two toxins, TcdA and TcdB, originally described as an enterotoxin and cytotoxin, respectively [255]. The toxins are taken up by the colonic epithelial cells and they irreversibly glycosylate certain cellular proteins. This alters the actin cytoskeleton leading to cell death from impaired nutrient transport. The toxins are transcribed from a pathogenicity locus that comprises five genes: two toxin genes, tcdA and tcdB, and three regulatory genes, one of which (tcdC) encodes a putative negative regulator of toxin transcription [255]. Certain BI/NAP1/027 isolates carry tcdC gene deletion mutations that have been associated with an approximately 16-fold more toxin A and 23-fold more toxin B production in vitro than strains that do not have these mutations [276]. In addition, a third toxin is produced by the NAP1 strain; a binary toxin, CDT, which has enterotoxic effects in vitro. Its role, however, in the pathogenesis of clinical disease is not known, though speculation exists as to whether it may have synergistic effects with toxins A and B to cause severe disease [84]. Not all toxins are produced by all strains of C. difficile; in the Canadian outbreak, toxin A and B production occurred in about 65% of strains, binary toxin in 35% of strains while toxin B was produced by all strains [173]. Further studies have suggested that NAP1 strains have greater sporulation capacity in vitro than nonoutbreak strains, but other studies refute this claim [22,67]. Another unusual aspect of the NAP1 strain is the resistance to fluoroquinolones. All older isolates prior to 2000 were susceptible to the newer quinolones moxifloxacin, levofloxacin and gatifloxacin, but recent isolates are all resistant. The widespread use of fluoroquinolones in hospitals has likely promoted the spread of NAP1 strains due to selection pressure [159,175,185,207]. Patient risk factors for CDI have typically included hospitalization, advanced patient age, especially older than 65 years and antimicrobial exposure. Almost every antimicrobial class has been reported to lead to CDI due to suppression of the normal bowel flora, allowing C. difficile to flourish. A few are more common culprits, namely, clindamycin, cephalosporins, penicillins, and now fluoroquinolones due to the resistance patterns of the epidemic NAP1 strain [191]. The duration of exposure to antibiotics as well as exposure to multiple agents versus a single agent increases the risk for CDI [206]. Duration of hospitalization is also a risk factor, serving as a proxy for the duration and possibly degree of exposure to the organism from other patients with CDI [35]. A controversial area is the role of gastric acid suppressants in CDI. Various studies have shown an association between stomach-acid suppressing medications such as proton-pump inhibitors and histamine-2 blockers and CDI, but this association could be the result of confounding from underlying severe illness [48,49,159,185,206]. Other reported risk factors include cancer chemotherapy, HIV disease, gastrointestinal surgery or gastrointestinal tract manipulation, including tube feeding [35]. Potential sources of C. difficile in the community include soil, salt, tap and fresh water, pets, meats, and vegetables [191]. To date, there is no conclusive evidence that ingesting food contaminated with C. difficile has led to clinical disease in humans. Disease has also been reported among populations not previously at risk, such as healthy peripartum women and close contacts of patients with C. difficile infection with no other risk factor [29]. The primary mode of transmission is person-to-person spread through the fecal–oral route. The presence of spores on the hands of healthcare workers transiently contaminated could be a mode of spread given the lack of activity of alcohol-based hand rubs on spores that are routinely used in most hospitals [178]. Environmental contamination with the spores is also important in healthcare settings [53]. Prevalence of asymptomatic colonization with C. difficile varies from 7 to 26% in adult inpatient acute-care facilities, and between 5 and 7% among elderly patients in long-term care facilities [35]. Colonization risk increases during hospitalization indicating a daily cumulative risk of exposure. However, the risk of developing CDI appears to decrease rather than increase over time, suggesting that progression to diarrhea develops early or not at all [231]. A recent prospective study in Canada found that the NAP1 strain was predominant among patients with CDI, while asymptomatic carriers were more likely to be colonized with other strains of C. difficile [158]. The usual incubation period between exposure and development of CDI is not known but is estimated to be between 2 and 3 days [35]. This is different from the increased risk of CDI that persists for weeks after exposure to, and cessation of, antimicrobial agents. The clinical manifestations vary widely from a self-limited diarrheal disease that abates when the offending antibiotic is discontinued to fulminant disease that can result in death [255]. Watery diarrhea multiple times a day is usual with lower abdominal pain and cramping, low-grade fever and leukocytosis often over 15,000 cells/mm3 [255]. Physical examination can reveal lower abdominal tenderness. Sigmoidoscopy or colonoscopy may show patchy mild erythema and friability to severe pseudomembranous colitis. Unexplained leukocytosis in a hospitalized patient even without diarrhea may represent underlying CDI, though diarrhea often develops in the next 1–2 days [275]. Fulminant disease presents with diarrhea, severe lower quadrant or diffuse abdominal pain, abdominal distension, fever, hypotension, lactic acidosis, and a marked leukocytosis. Ileus may mask the diarrhea. Toxic megacolon and bowel perforation can also occur [275]. Small-bowel infection is very rare but enteritis may occur in the setting of prior colectomy [150]. Outbreaks caused by the BI/NAP1/027 strain have been associated with more severe disease and increased mortality. In Quebec, in 2003, the 30-day attributable mortality was 6.9%, four times higher than the previous national average in 1997 [159]. The proportion of complicated disease increased from 7.1% in 1991–1992 to 18.2% in 2003 [208]. A high leukocyte count (≥20,000 cells/mm3) and an elevated creatinine (≥2.3 mg/dL) were strongly associated with adverse outcomes, as was age ≥ 65 years [208]. In the outbreak in England where greater than 59% of the isolates were NAP1 strains, the attributable mortality was 11% [278]. Increased rates of recurrence have also been reported with the NAP1 strain [206]. The gold standard for the diagnosis of CDI had previously been either the cell culture cytotoxicity assay or toxigenic culture [35], but PCR is rapidly becoming the preferred test. Testing should be performed only on unformed stool unless ileus is suspected. Enzyme immunoassay (EIA) for toxins A and B is rapid but less sensitive (64–94%) [44]. Other useful tests include detecting the presence of GDH (glutamate dehydrogenase) in stool (sensitivity 88–100% with negative predictive value of 98.8–100%) or real-time PCR of feces to detect toxin genes (sensitivity 80–94%, negative predictive value > 98%) [58]. Some laboratories utilize a two-step testing algorithm using an initial screening test such as EIA combined with the GDH test, with further testing of positive samples by toxigenic culture, cell cytotoxicity assay or PCR [138]. Repeat testing during the same episode is of limited value [35]. Diagnosis of the BI/NAP1/027 strain, while important for epidemiological purposes, is not specifically important for the individual patient and clinical disease severity should guide treatment decisions. Stopping any offending antimicrobial agent is a key. However, some cases are not associated with any antimicrobial use and a good number do not appear until after the antibiotic has been stopped. Metronidazole 500 mg orally three times daily for 10–14 days is suitable for mild–moderate disease, while vancomycin 125 mg orally four times daily for 10–14 days is recommended for severe CDI [44]. Severe, complicated disease should be treated with vancomycin either orally or rectally (if ileus is present) with or without intravenous metronidazole [160,295]. Patients with toxic megacolon, lactic acidosis, and hypotension may require a colectomy [144]. There are some newer approaches to CDI treatment. Fidaxomicin was approved in the United States in 2011 to treat CDI. At a dose of 200 mg twice daily, it had similar cure rates, but lower rates of disease recurrence for strains other than the BI/NAP1/027 strain [144]. Treatment of a first recurrence is usually with the same agent that was used for the initial episode; however recurrences after the first should not be treated with metronidazole due to the risk of cumulative neurotoxicity [129]. A vancomycin taper, or pulse and taper, regimen is usually employed to mop up cells that are still in spore form but germinate after the vegetative cells have been eliminated [177]. Other therapeutic agents that have shown variable efficacy include rifaximin and nitazoxanide. Toxin-binding regimens using cholestyramine and colestipol [123] have been utilized, although these drugs can bind antimicrobials in the colon as well. Probiotic agents such as Lactobacillus species and Saccharomyces boulardii may have some benefit in conjunction with standard treatments. Bioremediation or “fecal transplant” where a stool from a healthy donor is instilled by either nasogastric tube or enema, has been tried with high rates of success but there are several limitations to this method [1]. Human monoclonal antibodies against toxins A and B given in addition to antibiotics have shown a significant reduction in the recurrence of CDI, including patients infected with the NAP1 strain in a phase-2 trial [161]. Prevention strategies for CDI include those directed against patient to patient or environment to patient transmission and those minimizing patient risk of acquisition if exposure occurs. Isolation techniques (private rooms, contact precautions), rigorous environmental cleaning along with good hand hygiene with soap and water is recommended after contact with patients with CDI [35]. Antimicrobial stewardship programs to help decrease antibiotic consumption also play an important role [185,262]. A C. difficile toxoid vaccine is currently being evaluated in a phase-2 trial for the prevention of CDI (clinicaltrials.gov NCT00772343). CDI has evolved over the past decade and is a major cause of infectious diarrhea in healthcare settings. Most of this change has been due to the emergence of the BI/NAP1/027 strain. Progress has been made in regards to novel agents to treat disease and a better understanding of risk factors and measures needed to control outbreaks. However, reductions in the prevalence of the NAP1 strain will not prevent the emergence of new epidemic strains in the future and continued surveillance is warranted. Influenza viruses are members of the Orthomyxoviridae family and are classified into types A, B, and C. All influenza types have segmented, negative-sense, single-stranded RNA genomes with host-derived cell envelopes, and surface glycoproteins: hemagglutinin (HA) and neuraminidase (NA), which determine the specific subtype. HA is responsible for binding and fusion to host cell receptors, often on respiratory epithelium, while NA is responsible for release of viral particles from infected cells by catalyzing the removal of terminal sialic acids. The M2 ion channel, unique to influenza A, is also on the cell envelope and is necessary for viral uncoating. Influenza A can infect a variety of hosts in addition to humans, including: swine, equine, avian, marine mammals, canines, and feline populations [258]. Alteration in HA or NA structure can lead to new variants (antigenic variation). Antigenic drift is due to point mutations that can slightly alter structural antigens. This occurs during and between influenza seasons and may permit reinfection even in patients who have previously been infected or immunized by a parent virus. Antigenic shift is due to virus reassortment in an animal reservoir, resulting in a new virus and in some cases there may be little or no population immunity. While birds are easily infected by influenza viruses and shed the virus in their GI tracts allowing for easy human exposure, pigs have receptors for both avian and human influenza virus strains and thus create a crucible for the exchange of genetic information and the potential to create novel subtypes to which humans have little immunity [186]. In March 2009, a novel swine-origin influenza A virus (H1N1) was reported in Mexico with subsequent reports in the United States. Airline travel helped spread human infection to multiple continents, resulting in the WHO declaring a pandemic in June 2009, which continued through August 2010 [133]. The pandemic is thought to be due to a reassortment of two swine strains that served as reservoirs for multiple cross-species transmissions. The novel virus resulted in an unusually high rate of morbidity and mortality in children, healthy young adults, and pregnant patients [298] and a comparatively low rate of morbidity for older adults (including the elderly). The Centers for Disease Control and Prevention (CDC) estimated that there were over 60 million cases of pandemic H1N1 (pH1N1) in the United States, with over 250,000 hospitalizations and 12,500 deaths. Pandemic H1N1 was less common in persons over 65 years likely due to preexisting cross-reacting antibodies in those born prior to 1930 [72,100,181]. Person-to-person transmission of influenza viruses occurs through contact with large-particle droplets and respiratory secretions, though fomites are also thought to play a role. In contrast to seasonal influenza epidemics that occur during the winter, with highest severity in the elderly and adults with co-morbid conditions, pandemics often affect multiple age groups outside of the winter season, with increased mortality in young adults. Pandemic H1N1 occurred in two waves, which peaked in June and October with a lower rate while children were out of school [120]. This pandemic H1N1 variant was shown to replicate to a higher degree than usual in tracheobronchial epithelium and alveolar epithelial cells, resulting in desquamation of respiratory epithelium, loss of cilia, and inflammation of the trachea and bronchi. Severe cases may progress to necrotizing bronchiolitis, diffuse alveolar damage with alveolar hemorrhage, edema, hyaline membrane formation, and organizing fibrosis [113]. The incubation period is 1.5–3 days, with viral shedding starting a day prior to symptom onset and persisting for 7 days. Immunosuppressed and transplant patients can shed virus for weeks [31]. Clinical symptoms of pH1N1 are consistent with other subtypes, including: fever, chills, sore throat, malaise, anorexia, myalgias, nonproductive cough, rhinorrhea, and headache. Infants may present with lethargy, poor feeding, irritability, tachypnea, or apnea. Gastrointestinal symptoms such as diarrhea and vomiting appear to be more common with pH1N1. Complications are most severe in children less than 2 years, the immunosuppressed, and those with underlying co-morbid conditions, such as chronic lung disease including asthma, cardiovascular disease, cancer, diabetes, and children with neurologic conditions, although a high proportion of otherwise healthy adults, obese, and pregnant patients were also affected. Pregnant patients are at increased risk of spontaneous abortion, preterm labor, and fetal distress. The highest number of hospitalization and deaths were in those < 50 years with chronic lung or cardiac conditions, pregnant patients in their third trimester, and children < 5 years with neurologic or pulmonary disorders (although many infected patients had no underlying medical condition) [25,75,115,116,120,140,152,155,246,289]. A high rate of complications was noted during the pandemic including: secondary bacterial pneumonia with Streptococcus pneumoniae, Staphylococcus aureus, and Haemophilus influenzae, hypoxemic respiratory failure with acute respiratory distress syndrome (ARDS) requiring mechanical ventilation, renal insufficiency, multisystem organ failure, rhabdomyolysis, encephalopathy, seizure, and myocarditis. Laboratory abnormalities may include elevated liver transaminases and lactate dehydrogenase with lymphopenia and anemia. Chest radiographs in hospitalized patients commonly have mixed interstitial and alveolar infiltrates that vary on computed tomography from patchy consolidation or multilobar infiltrates to ground glass opacities [116,289]. Rapid influenza diagnostic tests (RIDT) such as enzyme-linked immunosorbent assay (ELISA), optical immunoassay, or lateral flow are commercially available and can be performed in < 30 min. While the specificity is very high, the sensitivity of RIDT ranges from 40 to 75% without any difference in accuracy during the recent H1N1 pandemic [27]. RIDT are able to distinguish influenza A and B viruses but are unable to distinguish subtypes of influenza A, as is the case with direct and indirect immunofluorescent antibody testing (DFA and IFA), which require a fluorescence microscope and take 1–4 h to perform. The sensitivity and specificity are over 90% and 95%, respectively, when compared to real-time reverse transcriptase PCR (rRT-PCR) [212]. Viral culture on a variety of cell lines, including Madin–Darby canine kidney (MDCK) cells and primary human airway epithelial cells, often reveals growth in 3 days [113]. rRT-PCR remains the most sensitive and specific testing modality and is able to differentiate influenza types and subtypes, although cost and availability may inhibit routine use [242]. Nasopharyngeal aspirates (NPA) have generally been considered the specimen of choice, although the diagnostic yield of combined nasal and throat swabs (CNTS) has been reported to have a higher diagnostic yield [197]. Testing can also be performed on bronchoalveolar lavage (BAL) fluid in those with lower respiratory tract disease. Serologic testing is more useful for influenza surveillance rather than diagnosis. Pandemic H1N1 contains a mutation in the M2 protein that confers resistance to M2 inhibitors (adamantanes) [241]. Neuraminidase inhibitors remain the drugs of choice for pH1N1. Sporadic oseltamivir-resistance has occurred due to neuraminidase mutation H274Y and often in the setting of oseltamivir chemoprophylaxis or prolonged therapy [11,213]. Oseltamivir-resistant pH1N1 remains susceptible to zanamivir although the inhaled formulation can cause bronchospasm and clog ventilator tubing [213]. Intravenous peramivir is available with emergency use authorization (EUA) and although large clinical trials are lacking, it appears to be effective [289]. Peramivir requires dose adjustment for renal insufficiency and failure with peramivir has been reported in those with oseltamivir-resistant virus [106]. The addition of glucocorticoids to standard treatment has not demonstrated clinical benefit and may increase the risk of developing critical illness and death [95]. Postexposure chemoprophylaxis is reserved for those who have not been vaccinated against the current circulating strain, had a recent close influenza contact, and are at high risk for influenza complications. Preexposure prophylaxis is often reserved for patients in long-term care facilities who are at high risk for influenza complications. Contact and droplet precautions remain the standard of care for outbreak control [213]. Vaccine effectiveness is determined by the degree of match between vaccine strains and the circulating influenza strain, and has been shown to decrease incidence and duration of illness, missed work days, and physician visits. Different formulations of an inactivated, nonadjuvant, split-virion, monovalent pH1N1 vaccine have been developed and most have been safe and immunogenic. While young children and transplant recipients may require two doses, a single dose has been safe and immunogenic in elderly, HIV-infected, and pregnant patients with sufficient transfer of antibodies to newborns [114,156,224,254,261,290]. The 2009 pH1N1 strain was subsequently included in the 2010 trivalent influenza vaccine. A single dose is recommended for those at high risk for influenza complications and there have been no substantial increase in adverse events, such as Guillain–Barré syndrome, in those receiving the pH1N1 compared to the seasonal influenza vaccine [214]. Although it appears that the 2009 H1N1 pandemic strain has been controlled, there may be new pandemics at any time due to antigenic shift. It is possible that future pandemic strains will lead to significant morbidity and mortality in younger individuals, as was the case with the 1918 as well as the 2009 pandemic. In early May 2011 through July 2011, northern Germany was the epicenter of an epidemic of bloody diarrhea associated with the development of hemolytic-uremic syndrome (HUS) caused by a Shiga-toxin-producing E. coli (STEC). The clone of STEC classified as O104:H4 resulted in over 4000 cases of diarrheal illness, 900 cases of HUS, and 50 deaths in residents and travelers to northern Germany. The source is thought to be fecally contaminated sprouted fenugreek seeds. The strain was a novel bacterium containing a plasmid-encoded extended spectrum beta-lactamase (ESBL) and genes from enteroaggregative E. coli (EAEC), which enhanced survival and intestinal colonization. In contrast to the more common Shiga-toxin-producing O157:H7 E. coli, which causes HUS in 5–10% of children and the elderly, O104:H4 had a longer incubation period and predominantly affected young adult women with a higher rate of HUS (approximately 22%) [2,15,20,89,103,220]. The principle reservoir of many STEC are cattle, with colonization rates of 0–23%, although other species including pigs, chickens, ducks, sheep, and goats have been known to be carriers [99,142,257]. The German outbreak strain O104:H4 has been traced back to ingestion of fenugreek sprouts and specific distributors, however, fecal studies of cattle in the vicinity of the outbreak area in northern Germany failed to isolate O104:H4 or other EAEC, suggesting that the German outbreak strain may have an alternate unidentified reservoir within or outside of Germany or is particularly adapted to humans [20,280]. Some enterohemorrhagic E. coli, including O157:H7, contain a chromosomal pathogenicity island called locus enterocyte effacement (LEE) responsible for intestinal mucosa attachment and microvilli effacement. LEE also produces the eae gene product intimin, which is essential for adherence and effacement in addition to colonization of animals [13,142]. The German outbreak strain lacked this pathogenicity island and was an enteroaggregative E. coli (EAEC) strain, meaning that organisms formed bundles firmly attached to the intestinal mucosa. In conjunction with the virulence potential of a Shiga toxin, this organism was able to cause an exceptionally high rate of morbidity and mortality. The Shiga-toxin gene was acquired from a bacteriophage [220]. STEC can release Shiga (Vero) toxins Stx1 (Shiga-toxin 1) and/or Stx2 (Shiga-toxin 2), encoded by lysogenic bacteriophages, in the gut lumen. When the toxin enters the bloodstream, systemic disease can follow [55,130,142]. Shiga toxin is composed of one A subunit, which leads to cell death by inhibiting protein synthesis, and five B subunits, which bind to glycosphingolipid globotriosylceramide (Gb3) receptors in human renal and cerebral vasculature [215,237]. Ruminants lack vascular Gb3 receptors and therefore can remain colonized without developing active disease [142]. Stx2 is more commonly implicated in development of bloody diarrhea and a higher rate of hospitalization compared to Stx1 [103]. The German outbreak strain acquired a variant Shiga-toxin 2 (stx2a) bacteriophage in addition to a large plasmid encoding a CTX-M-15 ESBL and a TEM-1 ß-lactamase, and a gene cluster encoding aggregative adherence fimbria (AAF/I) [228]. In addition, a high pathogenicity island encoding an iron uptake system was also identified [14]. There has been a long association between STEC and HUS and more than 200 different E. coli O:H serotypes are associated with the production of Shiga toxins. Risk factors for development of HUS include infection with O157:H7, which contain higher rates of Stx2 and intimin, exposure to antimotility agents, age ≤ 7 years, and possibly exposure to antibiotics, which may exacerbate Shiga-toxin release [12,13,64,130,240]. STEC is the main cause of renal failure in childhood as a majority of children that develop HUS will require dialysis, with a 3–7% mortality rate and 5–10% with permanent renal or neurologic sequelae [103,237]. Typical HUS consists of the triad: nonimmune hemolytic anemia, thrombocytopenia, and acute renal dysfunction. Pathologically, fibrin thrombi form within small vessels, resulting in ischemic damage accompanied by glomerular endothelial cell swelling, tubular epithelial cell damage and necrosis, and mesangiolysis [190,215,257]. A small proportion of patients can also develop neurologic manifestations such as seizure and encephalopathy [33]. The German outbreak STEC strain had a longer median incubation period of 8 days, compared to 3–4 days with STEC O157:H7, with symptoms of frequent watery stools that became bloody accompanied by severe abdominal pain and cramping and less commonly nausea or vomiting. Fever was not a predominant feature and most patients had normal leukocyte levels, although lactate dehydrogenase and C-reactive protein levels were elevated. The average interval from diarrhea to development of HUS was 5 days [78]. The German outbreak strain resulted in a 22% rate of HUS with 88% of cases affecting adults, with highest rates in adult women aged 30–34 years. Only 2% of case patients with HUS were children < 5 years of age and their course was historically similar to O157:H7 with a low mortality rate [132]. Severe neurologic complications were also common, manifesting as encephalopathy and seizure [14]. For patients with a fatal outcome, death occurred a median of 10 days after onset of disease, with the highest death rates in elderly women (median age 74 years) [78]. Many STEC can be readily identified on sorbitol-containing selective media. In contrast, the O104:H4 strain can ferment sorbitol on sorbitol–MacConkey agar (SMAC) or cefixime tellurite–sorbitol MacConkey agar (CT-SMAC), and like most strains of E. coli it will ferment lactose. Pure culture is then needed for further serotyping and molecular characterization, including pulsed-field gel electrophoresis (PFGE), useful in outbreak settings, and EIA or PCR for detection of Shiga toxin. EIA testing of cultures is preferred as the amount of free fecal Shiga toxin is often low, resulting in lower sensitivity and specificity. Cell cytotoxicity assays using Vero (African green monkey kidney) and HeLa cell lines are sensitive for detection of Shiga toxin as they contain high concentrations of Gb3. Cytopathic effect is confirmed using specific neutralizing antibodies to Shiga-toxin 1 and 2, although the test can take up to 72 h to complete [88]. Treatment of HUS with fresh frozen plasma (FFP), steroids, and commercial immunoglobulin preparations are no better than supportive care [128,180]. Antimotility agents have been associated with increased risk of developing HUS and neurological complications. Antibiotics have been controversial with some studies showing progression of HUS due to bacteriolysis and bacteriophage induction with increased Shiga toxin gene expression in vitro and in vivo, specifically with quinolones and co-trimoxazole. Other studies have demonstrated no association while some have shown that early treatment with fosfomycin improved outcome and reduced the incidence of HUS [12,109,136,192,230,274,287,297]. Eculizumab, a recombinant humanized monoclonal antibody to C5 complement protein, commonly used in paroxysmal nocturnal hemoglobinuria, has been approved for HUS and case reports have shown success in STEC-HUS [146]. None of the above methods have been extensively studied in O104:H4 but a case series with early plasma exchange in five patients with HUS and central nervous system dysfunction has shown efficacy in the German outbreak strain [37]. The outbreak strain is resistant to trimethoprim-sulfamethoxazole, beta-lactam antibiotics and third-generation cephalosporins, partially resistant to quinolones (nalidixic acid), but remains sensitive to carbapenems and aminoglycosides, although antibiotic treatment has overall not been shown to alter the course [14]. Azithromycin has been shown to decrease long-term carriage of STEC O104:H4 but the clinical implication is not clear [189]. Cryptococcus is an encapsulated, basidiomycetous yeast with worldwide distribution. It is often found in soil, decaying organic matter, and bird guano, including pigeons, turkeys, chickens, and parrots. Antigenic variability is based on the polysaccharide capsule, which is antiphagocytic, depletes complement, produces brain edema, and interferes with antigen presentation. Cryptococcus is composed of two species that consist of five serotypes including: C. neoformans var. grubii (serotype A), C. neoformans var. neoformans (serotype D), hybrid serotype AD, and C. gattii (serotypes B and C) [210,256]. C. neoformans serotype A has been the most common cause of human disease worldwide both before and after the HIV pandemic, predominantly causing meningitis although any organ can be infected, including lung, skin, eye, prostate, adrenal glands, and bone [47,210]. C. gattii has emerged as a pathogen of interest due to a recent outbreak on Vancouver Island, British Columbia, Canada and neighboring states in the Pacific Northwest in 1999. While some patients in this outbreak were immunocompromised, many were otherwise healthy non-HIV infected adults. C. gattii is classified into four discrete molecular types (VGI–VGIV) based on multilocus sequence typing [23,79,135]. The VGII class represents the predominant type in the Vancouver Island outbreak and the Pacific Northwest and falls into three discrete genotypes: major (VGIIa), minor (VGIIb), and a novel, independent strain (VGIIc) [24,79]. The major genotype, VGIIa, was also isolated from a Seattle patient dating back to 1971 suggesting that a recent genetic or environmental change may be driving reemergence. VGIIa represents the predominant strain, causing over 90% of infections on Vancouver Island [134]. VGIIb molecular type is similar to those also found in Australia and is the second most commonly identified genotype from Vancouver Island. In contrast, VGIIc has only been recovered in Oregon and has never been found on Vancouver Island or outside the United States [23,101]. Cryptococcus spp. generally exist as asexual, haploid yeasts that reproduce by budding or haploid fruiting in which the yeast produce basidiospores by meiosis without mating. Infectious propagules are inhaled, deposit in the alveoli, and are taken up by alveolar macrophages where they are either eliminated, remain dormant in the lung-lymph node complex, or proliferate. Previously thought to be exclusive to the laboratory, sexual reproduction via recombination is also possible and increasing evidence suggests this occurs in the natural environment. Two mating types, designated a and α undergo conjugation. Same mating type recombination or monokaryotic fruiting has also been shown to occur and α–α mating may be responsible for the Vancouver Island outbreak and increased virulence [47,79,210,233]. Murine models infected via intranasal instillation with Pacific Northwest C. gattii outbreak isolates, including VGIIa and VGIIc, have demonstrated higher intracellular proliferation capacity, suggesting that the ability to survive and proliferate in host macrophages may contribute significantly to C. gattii virulence. Despite evidence that most isolates from the Pacific Northwest outbreak are exclusively α–α mating, the VGIIc genotype may have resulted from a–α mating, suggesting both mating events are contributing to recombinant genotypes and increased virulence [24,164]. The incidence rate among residents of Vancouver Island was 8.5 cases per million residents in 1999 and increased to 37 cases per million residents in 2003 [47,83,166]. In a preliminary study by Kidd et al. of 38 C. gattii human infections [134], the majority were immunocompetent with lung or central nervous system involvement. During the same time period, a variety of animals were diagnosed with cryptococcal infection, including dogs, cats, ferrets, squirrels, horses, and washed-up porpoises. Birds seem to be protected by their higher body temperature resulting in GI colonization without clinical illness, however, C. gattii has not been detected in bird guano [210]. From 1999 to 2007, a total of 218 cases were reported to the British Columbia Centre for Disease Control. The majority of case-patients were immunocompetent adults living on Vancouver Island, there was no seasonal variation, and the median incubation period was 6–7 months [165]. Most patients presented with a respiratory syndrome consisting of cough, dyspnea, and chest pain accompanied by single or multiple lung nodules. A minority was asymptomatic or presented with cryptococcal meningitis consisting of headache, nuchal rigidity, photophobia, blurry vision, seizure, chills, nightsweats, and weight loss. The VGIIa strain was the predominant genotype, although clinical presentation did not differ by genotype. The case-fatality rate was 8.7% and death was more likely in those presenting with a central nervous system (CNS) syndrome, age greater than 60 years, and in those with an underlying medical condition. Case patients were more likely to have an underlying lung condition, systemic corticosteroid use, an invasive malignancy, or less commonly HIV. No clear environmental exposure was found and case-patients were actually less likely to report outdoor activities [83,101,165,166]. Human-mediated environmental disruption is thought to facilitate aerial dispersal [134]. Beginning in 2004, cases of C. gattii without exposure to Vancouver Island were detected in the British Columbia mainland, Gulf Islands, Puget Sound area, and the US Pacific Northwest. Most isolates were VGIIa and had identical multilocus sequence typing (MLST) profiles to isolates from Vancouver Island [166,260]. One case of the novel VGIIc genotype was also isolated [23,47]. From January 2004 through July 2010, a total of 60 cases were reported to the CDC from California, Idaho, Oregon, and Washington, with the majority having no exposure to British Columbia and at least nine reported deaths due to C. gattii. Half were of the VGIIa subtype but 32% were of the novel VGIIc subtype and most had an underlying chronic illness. By July 1, 2011 a total of 96 Pacific Northwest cases were reported to the CDC, with the majority VGIIa and VGIIc, and 83 cases likely acquired in the Pacific Northwest or from recent travel to British Columbia. The mortality rate did not differ between groups but the overall death rate was higher than previous reports, with 19 deaths (20%), which may be attributable to the increased virulence of VGIIc [101]. Identification of Cryptococcus can be carried out using a variety of methods. White-creamy, mucoid colonies grow readily in 48–72 h on routine agar at 30–35°C. Cryptococcus spp. convert urea to ammonia resulting in a positive rapid urease test and also contain laccase, which converts diphenols (e.g. L-dopa, norepinephrine) into melanin, producing a brown-black color on media containing niger seed, caffeic acid, or dopamine. Cryptococcal antigen testing can be performed on serum and cerebrospinal fluid but does not distinguish species. Further identification to C. gattii can be elicited by growth on L-canavanine, glycine, 2-bromothymol blue (CGB) agar as C. gattii is canavanine-resistant and able to use glycine as a sole carbon source, triggering a bromothymol blue color reaction. Cryptococcus neoformans is canavanine-sensitive and cannot use glycine as a sole carbon source [24,210]. Molecular typing of C. gattii can be accomplished by PCR fingerprinting, MLST, restriction fragment length polymorphism (RFLP), and random amplification of polymorphic DNA (RAPD) [210]. Traditionally, CNS cryptococcal disease is initially treated with combination amphotericin B and flucytosine, while extrameningeal disease is treated with azoles, most commonly fluconazole. In vitro susceptibility studies have demonstrated that older antifungal drugs such as amphotericin B, flucytosine, and fluconazole, while active, had lower levels of activity against C. gattii compared to itraconazole, voriconazole, posaconazole, and isavuconazole. VGII has been found to have significantly higher geometric mean minimum inhibitory concentrations (MICs) for most antifungal agents compared to VGI, with fluconazole and flucytosine having the lowest levels of activity. A study of 43 isolates from the Pacific Northwest typed by MLST showed that 23.3% had fluconazole MICs of 16–32 μg/mL, with VGIIc having the highest geometric mean MIC. The newer triazoles still demonstrate excellent potency against all genotypes, including those with reduced susceptibility to fluconazole [91,111,256]. Relapsing fever is a clinical syndrome often caused by tick-borne and louse-borne (Pediculus humanus) arthropod-zoonoses, more specifically, spirochetes of the genus Borrelia. Relapsing fever is characterized by recurrent bouts of febrile illness associated with spirochetemia. There are over 15 known Borrelia spp. associated with tick-borne relapsing fever (TBRF), which are distributed throughout North and South America, Europe, Africa, and Asia [87,225]. Within North America, most cases occur in British Columbia and the western states such as Washington, California, Colorado, Arizona, New Mexico, Nevada, and Idaho, especially in forested areas, low elevation mountainous areas, limestone caves, lakes, rustic cabins nears bodies of water, and state parks including Grand Canyon National Park [56,57]. Recreational activities such as camping and hiking also increase exposure risk, especially during the spring and summer months [56]. In 1995, Fukunaga et al. described a new Borrelia species in Hokkaido, Japan, using comparative 16S rRNA gene sequence analysis and DNA-DNA hybridization, termed HT31T or B. miyamotoi isolated from Ixodes persulcatus. Relapsing fever borreliae often contain only a single copy of the 23S and 5S rRNA gene compared to two sets in B. burgdorferi, a causative agent of Lyme disease. Further sequencing of the 38-kDa flagellar protein found more similarities to relapsing fever borreliae than Lyme disease borreliae [81]. The capacity for B. miyamotoi to infect humans was not known but would later be elucidated by Platonov et al. in 2011 [211]. Argasid soft-bodied and ixodid hard-bodied ticks transmit Borrelia spp. between reservoirs, which include rodents, mice, rabbits, voles, chipmunks, squirrels, birds, and lizards to human hosts [87,225,236]. All stages of the tick life cycle require blood feeding and are able to transmit the spirochetes between stages (trans-stadial transmission). Borrelia are taken up during nightly blood meals, multiply within the vector, and invade the salivary glands, which facilitates transmission to the host during feeding. Within the host bloodstream, Borrelia multiply and are sequestered resulting in specific antibody production [87,225]. Antigenic variation is the process that accounts for relapsing fever and evasion of the host immune system. Borrelia spp. produce several lipoprotein antigenic variants on the outer membrane called variable small proteins (Vsp) and variable large proteins (Vlp), formerly called variable major proteins (VMP). Encoded by linear plasmids, variation is mediated by recombination on the expression site, which causes a specific host antibody response. The process continues in a cyclical pattern, with high levels of spirochetemia and fever followed by recrudescence [7,8,248]. Further studies have identified B. miyamotoi outside of Japan. From 2001 through 2006 B. miyamotoi has been isolated from a variety of Ixodes ticks, including I. ricinus and I. scapularis in the United States (Connecticut, Rhode Island, New York, New Jersey, California, and Tennessee), Europe (Sweden, Germany, and France), and most recently Russia [21,77,184,211,227,235,236]. Further phylogenetic research indicated that B. miyamotoi forms a monophyletic relapsing fever group closely related to B. lonestari, the causative agent of southern-tick-associated rash illness (STARI) [226]. In 2011, patients in Yekaterinburg City, Russia with clinical symptoms and laboratory-confirmed B. miyamotoi infection were compared to patients with B.garinii in the same area and B. burgdorferi infections in the northeast United States. B.miyamotoi was found in 17% and the cause of borreliosis in almost 25% of the cases in Yekaterinburg City [211]. The mean incubation of TBRF is 7 days, although may be up to 15 days for B. miyamotoi [57,211]. Relapsing fever is characterized by episodes of fever interspersed with afebrile periods that correlate with levels of spirochetemia and the host immune response to lipoprotein antigenic variants. The first febrile period often lasts 3–6 days followed by milder episodes lasting up to 3 days and may be accompanied by fatigue, headache, or myalgias, and less commonly chills, arthralgias, neck stiffness, abdominal pain, nausea, vomiting, or diarrhea [57,211]. Intervening afebrile periods often last 4–14 days [57]. Severe symptoms occur more commonly with untreated louse-borne relapsing fever (LBRF) and may include jaundice, central nervous system or ocular involvement, thrombocytopenia, hematuria, bleeding diatheses, and death. Patients may have multiple relapses before seeking medical attention and many relapse under the care of a medical provider due to lack of illness recognition [56]. Those infected with B. miyamotoi tended to have a longer hospital length of stay and a higher incidence of systemic symptoms. Erythema migrans (EM) was substantially more common in patients with B. garinii and B. burgdorferi, although a low percentage of patients infected with B. miyamotoi may have EM likely secondary to co-infection. Proteinuria and elevated transaminase levels were slightly more common among patients with B. miyamotoi but without clinical features [211]. Diagnosis is often made clinically in the appropriate endemic setting and epidemiologic exposure. Spirochetes are motile, helical bacteria less than 25 μm in length, with an inner and outer cell membrane and one or more flagella. While dark-field microscopy, Wright stain, or Giemsa stain of thin and thick blood smears can be performed during spirochetemia, the yield is often low. Wet mounts can also be employed, although the yield is also poor and, as with other methods of direct visualization, is operator dependent and may depend on the laboratory’s level of experience. Xenodiagnosis, whereby mice are inoculated with infected blood to amplify the spirochetes to a detectable level, is reserved mainly for research purposes [57].While specific media are available for cultivation of blood specimens, including Kelly’s medium or Barbour–Stoenner–Kelly (BSK) medium, the overall yield is poor and may require up to 2 weeks of cultivation with regular examination under dark-field microscopy [8]. The yield of culture may be increased if collected prior to the administration of antibiotics [56]. Serologic confirmation through the indirect IFA or ELISA confirmed by Western blot is more commonly employed but is limited to relatively few diagnostic laboratories. A fourfold or greater rise in antibody titer between acute and convalescent sera, a single elevated convalescent serum, or a single acute IgM can be diagnostic although false positives between other spirochete species can occur. Serology is also limited by antigen variation in Borrelia spp. and the lack of well-defined, specific antibodies [56,57,87,225]. Polymerase chain reaction has been helpful to identify Borrelia to the species level but detects both viable and nonviable spirochetes and should be applied to the clinical context [87,225]. There is little evidence of acquired antimicrobial resistance among Borrelia spp. Tetracyclines and penicillins remain the treatment of choice. The most commonly used treatments include doxycycline 100 mg twice daily and tetracycline 500 mg four times daily. Alternatively, erythromycin 500 mg four times daily or ceftriaxone 1–2 g daily can be used for children, pregnant women, or those intolerant to tetracyclines [57,225]. The Jarisch–Herxheimer reaction (JHR) is well described following the initiation of treatment for spirochete illnesses including relapsing fever. The JHR often occurs within 1–4 h after initiation of treatment due to rapid spirochete death and release of inflammatory mediators, resulting in a massive cytokine cascade. Symptoms may include tachycardia, fever, chills, diaphoresis, and severe cases can progress to cardiovascular collapse and death [57]. Close observation should be undertaken during the first hours after treatment initiation. The incidence rate in a study by Platnov et al. in those with TBRF due to B. miyamotoi was 15% [211]. Prevention of TBRF includes measures to decrease tick and rodent burdens in common dwellings. Proper construction of homes and cabins with well-sealed walls and roofs reduces the rodent habitat. Avoiding infested areas such as burrows and caves, wearing long pants and long-sleeved shirts, insect repellants, and permethrin-coated clothing are also mechanisms to reduce acquisition of tick bites. Species of Rickettsia are intracellular Gram-negative bacteria that are almost always associated with arthropods. Human disease caused by Rickettsia has long been recognized. Although the causative organism was not known, epidemic typhus was recognized as a distinct clinical entity as early as the 16th century. New species of Rickettsia are continuously being identified as causes of emerging infectious diseases in humans [219]. Since 2002, six species of Rickettsia have been newly recognized to cause disease in humans: R. aeschlimannii [217], R. parkeri [199], R. heilongjiangensis [179], R. massilae [270], R. raoultii [204], and Rickettsia 364D [239]. This discussion will focus on R. parkeri
Emerging Infectious Diseases
21.1 Introduction
21.2 Plasmodium knowlesi
P. malariae
P. knowlesi
Host erythrocyte
size
Not enlarged
Not enlarged
shape
Rounded, not distorted
Rounded, generally not distorted
stippling
Ziemen’s stippling under certain staining conditions
Irregular dots or stippling in some erythrocytes with mature trophozoites, schizonts and gametocytes
Parasite
early trophozoite (ring form)
Ring form with dense cytoplasm and single chromatin; sometimes with accessory dot
Ring forms are compact with dense cytoplasm: single or double chromatin and rarely triple chromatin; appliqué forms: multiple parasites in single erythrocyte
late trophozoite
Regular and compact cytoplasm: appearance of pigment
Dense and thick cytoplasm; cytoplasm slightly amoeboid and irregular: band forms; varying pigmentation
mature trophozoite
Compact, rounded, heavily pigmented: band forms: not amoeboid
Compact and dense cytoplasm, rounded shape with dark brown pigment: band forms: not amoeboid
Schizont
Occupies whole erythrocyte. Contains 6–12 merozoites, usually 8: merozoites clustered around dark-brown malaria pigment forming rosette pattern
Occupies whole erythrocyte; contains up to 16 merozoites; merozoites irregularly scattered or grape-like cluster; malaria pigment scattered or collects into a single mass
Gametocyte
Round, compact. Occupies whole erythrocyte, scattered malaria pigment: early forms are very similar to mature trophozoites
Round, compact. Fills whole erythrocyte. Scattered or clumped malaria pigment: early forms are very similar to mature trophozoites
21.3 Clostridium difficile
21.4 Pandemic H1N1 influenza
21.5 Escherichia coliO104:H4
21.6 Cryptococcus gattii
21.7 Borrelia miyamotoi
21.8 Rickettsia parkeri
Stay updated, free articles. Join our Telegram channel

Full access? Get Clinical Tree
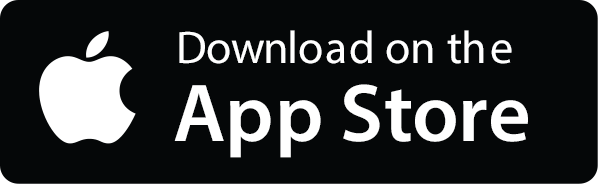
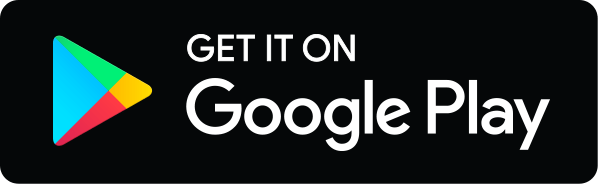