CHAPTER 20 CHAPTER OUTLINE Stress can be defined as any influence, arising either internally or externally to the body, that threatens to disrupt normal structure, function or behaviour. Not surprisingly, complex mechanisms have evolved to protect the body against such threats, whether physical or psychological. It may be surmised that during man’s evolution, the most important stresses were external, e.g. attack and lack of food, water or shelter. For many people, such factors are now of little or no relevance, but others, for example psychological stresses, have become more prevalent; stressors such as trauma and infection remain universal. The body’s response to stress tends to be qualitatively similar whatever the stress, although the severity of the stress affects the response quantitatively, albeit with considerable intra- and interindividual variation. It is reasonable to suppose that the development of the metabolic response to stress was subject to evolutionary pressures, and that the response is potentially beneficial. For some aspects this clearly is the case, but, as will become apparent, some aspects of the response to severe stressors, particularly sepsis, appear potentially harmful to the body as a whole. One can only speculate how this might have come about. The metabolic response to stress is complex, and includes processes that act to propagate the response as well as to curtail it and thereby limit its extent, both physiologically and anatomically. Conventionally, stressors are divided into four categories: 1. physical, e.g. heat, cold, immobilization and pain 2. psychological, e.g. anxiety and fear 3. social, e.g. bereavement, marital breakdown 4. cardiovascular and metabolic, e.g. exercise, haemorrhage and infection. While this system helps categorize the nature of the stimulus, multiple stressors can be applied to an individual at any one time and these, in turn, can be either acute or chronic in nature. Stress responses are intended to be short lived and self-limiting, but are proportional to the intensity of the stimulus and, consequently, can range from simple localized reactions to complex processes. Systemic responses involve vascular, endocrine and metabolic changes orchestrated by the hypothalamo–pituitary axis and sympathetic nervous systems. Centrally, there is facilitation of arousal, inhibition of vegetative functions (feeding and reproduction) and activation of counter-regulatory feedback loops. Respiratory and heart rates increase and there is an increase in vascular tone, resulting in a rise in blood pressure and hence oxygen and nutrient supply to the brain, heart and skeletal muscles. The intensity of stress may be gauged by peak concentrations of stress hormones and neurotransmitters, by physiological changes, such as increases in heart rate and blood pressure, and by the length of time for which these changes persist during stress and following the cessation of stress. Whilst intended to confer a survival advantage, the stress response can become part of the pathological process. In severe stress, responses that appear potentially advantageous if limited in extent (e.g. mobilization of muscle protein to provide glucose as an energy source) become harmful. Figure 20.1 summarizes the complexity of the systemic responses to potential stimuli. Stimulation of the hypothalamus and locus coeruleus leads to the release of pituitary hormones and activation of the sympathetic nervous system. The locus coeruleus, hypothalamus and brainstem are closely linked, both anatomically and functionally. The locus coeruleus and hypothalamus mutually innervate and stimulate each other. This positive feedback system allows for activation of the systemic stress response by any stimulus that initiates either side of this loop. The locus coeruleus is located in the lateral floor of the fourth ventricle, and represents the major pool of noradrenaline (norepinephrine) secreting neurons in the brain. It receives afferents from many areas, including the hypothalamus, the cerebellum, prefrontal cortex and the hypoglossi. The cingulate gyrus and amygdala also innervate the locus coeruleus, transmitting emotional and pain stressors. Nerve fibres from this nucleus innervate the spinal cord, brainstem, thalamic relay nuclei, cerebellum and hypothalamus and are predominantly excitatory in action. Adrenaline (epinephrine) secreting nerve terminals are also found within the locus coeruleus and are thought to represent part of the medullary regulatory circuit. At the centre of the limbic system, the hypothalamus controls most of the vegetative (homoeostatic) and endocrine functions of the body. Combined with the locus coeruleus, it is the major effecter of the stress response. The efferent neuronal pathways from the hypothalamus can be classified into two groups: direct monosynaptic projections to pre-ganglionic neurons in the lower brainstem and spinal cord projections to brainstem catecholaminergic neurons in the medulla that innervate neurons in the thoracic spinal cord. Additionally, hypothalamic nuclei participate in other regulatory pathways, such as temperature control, energy homoeostasis and body fluid composition. Stimulation of the paraventricular nucleus by afferent fibres from the limbic system and lower brainstem results in the secretion of corticotrophin-releasing hormone (CRH), into the primary capillary plexus of the hypophyseal portal system, from where it is transported to the anterior pituitary gland. The hypothalamus also secretes the polypeptide arginine vasopressin (AVP), which is transported along nerve tracts to the posterior pituitary. Under resting conditions, CRH and AVP are secreted in a circadian and highly concordant pulsatile fashion: stressors promote a surge in AVP secretion. Vasopressin increases water retention in the kidneys, is a potent vasoconstrictor, acts as a neurotransmitter and has the ability to modulate a number of physiological processes including gluconeogenesis, platelet aggregation and inflammation. Corticotrophin-releasing hormone and AVP act synergistically on the anterior pituitary to induce synthesis of the large preprohormone, proopiomelanocortin (POMC). The POMC molecule is processed to form a series of smaller peptides, the nature of which is dependent on the enzyme systems present within the tissue type. Anterior pituitary corticotrophin cells express prohormone convertase 1 (PC1), which cleaves POMC into ACTH, N-terminal peptide, joining peptide and β-lipotropin. In the hypothalamus the enzyme PC2 cleaves POMC to α-, β- and γ-melanocyte stimulating hormones and β-endorphin. α-Melanocyte stimulating hormone acts on melanocortin receptors in the paraventricular nucleus of the hypothalamus, stimulating the sympathetic nervous system. Adrenocorticotrophic hormone and the other peptide cleavage products of POMC have a negative feedback effect on the hypothalamus to limit CRH release. While CRH and AVP act synergistically on the anterior pituitary, only small amounts of ACTH are secreted in the absence of CRH, which is pivotal to an integrated stress response. Following stimulation by ACTH, cortisol is the principle glucocorticoid produced by the adrenal cortex, accounting for 95% of glucocorticoid activity. Approximately 90% of circulating cortisol is protein bound; some is attached non-specifically to albumin, but the majority binds with high affinity to cortisol-binding globulin (CBG); only free cortisol is biologically active. Cortisol-binding globulin is an α2-globulin, produced in the liver, which behaves as a negative acute phase protein. The concentration of CBG falls in response to some inflammatory stimuli, increasing the bioavailability of cortisol. Cortisol is a major effector of the metabolic response to stress. It is lipid soluble and readily crosses cell membranes, its activity being mediated via the glucocorticoid receptor (GR). Two isoforms of GR have been isolated, GRα, which binds cortisol and the GRβ, which does not bind cortisol and may act to inhibit the effect of endogenous glucocorticoid action. The hormone receptor complex is translocated to the nucleus where it interacts with glucocorticoid response elements and, following recruitment of co-activators, leads to increased gene transcription. Other actions are DNA independent and involve direct interactions with transcription factors, such as nuclear factor-κβ (NF-κβ), This is important in the modulation of the stress response, since NF-κβ activates the expression of interleukin-1(IL-1), IL-6 and tumour necrosis factor α (TNFα), all of which are key components of the inflammatory response and interact with the hypothalamo–pituitary–adrenal axis (HPAA). Cortisol exerts a negative feedback effect on CRH and ACTH release, affects carbohydrate, protein and fat metabolism, and modulates the immune response. Cortisol limits glucose utilization in cellular respiration, perhaps by reducing the oxidation of NADH to NAD+ in the tricarboxylic acid cycle. High concentrations of cortisol reduce the sensitivity of peripheral tissues to insulin. This anti-insulin effect is particularly marked in skeletal muscle and adipose tissue, reducing glucose uptake and metabolism. In extra-hepatic tissues, there is increased protein catabolism and decreased protein synthesis through reduced RNA formation and amino acid uptake. Within the liver, however, this metabolic picture is reversed, with increased amino acid uptake and the synthesis of acute phase proteins. Additionally, cortisol enhances the mobilization of fatty acids from adipose tissue, shifting oxidative metabolism to favour energy generation from glucose to fatty acids. Major disturbances of thyroid hormone metabolism and plasma concentration occur during the metabolic response to stress, typically with falls in the concentrations of tri-iodothyronine and often of thyroxine and, sometimes, in the critically ill, a fall in the concentration of thyroid stimulating hormone. (These changes are discussed further in Chapter 19.) The effects of trauma and sepsis on sex hormones are complex and dependent on the type of injury. Plasma concentrations of free testosterone may fall without any consistent change in the concentration of luteinizing hormone (LH), suggesting a change in the sensitivity of Leydig cells to LH or a disturbance of normal feedback regulation. The effects tend to be more marked in women, with reduced activity of the entire hypothalamo–pituitary–gonadal axis. Corticotrophin releasing hormone is known to have inhibitory effects at various levels in this axis, including antagonizing the effects of LH on Leydig cells. Chronic activation of the hypothalamo–pituitary–gonadal axis, such as has been demonstrated in long distance runners and ballet dancers, causes suppression of gonadal function in males and females. The anterior pituitary secretes growth hormone, stimulated by growth hormone releasing hormone, produced by the ventral medial nucleus of the hypothalamus. Growth hormone is a small protein that is active in most cells of the body, where it enhances amino acid uptake and protein synthesis. Under the influence of growth hormone, free fatty acids are used as an energy source in preference to carbohydrates and proteins. Growth hormone reduces glucose uptake by skeletal muscle and fat cells, promotes gluconeogenesis by the liver and consequently increases insulin secretion by the pancreas. Activation of the locus coeruleus by stressors causes the release of noradrenaline and increased sympathetic discharge via the brainstem and spinal cord. Pre-ganglionic sympathetic nerves pass through the sympathetic chain to innervate modified neuronal cells in the adrenal medulla. These cells secrete noradrenaline and adrenaline into the circulation. Catecholamine synthesis is an energy-dependent process with many mitochondria packing the terminal varicosities containing the secretory vesicles. Tyrosine is converted to L-DOPA and then to dopamine, which is transported into the secretory vesicles where a hydroxylation reaction converts it into noradrenaline. The majority of noradrenaline is then metabolized to adrenaline. The sympathetic nerve impulse to the adrenal medulla results in the influx of intracellular calcium and the discharge of the catecholamine secretory vesicles (in the ratio of 80:20, adrenaline:noradrenaline) directly into the circulation. The combined effect of this circulating adrenaline and noradrenaline is identical to that caused by direct sympathetic nervous system stimulation, but has a longer duration and can reach all cells of the body irrespective of their autonomic innervation. Fluid conservation occurs as part of the stress response, often resulting in low urine outputs despite euvolaemia. Increased sympathetic nervous system activity, combined with raised concentrations of circulating catecholamines, reduces renal blood flow. This initiates the metabolism of prorenin to renin in the juxtaglomerular cells of the kidneys. Renin is released into the blood where it acts on angiotensinogen to form angiotensin I. Under the influence of angiotensin-converting-enzyme, predominantly in the lung, angiotensin I is converted to angiotensin II, a potent vasoconstrictor. Angiotensin II stimulates AVP release from the hypothalamus, as well as having a direct affect on the kidney to increase salt and water retention. Furthermore, under the influence of angiotensin II, the mineralocorticoid aldosterone is synthesized in the zona glomerulosa of the adrenal cortex, by metabolism of corticosterone by the enzyme aldosterone synthase. Whilst ACTH has a permissive role in aldosterone synthesis, it does not control the rate of release. Aldosterone acts on the cortical collecting tubules where it stimulates a Na+,K+-ATPase, leading to the retention of sodium and water. (Salt and water balance is considered further in Chapter 4 and renal function in Chapter 7.) Cytokines are peptides secreted by many cell types including mast cells, macrophages and endothelial cells. They are released into the extracellular fluid and can function as autocrine, paracrine or endocrine hormones and they trigger the acute phase response.
Metabolic response to stress
INTRODUCTION
THE RESPONSE TO STRESS
Initiation of the stress response
Hypothalamo–pituitary–adrenal axis
Cortisol
Thyroid hormones
Sex hormones
Growth hormone
Adrenal medulla
STRESS AND THE KIDNEYS
CYTOKINES
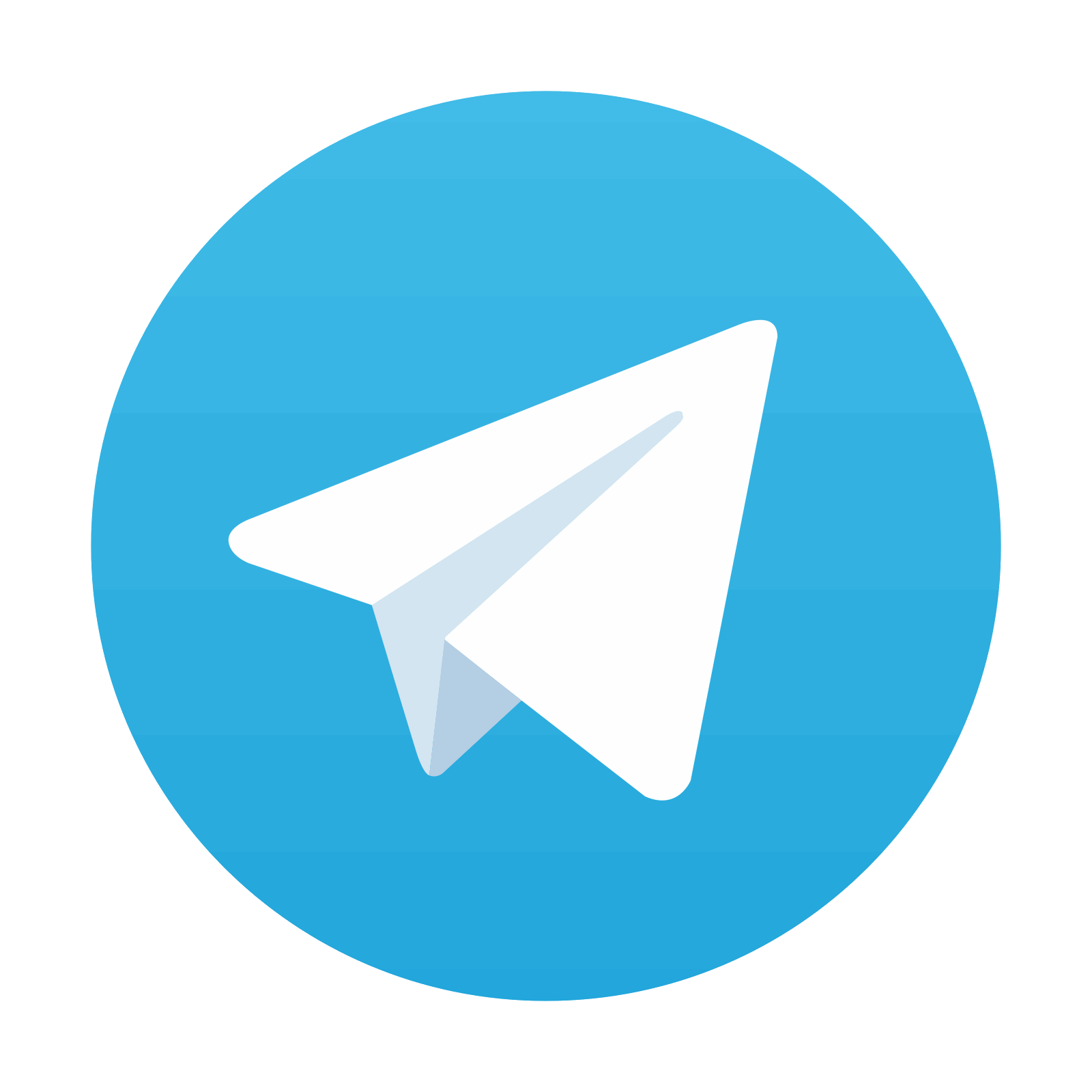
Stay updated, free articles. Join our Telegram channel

Full access? Get Clinical Tree
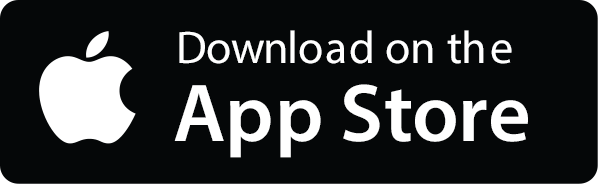
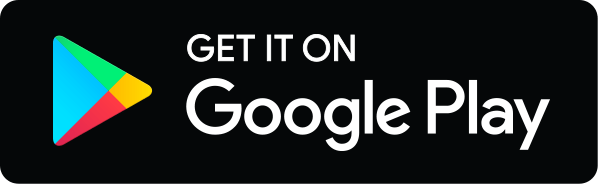