CHAPTER 18 Ruth Ann Luna Texas Children’s Hospital and Baylor College of Medicine, Houston, TX, USA Molecular typing allows for the identification of different strains of a particular bacterial species. While all of the strains tested are the same species (e.g. Pseudomonas aeruginosa), subtle differences in the bacterial genomes allow differentiation between strains that are unrelated and those that may have originated from a common source. Clonally related strains will create a cluster, a group of highly similar molecular fingerprinting patterns, when analyzed by molecular methods. A clonal relationship would suggest that they originated from the same bacterial cell and could indicate a lateral or point source of transmission. Molecular epidemiological surveillance has become a routine tool for many infection control practitioners. The ability to track outbreaks in real time as well as ruling out potential new opportunities for nosocomial infections is greatly enabled by the in-house utilization of molecular typing techniques. Routine surveillance has also proven useful in determining whether changes in infection control guidelines are effective in preventing transmission of known strains [29]. With accurate clustering of a well-characterized group of isolates, further analysis can enable determination of risk factors for acquisition of a particular strain and outcome data, such as the potential association of greater morbidity and mortality with documented infection of a known strain. The discriminatory power of a molecular typing technique describes the technique’s ability to differentiate unrelated isolates [50]. Techniques with reasonable discriminatory power for molecular typing include restriction fragment length polymorphism (RFLP), ribotyping, random amplified polymorphic DNA (RAPD), pulsed-field gel electrophoresis (PFGE), arbitrarily-primed PCR (AP-PCR), repetitive element PCR (rep-PCR), multilocus sequence typing (MLST), and most recently, next-generation sequencing. Additional strategies for discriminating strains have been employed through the years, such as spa sequencing for methicillin-resistant Staphylococcus aureus typing [22,34], specific polymorphism-targeted approaches for Mycobacterium tuberculosis differentiation [33,63], and multilocus variable number tandem repeat analysis [30,37], but these techniques will not be discussed in detail in this chapter. Various factors of each technique determine their acceptability for clinical use. Lack of reproducibility has hampered clinical application of AP-PCR and RAPD [54,62]. RFLP proved too labor-intensive for daily clinical use, and ribotyping provided only moderate discriminatory power compared to the other techniques [54]. PFGE was considered the gold standard for many years, but there are several factors that make this technology less than ideal for the clinical laboratory. PFGE requires a 2–4 days turnaround time, is costly to perform, is labor-intensive, and requires specialized expensive equipment [54,62]. In contrast, the major complaint of rep-PCR was that the technology lacked standardization [62], while others suggested the improved reproducibility of the technology would lend itself to standardization as a library typing system [54]. The issues with standardization would later be remedied by the introduction of the DiversiLab system (bioMérieux, Durham, NC) [23]. The DiversiLab system offered several improvements to the rep-PCR process, including optimized PCR chemistry provided in a reagent kit format, microfluidics-based DNA amplicon detection, and internet-based computerized analysis and data storage. While the DiversiLab system was one of the first standardized molecular typing platforms, further advances in the areas of next-generation sequencing may ultimately prove to have greater discriminatory power [16,32]. When reviewing studies in molecular typing, the definitions of clones and strains become important. Clones are defined as genetically related isolates that are indistinguishable or so similar to each other that they are presumed to descend from a common parent, and a strain is an isolate or group of isolates that can be distinguished from other isolates of the same genus and species [57]. In regards to conventional typing strategies (nonsequencing-based), indistinguishable or closely related isolates should have no more than a two to three band difference (absence or presence of a band), isolates that are possibly related would have a four to six band difference, and those isolates that are clearly different, considered distinguishable, would have a seven or greater band difference [57]. Several current typing methods utilize bacterial DNA purified from a single microbial isolate. This generally requires routine culture in the clinical microbiology laboratory to isolate a single bacterial species (a pure culture) and provide enough cultured material for adequate extraction of bacterial DNA associated with that single isolate. Most laboratories then extract DNA using commercially available kits and platforms. The resulting nucleic acid is then assessed for both quantity and quality prior to use in downstream molecular typing applications. Currently, there is no FDA-approved assay or platform for molecular bacterial strain typing. This chapter will include selected methods, products, and platforms used for strain typing and data analysis. Other products and instruments may be available worldwide in this area. The reader is encouraged to review current scientific literature, web sites, and regional vendors and companies for additional information. Pulsed-field gel electrophoresis (PFGE) is performed by digestion of chromosomal DNA with rare-cutting restriction enzymes and analysis of the resulting series of fragments by agarose gel electrophoresis [50]. The “pulsed-field” is obtained by constantly changing the direction of the electrical field during electrophoresis: the final molecular profiles can then be analyzed by various software programs including BioNumerics by Applied Maths (Austin, TX). A representative workflow of PFGE developed by the Centers for Disease Control (CDC) is shown in Figure 18.1. Figure 18.1 Simplified Process of Pulsed-Field Gel Electrophoresis. This schematic developed by the Centers for Disease Control explains the PFGE process from bacterial culture to data analysis. (Source: http://www.cdc.gov/pulsenet/pathogens/protocol-images.html). Pure culture of the desired isolate is mixed with a cell-suspension buffer. The cell suspension is then added to melted agarose and poured into a mold to form a gel plug. Cells are lysed within the agarose using a cell lysis buffer (usually containing 1% Sarcosyl), and the agarose plugs are washed with water and TE (Tris-EDTA) buffer. Restriction enzyme digestion is performed with a complement of enzymes specifically chosen for the bacteria to be typed. Digestion reactions are loaded into an agarose gel, and voltage conditions specific to the bacteria are applied. When electrophoresis is complete, a stain, such as ethidium bromide, is applied to visualize the DNA bands under UV light. The resulting profiles can then be captured by digital image and analyzed using the appropriate software program. Once PFGE profiles are obtained, comparisons can also be made with national data repositories such as PulseNet, a resource maintained by the CDC (http://www.cdc.gov/pulsenet). PulseNet was created to alert healthcare professionals about potential pathogenic outbreaks of food-borne illnesses and maintains national data for a limited number of key bacterial species [55]. PFGE has been used in clinical molecular typing studies in many institutions and has been instrumental in the discovery of outbreak situations. PFGE typing in a neonatal intensive care unit determined a clonal relationship between the strains of Serratia marcescens isolated from the patients and those found in the hand-washing disinfectant used by the nursing staff [59]. Isolating each of the patients infected with S. marcescens and more diligent hand washing and glove use by the staff contained the outbreak. PFGE was also shown to identify two endemic strains of methicillin-resistant Staphylococcus aureus [39] and a cluster of Enterobacter cloacae associated with a high mortality rate [27]. In another example of molecular typing of antibiotic resistant organisms, PFGE identified a strain of vancomycin-resistant Enterococcus faecium that was present in 85% of the samples typed [14]. The implementation of weekly surveillance cultures and the increased use of barrier precautions were effective in controlling the spread of the strain in the hematology unit of the hospital. Repetitive element PCR (rep-PCR) capitalizes on the widespread distribution of repetitive DNA elements throughout the genomes of many microorganisms [58]. By positioning amplification primers in these repetitive regions, amplified fragments of various lengths and quantities are produced leading to a unique molecular fingerprint for each strain of a particular species. Rep-PCR has been significantly improved by the introduction of bioMérieux’s DiversiLab system (Durham, NC). The complete DiversiLab system offers quality-controlled reagents in a kit format, microfluidics-based amplified fragment detection, and internet-based computer assisted analysis, reporting, and data storage [23]. The level of discrimination and reproducibility of the technology paired with the electronic storage of data created an ideal environment for the archiving of rep-PCR data for comparative longitudinal and epidemiological studies. Rep-PCR oligonucleotide primers bind to conserved, interspersed repetitive sequences throughout the bacterial genome, and these primers direct amplification at multiple primer binding sites to produce multiple DNA fragments of various lengths, yielding strain-specific DNA profiles. Amplification kits are available for various bacterial genera and bacterial groups, with each kit containing an optimized set of primers to promote discriminatory power. The amplified products are loaded into the DNA LabChip, combined with a proprietary gel-dye matrix, and size-fractionated by microfluidics and electrophoresis in the Agilent 2100 Bioanalyzer (Agilent Technologies, Santa Clara, CA). The Bioanalyzer uses a series of wells and microchannels to electrophoretically separate DNA fragments, and each fragment is detected by laser-induced fluorescence (excitation wavelength of 635 nm, emission wavelength of 685 nm) of a proprietary dye that intercalates into DNA. The various sizes and relative intensities of the amplified fragments yield clone- or strain-specific chromosomal DNA profiles. Automated DNA fingerprinting results are then transferred to the DiversiLab analysis software. The software uses the Pearson correlation coefficient and the unweighted pair group method with arithmetic mean (UPGMA) to compare the presence or absence of amplified fragments as well as the intensity of each fragment. This analysis leads to the generation of a dendrogram, similarity matrix, and scatterplot that conveys the relative similarities of isolates based on the DNA profiles generated by rep-PCR (Figure 18.2). The dendrogram is a hierarchical tree representation that uses group averages to display relative similarities or clusters of the isolates. The similarity matrix builds upon the information provided in the dendrogram by providing a percent similarity for each node of the tree. The scatterplot is a nonhierarchical data display that uses a two-dimensional spatial representation to display relative similarities of isolates by virtue of relative distances on a grid. Figure 18.2 DiversiLab Report Components. This DiversiLab report is an example of an automated analysis and reporting system for molecular strain typing that provides several options for visualizing relatedness of clones including: (a) upper left, a dendrogram, (b) upper right, a scatterplot, and (c) lower, a similarity matrix. (Courtesy of bioMérieux.) Rep-PCR is a proven technology for typing of various bacterial species. Published studies have used rep-PCR to type Acinetobacter baumanii [31] and Vibrio vulnificus [7], as well as several other bacteria isolated from the International Space Station [6]. One study that separated Listeria monocytogenes isolates into four separate clusters suggested that molecular fingerprints be considered different if there was a discrepancy (presence or absence) of at least two bands [25]. A larger study that evaluated the relatedness of several organisms (Acinetobacter spp., Enterobacter spp., and coagulase-negative Staphylococcus) between an individual’s hands and the immediate environment stated specific criteria for the identification of clusters [38]. Interestingly, greater than 25% of the bacterial isolates found on the hands and in the immediate environment of a single household were found to be the same strain. Different isolates were defined as those with less than 95% similarity and a two to three band difference. Similar isolates were described as those with less than 97% similarity but only one or two bands difference; and indistinguishable isolates were defined as those with greater than 95% similarity and no band differences, including intensities. Overall profile intensity differences were acceptable because that indicated a universal change throughout the whole rep-PCR profile most likely due to efficiency of the PCR rather than actual differences between the bacterial isolates. In addition to bacterial typing, rep-PCR has been recommended as an effective tool for the typing of fungi [24] and dermatophytes [41]. Most importantly, rep-PCR has been proven for use in infection-control investigations. Rep-PCR of vancomycin-resistant Enterococcus was instrumental in the control of an outbreak in the neonatal intensive care unit (NICU) in one hospital [51]. In another institution, rep-PCR epidemiologically linked a NICU outbreak of methicillin-resistant Staphylococcus aureus (MRSA) to a healthcare worker afflicted with chronic otitis externa and nasal colonization with a specific MRSA clone [4]. Several studies have compared the discriminatory power of fragment-based molecular profiles for molecular typing. One study compared PFGE, rep-PCR, and AP-PCR for typing of Bartonella henselae and found that PFGE and rep-PCR had the highest discriminatory potential [46]. Another study compared rep-PCR and RFLP for the typing of Pseudomonas isolates from soil and concluded that rep-PCR and RFLP were comparable methods [9]. A comparison of rep-PCR, PFGE, and ribotyping in Clostridium difficile found a high correspondence between rep-PCR and PFGE results but lesser discrimination with the ribotyping results [52]. Multiple investigators chose to perform a direct comparison between PFGE and rep-PCR. Candida albicans was a specific pathogen of interest, and rep-PCR was found to be comparable to PFGE [8]. One study showed that the DiversiLab rep-PCR system was more rapid and conducive to ongoing epidemiologic studies in Candida species [61]. The DiversiLab system was also evaluated for typing of S. aureus
Molecular Typing Instruments and Methods
18.1 Introduction
18.2 Background
18.3 Current molecular typing methodologies
18.3.1 Pulsed-field gel electrophoresis
18.3.2 Repetitive element PCR
18.4 Comparison of typing techniques
Stay updated, free articles. Join our Telegram channel

Full access? Get Clinical Tree
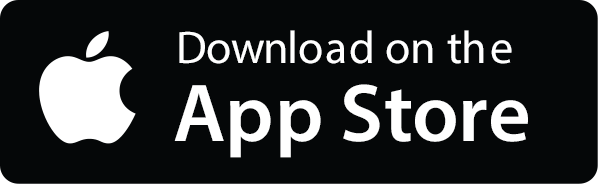
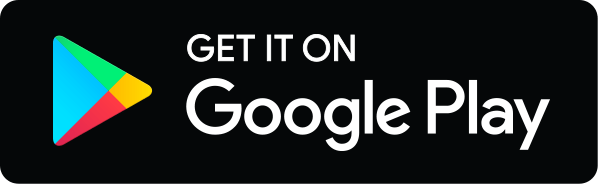