CHAPTER 19 Thomas J. Inzana1, Xiang-Jin Meng1, Tanja Opriessnig2, and Lora Ballweber3 1 Virginia Tech, Blacksburg, VA, USA 2 Iowa State University, Ames, IA, USA 3 Colorado State University, Fort Collins, CO, USA The field of clinical veterinary microbiology is becoming more standardized and adopting many of the tests and protocols that are commonly used in human clinical microbiology. Standard protocols for antimicrobial susceptibility testing of animal pathogens continue to be revised and advanced by the Clinical Laboratory Standards Institute and now include: These are available from http://shop.clsi.org/veterinary-medicine-documents/ The One Health Initiative is a collaborative effort designed to unite human and veterinary medicine, and recognizes that zoonotic infections are becoming more common and have a greater impact on human health than ever before. Factors that compromise the immune system (advanced age, HIV and other chronic infections, chemotherapy, etc.) have resulted in animal pathogens or commensals being isolated from human specimens more commonly. Currently, 39% of US households own at least one dog and 33% of US households own at least one cat (http://www.humanesociety.org/issues/pet_overpopulation/facts/pet_ownership_statistics.html). Furthermore, 56% of American households own some type of pet (https://www.avma.org/KB/Resources/Statistics/Pages/Market-research-statistics-US-pet-ownership.aspx). Therefore, there is a greater demand for diagnostic services related to animal health care. While zoonotic agents are more commonly being isolated from human patients [37], anthropophilic infections are also more commonly being seen in pets (e.g. methicillin-resistant Staphylococcus aureus in dogs). Agents that are currently recognized as responsible for emerging infectious diseases are also often of zoonotic origin or use animals as intermediate hosts [87,101,108], and many of the select agents are zoonotic or animals are their primary host. Therefore, human and veterinary clinical and diagnostic laboratories need to be capable of identifying and determining the antibiotic susceptibility of microbial pathogens of animal origin. Although the demand for the accurate identification of animal pathogens is increasing, the capability of commercial tests to identify these pathogens has not kept up with demand. The lack of commercial tests for these agents reflects the consumer cost of such tests, which is usually the full responsibility of the owner, as opposed to insurance. Such costs are most restricted in diagnostic and medical expenses for food animals because any disease intervention is based on the cost-benefit of the animal or animals (a disease that is likely to affect a large number of animals in the herd warrants more attention to treatment than that of only an individual animal). Because many of the pathogens isolated from animals are identical or similar to those isolated from humans, veterinary diagnosticians will often use products made for human clinical microbiology. Most of the fungi responsible for animal infections are essentially identical to and cause the same diseases as those causing human infections. The enteric bacterial pathogens that cause infections in animals are also either identical or of the same genus. Many of the bacteria that colonize or infect host mucosal surfaces (e.g., Bordetella, Haemophilus, Chlamydia species, etc.) differ only at the species level. Parasites isolated from animals may either be similar or identical to those isolated from humans, or more specific to a host species. However, the viruses that commonly cause infections in animals are usually the most host-specific. To address the need for identification of many animal-specific pathogens, many academic and state animal diagnostic laboratories offer “in-house” tests to identify these agents, although these tests are largely unregulated. Currently, most of these tests are based on polymerase chain reaction (PCR) and other molecular assays, or on serology. The American Association for Veterinary Laboratory Diagnosticians (AAVLD) voluntarily inspects and accredits laboratories offering veterinary diagnostic services. Currently, there are 45 public veterinary diagnostic laboratories that are AAVLD-accredited in the United States and Canada. AAVLD accreditation is designed to assist laboratories to meet or exceed the standards of the World Organization for Animal Health (OIE Quality Standard and Guidelines for Veterinary Laboratories: Infectious Diseases, 2008). In this chapter, due to the scarcity of commercial diagnostic tests specific for many animal pathogens, reviews of how well tests designed for human pathogens perform to identify related species are reported, as well as diagnostic services that are provided by veterinary laboratories for specific agents. Tests that are primarily used in human clinical medicine and are highly relevant to use in veterinary medicine may be mentioned, but the reader will be referred to other chapters in the text that describe these tests in more detail. Recommendations on the collection and transport of veterinary clinical specimens are also described. The successful detection of microorganisms by the laboratory depends critically on the proper collection and transportation of the clinical sample by the submitting veterinarian. In general, care should be taken to protect the agent in specimens from environmental damage and maintain viability by using the proper transport system [70]. The materials intended for diagnosis should be collected as soon as possible after the onset of clinical disease, and in a manner to avoid contamination. The samples collected during the acute phase of infection usually contain adequate amounts of organisms, which are often readily detectable with available assays. Samples collected at later times, such as the convalescent phase of infection, may lead to false-negative results even though a microorganism was the cause of the disease. Certain viral infections can predispose the host to secondary viral or bacterial infections [115,165]. Therefore, samples collected late in the disease process may lead to a misdiagnosis when infection with secondary pathogens is involved. The types of samples to be collected are also important, but will largely depend upon the diagnostic tests selected and the suspected agents or clinical diseases manifested. The knowledge of the pathogenesis of a particular disease by the submitting veterinarian is important for the selection of suitable clinical samples. In general, the appropriate samples for viral or bacterial detection are nasal swabs, body fluids, fecal, and blood samples from infected, live animals, and relevant tissues and organs from necropsied animals. More specifically, it is recommended to collect nasal or nasopharyngeal swab materials from animals with respiratory diseases; fecal samples from animals with enteric diseases; cerebrospinal fluid, fecal samples, and nasal swab materials from animals with central nervous system signs; genital swab materials from animals with genital diseases; conjunctival swab materials from animals with eye diseases; and vesicle swab materials and biopsy samples from animals with skin lesions [37,87,108]. More recently and especially for swine, alternative noninvasive sample types have become popular. For example, pen-based oral fluid samples, using three-strand twisted cotton ropes that hang into the pens [127], are collected on a routine basis in many large swine operations. The pigs are usually allowed to chew on the ropes for about 30 min and the absorbed oral fluid is later mechanically extracted from the ropes and used for diagnosis [126]. As a general practice, blood samples should always be collected from animals with suspected microbial diseases. Whole blood should not be frozen, as this will cause complete hemolysis and render the samples unsuitable for serological diagnoses. The whole blood should be allowed to clot at room temperature, and the serum and buffy coat cells separated by centrifugation. In most cases, paired serum samples collected several weeks apart are needed for a definitive diagnosis of a microbial disease. Cross-sectional blood collection from animals manifesting different stages of the disease is also an alternative and often very effective diagnostic approach for farm animals housed in larger groups. A fourfold or greater increase in serum antibody titer to a particular agent is indicative of current infection associated with the suspected microorganism. However, a very high single titer to a specific pathogen that is supported by additional testing is also of diagnostic value. The amount of clinical material collected is also important for a definitive diagnosis. Whenever possible, adequate amounts of the clinical samples should be obtained because insufficient amounts of the sample could lead to an inconclusive or false-negative result. Many organisms are labile and could quickly be inactivated if stored or transported improperly. Nonbleached swabs should be used to collect swab materials in order to avoid toxicity to either the microorganism or to cell cultures [70]. Samples collected for the detection of most microbial agents should be kept cold and moist, and in the appropriate transport medium for that agent. For viruses, the medium usually contains a buffered salt solution, proteins to prevent virus inactivation, and antibiotics to prevent bacterial and fungal growth. A variety of transport media are suitable for virus transport [70]. Commercial swabs (such as from Baxter, Deerfield, IL) or transport systems (such as from Becton, Dickinson and Company, Franklin, NJ) are available for collecting, storing, and transporting clinical materials containing infectious agents. Samples intended for bacterial identification should be collected separately in transport medium that does not contain antibiotics. Clinical materials intended for microbial identification should be refrigerated at 4°C after collection and transported to a diagnostic laboratory as soon as possible. During transportation, samples should be carefully packed in an appropriate shipping container with an adequate amount of commercial refrigerant packs. Care should be taken to prevent any potential leakage and safety hazards. Most microorganisms are stable at 4°C for up to 2–3 days [70]. However, if samples cannot be submitted in a timely manner, clinical materials should be immediately frozen at −70°C. Storage of samples intended for virus detection at −10°C to −20°C, such as the freezer sections of standard refrigerators, is detrimental and should be avoided as most agents rapidly lose infectivity when stored at this temperature due to water crystal formation [37,101]. Frozen samples should be transported to a diagnostic laboratory on dry ice. Special import and export permits are required for international and some domestic transportation of infectious materials, and appropriate authorizations from respective countries should be obtained prior to transportation. Interstate transportation of certain veterinary organisms within the United States also requires a permit from the US Department of Agriculture’s Animal and Plant Health Inspection Service (4700 River Road, Riverdale, MD 20737; phone: 301-734-8695; http://www.aphis.usda.gov/permits/). Readers are referred to published federal guidelines and regulations (Title 42 CFR Part 72; Title 49 CFR Part 173.386-388) for details regarding packaging, labeling, and interstate shipping of infectious agents. Proper labeling of samples and recording relevant clinical information prior to submission is as important as sample collection and transportation. Each veterinary diagnostic laboratory has its own standard sample submission form, which is usually available through the diagnostic laboratory’s web site. Submitting veterinarians are usually asked to provide information such as animal species or source, age, body weight, sex, herd or flock size, number of animals in the herd or flock affected or dead, location of animals, major clinical signs, date of disease onset, date of samples collected, presumptive clinical diagnosis, vaccination history, types and numbers of samples submitted, and type(s) of diagnostic tests requested. The clinical information, especially the presumptive diagnosis, is important for laboratory diagnosticians not only to select the most appropriate and sensitive tests for the submitted specimens, but to evaluate and interpret the test results as well. Most states in the United States have their own veterinary diagnostic laboratory system, many of which are associated with their respective veterinary school. Each of these laboratories usually specializes in one or more areas such as poultry diseases, food animal diseases, fish diseases, companion animal diseases, and laboratory animal diseases. A few national laboratories such as the US Department of Agriculture’s National Veterinary Service Laboratories (NVSL) have the capacity to provide more comprehensive diagnostic services and reagents. NVSL-Ames (1920 Dayton Avenue, Ames, IA 50010; phone: 515-337-7266; fax: 515-337-7397) provides reagents and comprehensive diagnostic services for most animal diseases. NVSL-Foreign Animal Disease Diagnostic Laboratory (FADDL) has the capacity of diagnosing animal diseases that are foreign to the United States (NVSL-FADDL, P.O. Box 848, Greenport, NY 11944-0848; phone: 515-323-3256; fax: 631-323-3366). The selection of a particular diagnostic laboratory depends largely on the submitting veterinarians’ preference, test availability, animal species involved, and type of disease suspected. Most veterinarians tend to use local or regional diagnostic laboratories for convenience, but such preference is sometimes limited by the availability of certain tests at local laboratories. For lists of available diagnostic tests from veterinary diagnostic laboratories in the United States, readers are referred to nearby veterinary schools for locations of reference laboratories and their available diagnostic services. Each laboratory usually has a list of diagnostic services that are available on their diagnostic laboratories web site (see Appendix). Selection of a diagnostic test for an infectious agent depends on many factors, such as the clinical specimens available for submission, the type of disease suspected, test availability, and cost consideration. For a given disease, multiple diagnostic tests may be available. The principles and selection of these tests are discussed below. In many cases, a confirmatory test is also performed on the same sample or different samples from the same diseased animal. Many traditional tests and procedures are still important in the diagnosis of veterinary microbial diseases, such as pathological and histopathological examination. However, electron microscopy and virus isolation are being used less frequently for diagnosis of viral diseases. Many modern diagnostic tests are rapid assays [58,67,87,108] aimed at detection of antimicrobial antibodies (enzyme-linked immunosorbent assay (ELISA), Western immunoblot analysis, immunofluorescence, serum virus neutralization, hemagglutination inhibition, agar gel immunodiffusion, complement fixation), detection of antigens (immunohistochemistry, antigen-capture ELISA, frozen tissue immunofluorescence), or detection/amplification of nucleic acids (PCR and its related techniques, in situ hybridization, Southern blot hybridization, dot blot hybridization). In addition, modern molecular tests and procedures (such as PCR-restriction fragment length polymorphism, sequencing and sequence analysis) are being used more commonly for further genetic characterization of viruses and highly fastidious bacteria, and for differential diagnosis. The basic principles and general application of the commonly used laboratory procedures and diagnostic assays in veterinary diagnostic microbiology are discussed below. Pathology and histopathology form integral parts of laboratory procedures in veterinary diagnostic laboratories. Veterinary pathologists play an important role in the diagnosis of microbial diseases, especially in the identification of previously unrecognized agents. Live diseased animals with acute clinical signs and dead animals with no postmortem autolysis can be submitted for pathological evaluation. Specimens submitted for histopathological examination should be fixed in 10% neutral-buffered formalin. Veterinary pathologists can evaluate fresh tissues for the presence of macroscopic lesions and formalin-fixed tissues for microscopic lesions. Proper selection and preservation of samples by the submitting veterinarian is critical for histopathological examination. Generally, samples submitted for histopathological examinations should be fresh slices (about 0.5 cm) of affected organs fixed in 10% neutral buffered formalin. The organ slices should include the lesion and transitional areas, as well as the adjacent normal tissues. Samples from multiple organs should be collected and submitted in case the submitting veterinarian is unsure of the organ type to be sampled, and this will allow the veterinary pathologist to select the most appropriate samples for examination. Based on characteristic lesions, the veterinary pathologist can make a tentative diagnosis or come up with a differential list of potential pathogens, which can then be confirmed by laboratory diagnostic tests. Accurate diagnosis of a veterinary infectious disease is critically important for effective and timely treatment of diseased animals, for prevention of disease spread within and between herds, and, in cases where the microorganism may have zoonotic potential, for prevention of disease spread to humans. The evaluation and interpretation of test results is largely dependent upon the sensitivity and specificity of a diagnostic test [21]. In general, the diagnostic test must be sensitive enough to detect its target at an acceptable detection threshold (analytical sensitivity) and minimize false-negative results (diagnostic sensitivity). The test must also be specific enough to minimize nonspecific detection resulting in false-positive results. Factors affecting the specificity and sensitivity of a diagnostic test may include the purity of the reagents, the cutoff values used for immunoassays, the quality of clinical samples, the instrument used, and the technical skill of the technicians. The sensitivity and specificity also vary from test to test. Therefore, the selection of a particular diagnostic assay for a given organism is important. A confirmatory test, if available, may also need to be performed. The commercial diagnostic kits in veterinary use have set sensitivities and specificities that are standardized from lot-to-lot, thereby rendering quality control relatively easy. Since fewer commercial kits are available for veterinary use than for human diagnostics, veterinary diagnostic laboratories frequently use in-house assays in which the sensitivity and specificity may not be known. Each laboratory usually follows its own standard for quality control, evaluation, and interpretation of test results. Therefore, reproducibility of test results from laboratory to laboratory is often not known for the in-house assays. The specificity and sensitivity of a test can usually be improved by including adequate positive and negative controls for each run of specimens. Even for commercial kits that come with standard controls, inclusion of additional in-house positive and negative controls is still necessary for quality assurance. In addition to the routine quality control procedures for a clinical microbiology laboratory, extra care must be taken in a molecular diagnostic laboratory setting. Many factors may affect the quality control of a molecular diagnostic test such as routine calibration of pipettors, the temperatures and ramping times of thermal cyclers, and monitoring for accurate temperatures of water baths, heating blocks, and incubators [112]. To minimize cross-contamination, supplies and reagents intended for PCR use should be delivered directly to a designated area of a laboratory, and stored in a separate refrigerator or freezer. Nonspecific amplification due to carry-over or cross-contamination in PCR-related methods can be significantly reduced by performing procedures (such as reagent preparation, RNA extraction, cDNA synthesis, and PCR assembly) in a laboratory that is physically separate from the one in which PCR products are analyzed. Due to its low sensitivity (usually > 105–106 virus particles/mL of sample are required), high cost of equipment purchase and maintenance, necessity of a skilled technician, and long turn-around time, electron microscopy (EM) has generally lost its importance as a diagnostic tool for virus detection in veterinary medicine, although it affords an opportunity for direct visualization of viruses in clinical materials [116]. Nevertheless, EM is still useful for the identification of viruses that cannot be propagated in vitro, for identification of viruses from clinical samples that are toxic to cell cultures (such as urine, feces, and semen), or for further characterization of suspected viruses. Based on the morphological properties of viruses, a skilled diagnostic virologist can readily identify the virus family it belongs to, which can help make a tentative diagnosis or establish a differential list of potential pathogens. However, a definitive diagnosis usually cannot be made since EM is generally considered a nonspecific test. Samples appropriate for EM examination include, but are not limited to, secretions, excretions, thin tissue sections, vesicular fluids, and cell culture materials. Negative staining is the most commonly used EM technique for viral detection. Viruses present in clinical materials have little contrast with their surroundings. The negative staining technique overcomes this problem by staining the surrounding background with a heavy metal stain such as phosphotungstate or uranyl acetate to increase the contrast between viruses and the background. After negative staining, the structural outlines and surface projections of viruses can be visualized using an electron microscope. Negative staining EM is useful for detecting enteric viruses [12,19,23,28,34,96,137]. As mentioned above, EM generally can detect, but cannot definitively identify, viruses. Additional tests such as immuno-EM and immunogold-EM are usually required for a definitive diagnosis. Negative staining immuno-EM is achieved by adding an antiviral antibody specific to the suspected virus in the clinical specimen. The antibody will aggregate the virus and form virion–antibody complexes that can be visualized by negative staining EM. For immunogold-EM, gold (5-nm particles)-labeled protein G (or protein A) is usually added to the virion–antibody mixture. The gold-labeled protein G (or protein A) binds to the Fc fragment of the antibody. The gold-labeled virus particles are pelleted by centrifugation, washed to remove unbound gold particles, sprayed on EM grids, and visualized using an electron microscope. The immunogold EM technique has been used for the identification of many viruses of veterinary importance such as porcine rotavirus [94], porcine reproductive and respiratory syndrome virus [94,103], and bovine coronavirus [55]. Virus isolation (VI) followed by virus identification as a diagnostic tool is usually slow and laborious, but is still considered the gold standard in veterinary viral diagnosis. Virus isolation is still used in veterinary diagnostic laboratories to recover virus isolates from various diseases for further genetic characterization or autogenous vaccine production. Virus isolation is particularly useful for isolation of viruses from previously unrecognized diseases, for detection of viruses for which there are no other available assays, and for production of an adequate amount of virus required for further genetic analyses. The clinical materials appropriate for VI include samples of biopsy and necropsy tissues, blood, secretions and excretions from diseased animals, although some clinical samples (such as urine, feces, and semen) may be toxic to cell cultures. When collecting tissue samples for VI, it is recommended to collect the cleanest tissues (such as lymph nodes and liver tissues) first and the dirtiest tissues (such as enteric tissues) last in order to prevent bacterial contamination of the clean samples. The amount of material required for VI is usually more than that needed for rapid diagnostic assays; therefore a sufficient amount of clinical material intended for VI should be submitted. The choice of cell culture for VI will largely depend upon the particular suspected virus [108], although some viruses cannot be propagated in cell culture. At least three major types of cell cultures are routinely used for VI: primary cultures, diploid cell lines, and established cell lines [101]. Primary cell cultures are prepared from organs of freshly killed animals. Diploid cell lines usually have a definite life span of 50–60 in vitro passages. A variety of established cell lines from various animal species can propagate various veterinary viruses, and are commercially available from the American Type Culture Collection (ATCC, Manassas, VA). The capability of cell cultures to isolate certain viruses can be greatly enhanced by biochemical or physical treatments [64]. For example, the growth of rotaviruses and reoviruses can be enhanced in the presence of trypsin-containing culture medium and by treatment of clinical samples with trypsin. Isolation of an unrecognized virus in cell cultures often represents a tremendous challenge to veterinary virologists, and primary cell cultures derived from tissues of the same species as the diseased animal are usually the first choice for attempting to isolate an unknown virus. Many viruses produce characteristic cytopathic effects (CPEs) in cell cultures that can be recognized under a microscope. However, for viruses that are noncytopathic, recognition of virus growth in cell cultures is sometime difficult. The observation of a CPE in cell cultures is not sufficient for a definitive viral diagnosis, and additional tests such as hemagglutination inhibition, hemadsorption, virus neutralization, immunodetection techniques, or other antigenic and genetic characterizations must be performed for identification of viruses growing in cell cultures. In addition, chicken embryos and live animals are also used for VI. For example, chicken embryos are often used to isolate avian viruses and influenza virus. Evidence for virus growth in embryonated chicken eggs can be visualized on the chorioallantoic membrane for certain viruses such as poxviruses and avian herpesviruses, although additional confirmatory tests such as hemagglutination, immunofluorescence, and PCR are usually required to verify virus growth. In mice inoculated with virus, clinical signs and characteristic pathological lesions are often indicative of virus growth, but additional laboratory tests such as serology, immunofluorescence, and immunohistochemistry must also be performed for a definitive diagnosis. Natural host animals may also be used to isolate viruses when other methods fail to produce results. Enzyme-linked immunosorbent assay is commonly used for detection of antibodies to infectious agents in veterinary diagnostic laboratories. The basic principle of ELISA is an antigen–antibody reaction measured by colorimetric reaction (optical density or OD) between enzyme-labeled antibody and substrate. The assay is typically inexpensive, sensitive, and rapid, and a diagnosis can usually be made within hours. A large number of samples can be processed in a single run in a fully automated fashion using an automated plate washer and reader. With a known viral antigen coated on the ELISA plates, serum viral antibodies can be measured both qualitatively (positive or negative) and quantitatively (antibody titers). Competitive ELISA (cELISA) or blocking ELISA utilizes an antibody to a known specific agent to compete against specific antibodies in the test serum sample. An antigen is coated onto a solid-phase surface, the test serum and an enzyme-labeled antibody specific for the coated antigen are added together, and they compete for binding to the immobilized antigen. The color developed (by adding a chromogenic enzyme substrate) is inversely proportional to the amount of viral antibody present in the test serum. A serum sample is considered positive in the competitive or blocking ELISA if the OD value is reduced by a defined percentage compared with an unblocked sample. The availability of commercial kits renders quality control relatively simple. ELISA kits for detection of viral antibodies are commercially available for a variety of veterinary viruses (Table 19.1). These commercial ELISA kits are approved by regulatory agencies such as the Department of Agriculture’s Animal and Plant Health Inspection Service (APHIS) in the United States, and the results are usually reproducible from laboratory to laboratory. Table 19.1 Selected commercial diagnostic kits for veterinary viruses of major domestic animal species * Vendor information: Alere Scarborough Inc. – 10 Southgate Road, Scarborough, ME 04074; Centaur – Centaur, Inc., P.O. Box 25667, Overland Park, Kansas 66225-5667; IDEXX – IDEXX Laboratories, Inc., One IDEXX Drive, Westbrook. ME 04092; Ingenesa – Hermanos Garcia, Noblejes, 39, 28037 Madrid, Spain; Life Technologies – Life Technologies, 3175 Staley Road, Grand Island, NY 14072; Meridian – Meridian Bioscience, Inc., 3471 River Hills drive, Cincinnati, OH 45244; Svanova – Boehringer Ingelheim Vetmedica, Svanova, Box 1545, SE-751, 45 Uppsala, Sweden (Svanova Biotech’s US distributor – Diagnostic Chemical Limited, Marketing and Product Manager, Veterinary Diagnostics, 160 Christian Street, Oxford, CT 06478); Synbiotics – Synbiotics, Inc., subsidiary of Pfizer Corp, 812 Springdale Drive, Exton, PA 19341; VDT – Veterinary Diagnostic Technology, Inc., 4890 Van Gordon St. Suite 101, Wheat Ridge, CO 80033; VMRD – VMRD, Inc., 4641 Pullman-Albion Road, PO Box 502, Pullman, WA 99163. † Antigen-capture ELISA: for detection of viral antigen. ‡ ELISA: for detection of viral antibody (serology). Serum virus neutralization (SVN) is used to detect and quantify serum virus-neutralizing antibodies, and is usually performed by mixing a standard amount of virus with serial dilutions of a serum sample. Alternatively, a standard dilution of a serum sample is mixed with serial dilutions of the test virus. The serum–virus mixtures are then inoculated onto monolayers of susceptible cell cultures, and the inoculated cells are monitored for evidence of virus infection. The highest dilution of serum that protects susceptible cells from virus infection is defined as the SVN titer. A susceptible cell culture is required to perform SVN for the test virus. Therefore, for viruses that do not replicate in cell cultures, antiviral-neutralizing antibodies can be detected by inoculating the in vitro serum–virus mixtures into live animals or embryonated eggs for evidence of virus infection. However, the in vivo neutralization approach is expensive and time-consuming, and is of little practical value. Commercial SVN kits are not readily available and veterinary diagnostic laboratories usually have in-house SVN procedures for each test virus. Neutralizing viral antibodies or reference viruses used for SVN controls are commercially available from many veterinary diagnostic laboratories (such as NVSL) or commercial vendors such as VMRD, Inc. (Pullman, WA), as well as the ATCC. Agar gel immunodiffusion (AGID) is a simple and easy assay to detect viral antibodies in serum samples. Also, with a known reference viral antibody, AGID can be used to detect viral antigen as well. The basic principle of AGID is the reaction between diffusing viral antibody and viral antigen on an agar plate. Viral antigen preparations are placed in a central agar well, and positive control and test sera are placed in peripheral wells. Precipitin lines are formed when the diffusing viral antibodies meet and react at optimal conditions with the diffusing viral antigen on the agar plate. Commercial AGID kits are available for antibody detection of many viruses such as equine infectious anemia virus and bluetongue virus (Table 19.1). AGID has also been used in many diagnostic laboratories to detect antibodies to viruses for which commercial AGID kits are not yet available, such as caprine arthritis-encephalitis virus [135], swine encephalomyocarditis virus [75], avian influenza virus [190], and bovine leukemia virus [151]. AGID is usually considered a relatively specific test, but the sensitivity is usually low resulting in false-negative results. A confirmatory test is often needed to validate AGID results. Hemagglutination inhibition (HI) is a simple and inexpensive assay for the detection of serum antiviral antibodies of viruses that hemagglutinate red blood cells (RBC), such as influenza virus and paramyxovirus. The test serum is diluted serially in 96-well plates and incubated with a standard amount of virus. After addition of RBCs from certain animal species, hemagglutination is inhibited in the presence of sufficient amount of serum antibodies that react with the virus. The serum HI titer is expressed as the highest dilution of test serum that inhibits the aggregation of RBCs. The HI reagents are commercially available from diagnostic laboratories (such as NVSL) and commercial vendors such as ATCC and VMRD, Inc. With a known reference viral antibody, HI can also be used to detect viral antigen. Fluorescent antibody assay (FA) is a rapid and specific test for detection of viral antibody. A known viral antigen is immobilized on a slide or plate, and the test sera containing viral antibody is then added to the wells, and the antigen–antibody complex is visualized by adding a fluorochrome (such as fluorescein isothiocyanate (FITC) or Texas-red)-conjugated secondary antibody that reacts with the specific viral antibody. A few commercial FA kits for human and zoonotic viruses (such as Western and Eastern equine encephalitis viruses, parainfluenza virus, respiratory syncytial virus, herpes simplex virus, and adenovirus) are available. Commercial FA kits for veterinary viruses are rare, but FA reagents are commercially available from vendors such as VMRD, Inc., ATCC, and KPL (Gaithersburg, MD), and from veterinary diagnostic laboratories (such as NVSL). Each veterinary diagnostic laboratory usually has its in-house FA procedures for each virus. An immunofluorescence assay can also be used to detect viral antigens if a known specific viral antibody is available (see below). The ability of complement to lyse RBC’s in the presence of anti-RBC antibody (hemolysin) forms the basic principle of the complement fixation (CF) test [21]. The test serum is mixed with defined amounts of a known viral antigen, guinea pig complement, sheep RBCs, and hemolysin (rabbit anti-sheep erythrocyte stromata serum). In the absence of specific viral antibody, sheep RBCs and hemolysin activate complement leading to the lysis of RBCs. If the test serum contains viral antibody, the antigen-specific viral antibody forms immune complexes with the viral antigen. The antibody–antigen complexes bind to and activate complement, thus preventing RBCs from lysis by hemolysin. The amount of CF viral antibody in the test serum is inversely proportional to the amount of RBC lysis. The CF test has been used to detect antibodies of many viruses such as bovine leukemia virus [89], bovine respiratory syncytial virus [46], bovine herpesvirus [48], and malignant catarrhal fever virus [149]. The CF test is usually laborious and time-consuming [21], and therefore is not routinely used in modern veterinary diagnostic laboratories. Nevertheless, CF test kits for certain veterinary viruses are commercially available (Table 19.1). The CF test can also be used to detect viral antigen, such as foot-and-mouth disease virus antigen, when a known antiviral antibody is available [114]. Western immunoblot analysis can detect viral antibodies in serum specific to a viral protein. A purified known viral protein or the entire purified virion is denatured with sodium dodecyl sulfate (SDS), separated by SDS-polyacrylamide gel electrophoresis, transferred and immobilized to a nitrocellulose or nylon membrane, and hybridized with the test serum containing viral antibodies. The presence of an antibody–antigen complex is demonstrated by adding an enzyme-, chemiluminescent-, or radioisotope-labeled secondary antibody. Western immunoblot analysis is often used as a confirmatory test to verify the results of serological assays. This assay has been used for detection of viral antibodies of many viruses such as equine lentivirus [20], bovine immunodeficiency virus [188], African horse sickness virus [17], and bovine leukemia virus [71]. With a known specific viral antibody, Western blot analysis can also be used to detect virus antigens in clinical samples. Supplies and reagents for Western immunoblot analysis are available from commercial companies such as Bio-Rad Laboratories (Hercules, CA). Reference viral antigens and viral antibodies for Western blotting can be purchased from commercial vendors such as VMRD, Inc. and veterinary diagnostic laboratories (such as NVSL). A modification of the conventional ELISA platform is the microsphere-based technology that is based on a liquid suspension array and is designed for multiplex testing [32]. This technology utilizes multiple microspheres (up to 100 sets) and each one is conjugated to a different antigen for uniform detection of multiple antibodies in a small sample volume. Commercial assays using this technology have been introduced for chickens (Biovet Inc., Saint-Hyacinthe, Québec, Canada) and allow simultaneous detection of antibodies to Bursal disease virus, Newcastle disease virus, infectious bronchitis virus, and reovirus in serum samples. Antigen-capture ELISA detects viral antigen (both infectious and inactivated viruses) from a variety of clinical materials such as tissues, secretions, and excretions. Viral antigen-specific antibody is coated to a solid-phase surface (usually a 96-well plate), and the clinical specimen containing viral antigen is then added. After addition of an enzyme-labeled antibody, a chromogenic enzyme substrate is added for color development. The color developed is proportional to the amount of viral antigen present in the specimen. The antigen-capture ELISA is very useful for rapid diagnosis of veterinary viral infections because it is simple and easy to perform. Antigen-capture ELISA kits are commercially available for many veterinary viruses (Table 19. 1). The assay has also been used in veterinary diagnostic laboratories for detection of viral antigens of many other viruses for which commercial antigen-capture ELISA kits are not yet available, such as bluetongue virus [53], bovine coronavirus [146], bovine viral diarrhea virus [144], bovine respiratory syncytial virus [180], and infectious bursal disease virus [52]. Direct immunofluorescence assay utilizes a specific viral antibody labeled with a fluorochrome such as FITC to detect viral antigen. For indirect immunofluorescence assay, the specific viral antibody is not labeled and the antibody–antigen complex is visualized by adding a fluorochrome (such as FITC)-labeled secondary antibody that reacts with the specific viral antibody. Many clinical materials, such as frozen tissue sections, cell smears, or monolayers, are appropriate for this assay, but fresh clinical samples are the best. The limitation of the immunofluorescence assay for viral antigen detection is that cells or tissues containing viral antigens are required, and other samples such as serum or feces cannot be used for this assay. Immunofluorescence assay is routinely used in combination with VI for identification of viruses growing in cell cultures. The reagents needed for immunofluorescence assay are commercially available from many vendors such as VMRD, Inc., and ATCC, or from veterinary diagnostic laboratories (such as NVSL). Each veterinary diagnostic laboratory usually has its in-house immunofluorescence assay procedures for each virus. Immunohistochemistry (IHC) can utilize convenient formalin-fixed tissues for detection of viral antigen, and affords the opportunity to visually localize specific viral antigens within the lesions. The specific viral antigen within tissue sections can be visualized by adding a viral-specific antibody, followed by a biotinylated secondary antibody, an avidin–biotin–peroxidase complex, and a substrate such as DAB (diaminobenzidine tetrahydrochloride) for color development (Figure 19.1). IHC has been widely used among veterinary pathologists to confirm histopathological diagnoses, since the formalin-fixed tissue sections can be used for both histopathological examination and IHC. Most IHC tests employ the avidin–biotin–peroxidase complex method, although other systems such as peroxidase–antiperoxidase complex, and streptavidin–biotinylated–alkaline phosphatase complex are also used. Nonspecific reactions in IHC due to the presence of endogenous peroxidase activity in tissues are a major concern, but can be minimized by blocking the endogenous peroxidase activity with hydrogen peroxide and by including adequate controls. IHC has been successfully used to detect antigens of many veterinary viruses, including but not limited to, feline leukemia virus [66], porcine reproductive and respiratory syndrome virus [50], eastern equine encephalomyelitis virus [120], swine vesicular disease virus [107], swine influenza virus [168], and bovine coronavirus [28]. Many commercial companies, such as DAKO (Carpinteria, CA), Vector Laboratories, Inc. (Burlingame, CA), and KPL, manufacture universal IHC kits that usually contain all necessary IHC reagents except for the specific viral antibody. Specific viral antibody can be purchased separately from veterinary diagnostic laboratories (such as NVSL) or commercial vendors such as VMRD, Inc. A searchable database of available IHC assays, currently being offered at different United States veterinary diagnostic laboratories, is available at http://ihc.sdstate.org/. The intended users of this database are veterinary diagnosticians (i.e., pathologists, microbiologists, virologists, and others). Figure 19.1 Immunohistochemical detection (IHC) of porcine reproductive and respiratory syndrome virus (PRRSV) antigen in the alveolar macrophages of a lung from a pig infected with PRRSV. Alveolar macrophages show dark brown staining of PRRSV antigen. Avidin–biotin peroxidase complex staining, hematoxylin counterstain. (Courtesy of Dr. Patrick G. Halbur, Iowa State University.) The latex agglutination test (LAT) is a simple test to detect viral antigen present in clinical specimens by agglutination with small latex beads coated with known specific viral antibody. The antibody-coated latex beads are stable, and the results can be read by eye or can be measured photometrically within 30 min. This assay is traditionally used to detect the presence of viral antigen in feces of many veterinary viruses such as porcine rotavirus [145], bovinerotavirus [51,113], and canine parvovirus [15]. With a known viral antigen, LAT can also be used to detect viral antibody. LAT kits are commercially available for a few veterinary viruses (Table 19.1). The major concern for the use of LAT is its low sensitivity compared to other immunoassays. Edwin Southern in 1975 [153] pioneered the Southern blot to detect specific sequences among hundreds and thousands of restriction fragments of genomic DNA. The technique utilizes restriction enzymes that recognize specific cleavage sites of DNA and cleave the DNA into smaller fragments. After separation of the cleaved DNA fragments by gel electrophoresis, the DNA fragments are transferred to a membrane such as nitrocellulose, denatured, and hybridized with a labeled specific probe. Southern blot analysis is useful for detecting nucleic acids of DNA viruses such as porcine parvovirus [79], bovine leukemia virus [43], ovine papillomavirus [95], and bovine herpesvirus [91]. Traditionally, 32P was used to label probes by methods such as nick translation and end-labeling. However, due to safety concerns, many diagnostic laboratories now use nonradioisotope labeling methods such as biotin [80], digoxygenin [73], or chemiluminescence. The digoxygenin system is particularly attractive because nonspecific background can be minimized. The modified digoxygenin only occurs in nature in the Digitalis plant. Thus, nonspecific reactions are greatly reduced. Several companies such as DAKO, Roche Diagnostics (Indianapolis, IN), Thermo Scientific (Rockford, IL), Applied Biosystems (Bedford, MA), and GE Healthcare Life Sciences (Amersham Pharmacia Biotech, Inc.; Pittsburgh, PA) manufacture commercial reagents and/or kits for the biotin, digoxigenin, and radioisotope labeling systems. However, the specific viral genomic fragments to be labeled as probes have to be generated in the research or diagnostic laboratories for each virus of interest. The popularity of Southern blot, dot blot, or in situ hybridization is diminishing due to the widespread use of PCR diagnostic technologies. Dot blot hybridization can detect viral DNA or RNA immobilized onto a filter such as a nitrocellulose membrane. Total nucleic acids extracted from clinical samples are immobilized, denatured, and hybridized with a radioisotope-, chemiluminescent-, or enzyme-labeled single-strand viral nucleic acid probe. After washing away the unbound probe, the membrane is exposed to a film for autoradiography or, in the case of an enzyme-labeled probe, the signal of bound probes in the membrane is measured by color development with a substrate. Dot blot hybridization has been used to detect nucleic acids of many viruses such as porcine and bovine enteric coronavirus [150], infectious bursal disease virus [56], bovine rotavirus [118], swine encephalomyocarditis virus [104], feline infectious peritonitis virus [97], and avian reoviruses [93]. Like Southern blotting, virus-specific probes have to be generated, but reagents for probe labeling and hybridization are commercially available from companies such as Amersham (now GE Healthcare Life Sciences). In situ hybridization (ISH) can detect viral nucleic acids in affected tissues at the genomic level. The principle of ISH is base-pairing (hybridization) between a labeled viral nucleic acid probe and the complementary base sequences of the target viral genomic DNA or RNA present in tissues or cells. Non-radioactive methods (such as biotin, digoxigenin, or chemiluminescence) have now gradually replaced the radioactive methods in labeling nucleic probes in ISH. For example, biotin-labeled viral DNA or RNA probes are now commonly used to detect viral nucleic acids in clinical samples by adding enzyme-labeled avidin and a substrate (Figure 19.2). Under a light microscope, a colorimetric reaction within a particular virus-infected cell can be visualized in the context of other surrounding microscopic structures. Commercial ISH kits are available from many vendors such as Roche Diagnostics and DAKO, and these kits usually contain all the necessary reagents for probe labeling and ISH procedures. However, a specific viral genomic fragment or a synthetic oligonucleotide for each virus of interest has to be generated in a research or diagnostic laboratory for labeling as probes. ISH has now been widely used to detect nucleic acids of many veterinary viruses such as porcine reproductive and respiratory syndrome virus [54,83], porcine circovirus [102], porcine epidemic diarrhea virus [76], avian reoviruses [92], chicken anemia virus [141], avian adenovirus [85], bovine coronavirus [189], and rabbit hemorrhagic disease virus [44]. Figure 19.2 In situ hybridization detection (ISH) of porcine reproductive and respiratory syndrome virus (PRRSV) nucleic acid in the alveolar macrophages of a lung from a pig infected with PRRSV. PRRSV nucleic acid is detected in the cytoplasm of macrophages. ISH with a digoxigenin-labeled PRRSV riboprobe detected by anti-digoxigenin–alkaline phosphatase complex, nuclear fast red counterstain (Courtesy of Dr. Patrick G. Halbur, Iowa State University). The unmatched sensitivity and quick turnaround time of PCR is very useful for rapid detection of low levels of viral nucleic acids from virtually any clinical sample. PCR-based viral diagnosis is now being widely used in veterinary diagnostic laboratories, and many commercial PCR diagnostic kits are now available for detection of viral nucleic acids. The basic principle of PCR is the enzymatic synthesis of a specific DNA sequence by using two short synthetic oligonucleotide primers, usually 18–24 base pairs (bp). The primers bind to the complementary sequences of the target DNA, and the viral genomic region flanking the two primers is amplified repeatedly by a thermostable Taq polymerase in an automated cycling fashion in a Thermocycler. A typical PCR cycle consists of denaturation of target DNA usually at 94°C for 30–60 s, annealing of primers to the target DNA at an appropriate temperature (usually between 42 and 60°C) for 30–60 s, and extension of the products at 72°C for a certain length of time depending upon the size of the amplified fragments. After 25–35 cycles, the target DNA is amplified to a level that can be detected as a visible band by gel electrophoresis. The identity of an expected size of PCR product usually can be further confirmed by restriction enzyme digestion or nucleotide sequencing. The design of PCR primers is a key to the successful amplification of the target viral nucleic acids. The target virus nucleotide sequence must be known in order to design primers for PCR detection, and the primers need to be specific to the virus in question, but common to all strains of that virus. Since the introduction of PCR in 1983, numerous modifications of the original method have been applied for the diagnosis of infectious diseases such as reverse transcriptase PCR (RT-PCR), nested PCR, multiplex PCR, quantitative PCR, in situ PCR, and real-time quantitative PCR. Reagents for these PCR-related assays are readily available from many commercial vendors. A searchable database for veterinary diagnosticians that includes currently available commercial and in-house PCR tests offered at different US veterinary diagnostic laboratories can be found at http://pcr.sdstate.org/. RT-PCR detects viral RNA from clinical samples. The first step of RT-PCR is to extract viral genomic RNA or total RNA from clinical samples. RNA extraction kits and reagents from a variety of clinical specimens are available from many commercial companies, such as Qiagen RNA extraction kits (Qiagen Inc., Valencia, CA) and TriZol Reagents (Life Technologies, Grand Island, NY). The RNA template is reverse-transcribed into cDNA with a reverse transcriptase, which is then amplified by PCR with specific viral primers. A limitation of PCR has been that the enzymatic activity of the traditional retrovirus reverse transcriptase does not function in temperatures over 42°C. Therefore, the presence of strong secondary structures in the single-strand RNA template during cDNA synthesis at 42°C may limit specific cDNA synthesis. The discovery of a DNA polymerase with reverse transcriptase activity from a thermophilic bacterium has overcome this problem, and cDNA synthesis can now be performed at up to 65–70°C. Commercial RT-PCR kits in which cDNA synthesis is carried out at up to 70°C are now available, such as the rTth RT-PCR kit (Perkin Elmer, Waltham, MA). RT-PCR has been widely used to detect nucleic acids of many RNA viruses such as porcine epidemic diarrhea virus [65], infectious bronchitis virus [172], avian pneumovirus [2], porcine reproductive and respiratory syndrome virus [170], and bovine viral diarrhea virus [132]. Nested PCR and nested RT-PCR are extremely sensitive assays that are used for detection of nucleic acids of both DNA viruses, such as canine herpesvirus [147] and bovine herpesvirus [136], and RNA viruses, such as feline infectious peritonitis virus [40] and canine coronavirus [125]. The assays utilize two sets of specific PCR primers. In the first round of PCR, the external sets of primers amplify a product that serves as the template for the second round of PCR amplification. The second round of PCR (nested PCR) utilizes an internal set of PCR primers within the region of the first round PCR product. Therefore, the second round of PCR amplification often serves as a confirmation for the specificity of the PCR products amplified in the first round. Because of the extreme sensitivity, care must be taken to avoid carry-over or cross-contamination, which could lead to false-positive results. Multiplex PCR detects two or more target viral sequences in a single PCR reaction. Therefore, multiplex-PCR is more cost effective than other PCR methods. Usually two or more sets of primers specific for different target viral sequences are included in the same amplification reaction, and each specific set of primers produces different sized PCR products that can be distinguished by gel electrophoresis. Multiplex PCR has been used for the diagnosis of multiple virus infections from the same specimen, and for simultaneous detection and differentiation of different genotypes or serotypes of related viruses [45,82,133,159,162,173,186]. In situ PCR and in situ RT-PCR can directly detect viral nucleic acids in paraffin-embedded, formalin-fixed tissue sections by directly incorporating a labeled dNTP, such as digoxigenin-11-deoxyuraciltriphosphate (dUTP), into the amplicon during the in situ PCR reaction to visualize viral DNA or RNA in tissues by a colorimetric reaction. In situ PCR and in situ RT-PCR have been successfully used to detect nucleic acids of many viruses such as equine herpesvirus [16], avian reoviruses [92], infectious bursal disease virus [93], and porcine circovirus type 2 (PCV2) [90]. Negative-strand RT-PCR detects nucleic acids of replicating RNA viruses from clinical samples. Positive-strand RNA viruses produce an intermediate negative-strand RNA during virus replication. A specific primer complementary to the negative-strand RNA of the target virus is used for reverse transcription and cDNA synthesis. The resulting cDNA is then amplified by PCR. This method allows for distinction between virus replicating in the tissues and circulating virus. A positive result of a negative-strand RT-PCR indicates the presence of nucleic acid from a virus replicating in the particular tissue, and not due to contamination of tissues by circulating virus through the bloodstream. Negative-strand RT-PCR is primarily used for viral pathogenesis studies, for identification of virus replication sites [14,183], and for confirmation of virus replication in cell cultures in combination with VI.
Commercial Methods in Clinical Veterinary Microbiology
19.1 Collection and transportation of clinical samples
19.2 Selection of diagnostic laboratories and tests
19.3 Pathology and histopathology
19.4 Quality control of veterinary diagnostic assays: sensitivity and specitivity
19.5 Veterinary virology
19.5.1 Assays for detection of virus
19.5.1.1 Electron microscopy
19.5.1.2 Virus isolation and identification
19.5.2 Assays for detection of antiviral antibodies
19.5.2.1 Enzyme-linked immunosorbent assay
Animal
Virus
Assay
Vendor*
Avian
Newcastle disease virus
ELISA†
PCR
IDEXX, Synbiotics
Life Technologies
Infectious bursal disease virus
ELISA
IDEXX, Synbiotics
Infectious bronchitis virus
ELISA
IDEXX, Synbiotics
Avian Reovirus
ELISA
IDEXX, Synbiotics
Avian encephalomyelitis virus
ELISA
Synbiotics, IDEXX
Chicken anemia virus
ELISA
Synbiotics, IDEXX
Reticuloendotheliosis virus
ELISA
IDEXX
Avian leukosis virus
ELISA, Antigen-capture ELISA‡
Synbiotics, IDEXX
Avian pneumovirus
ELISA
Svanova, IDEXX, Synbiotics
Avian influenza virus
ELISA
Antigen-capture ELISA
Synbiotics, IDEXX
Alere Scarborough, Inc.
PCR
Life Technologies
Bovine
Bovine viral diarrhea virus
Antigen-capture ELISA
IDEXX
ELISA
IDEXX, Svanova, VMRD, Synbiotics
PCR
Life Technologies
Bluetongue virus
AGID
VMRD, VDT
cELISA
ELISA
VMRD
Synbiotics
CF
PCR
VDT
Life Technologies
Bovine leukemia virus
ELISA
Svanova, IDEXX, Synbiotics
AGID
Synbiotics
Infectious bovine rhinotracheitis virus (bovine herpesvirus-1)
ELISA
Svanova, IDEXX, Synbiotics
PCR
Life Technologies
Bovine coronavirus
ELISA
Svanova
Bovine respiratory syncytial virus
ELISA
Svanova
Parainfluenza virus (PIV-3)
ELISA
Svanova
Epizootic hemorrhagic disease virus
AGID
VDT
Canine
Canine parvovirus
Antigen-capture ELISA
ELISA
Synbiotics
Synbiotics
Canine distemper virus
Rabies
ELISA
ELISA
Synbiotics
Synbiotics
Caprine Ovine
Border disease virus
ELISA
Svanova
Caprine arthritis encephalitis virus
AGID
Antigen-capture ELISA
VDT
VMRD
Ovine progressive pneumonia virus
AGID
VDT
Equine
Equine influenza virus type A
Antigen-capture ELISA
Centaur
Equine infectious anemia virus
AGID
VMRD, IDEXX, Synbiotics
ELISA
Centaur, Synbiotics, VMRD
Equine herpesvirus
ELISA (type 1 and 4 differential test)
Svanova
West Nile Virus
ELISA
IDEXX
Feline
Feline leukemia virus
Antigen-capture ELISA
Synbiotics
Feline immunodeficiency virus
ELISA
Avecon, IDEXX
Feline infectious peritonitis virus
ELISA
Avecon, Synbiotics
Rabies
ELISA
Synbiotics
Porcine
Porcine reproductive and respiratory syndrome virus
ELISA
PCR
IDEXX
Life Technologies
Pseudorabies virus
ELISA
Svanova, IDEXX, Synbiotics
Porcine circovirus type 2
Classical swine fever virus
ELISA
Antigen-capture ELISA
Synbiotics, Ingenasa
Synbiotics
ELISA, antigen-capture ELISA
IDEXX, Synbiotics
Swine influenza virus H1N1
ELISA
IDEXX
Swine Influenza virus H3N2
ELISA
IDEXX
Influenza virus type A
Antigen-capture ELISA
PCR (detection or subtyping)
RIM test
Centaur
Life Technologies
Synbiotics
Transmissible gastroenteritis virus/porcine respiratory coronavirus
ELISA (differential test)
Svanova
19.5.2.2 Serum virus neutralization
19.5.2.3 Agar gel immunodiffusion assay
19.5.2.4 Hemagglutination inhibition assay
19.5.2.5 Fluorescent antibody assay
19.5.2.6 Complement fixation test
19.5.2.7 Western immunoblot analysis
19.5.2.8 Fluorescent microbead-based immunoassay
19.5.3 Assays for detection of viral antigen
19.5.3.1 Antigen-capture ELISA
19.5.3.2 Immunofluorescence assay for antigen detection
19.5.3.3 Immunohistochemistry
19.5.3.4 Latex agglutination test
19.5.4 Assays for detection of viral nucleic acids
19.5.4.1 Southern blot hybridization
19.5.4.2 Dot blot hybridization
19.5.4.3 In situ hybridization
19.5.4.4 Polymerase chain reaction
19.5.4.4.1 RT-PCR
19.5.4.4.2 Nested PCR and nested RT-PCR
19.5.4.4.3 Multiplex PCR
19.5.4.4.4 In situ PCR and in situ RT-PCR
19.5.4.4.5 Negative-strand RT-PCR
19.5.4.4.6 Real-time PCR
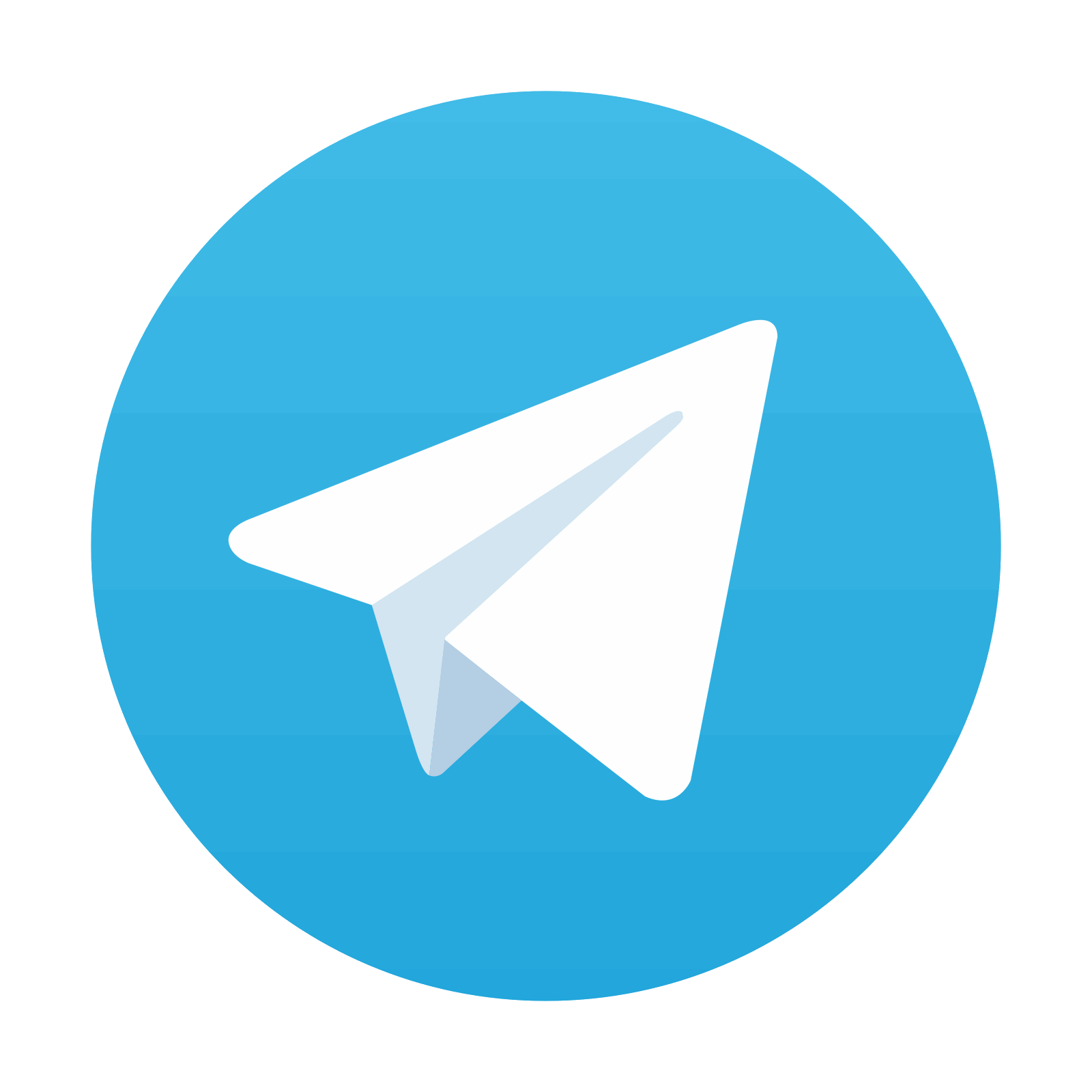
Stay updated, free articles. Join our Telegram channel

Full access? Get Clinical Tree
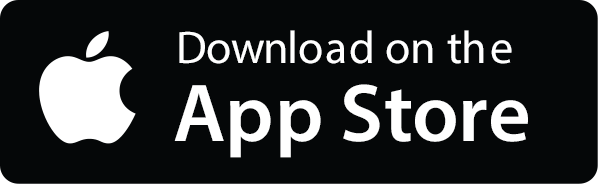
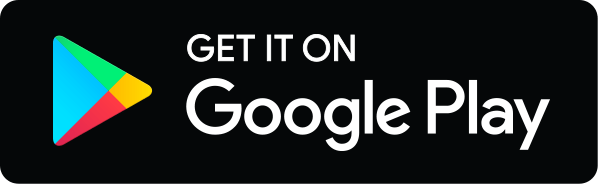