CHAPTER 16 Ian W. Seetho; John P.H. Wilding CHAPTER OUTLINE Pharmacological management of cardiovascular risk GLUCOSE-LOWERING THERAPY IN DIABETES Insulin use in type 1 diabetes Glycaemic management in type 2 diabetes Sulfonylureas (and related insulin secretagogues) Peroxisome proliferator activator γ analogues Glucagon-like peptide 1 analogues Dipeptidyl peptidase IV inhibitors Insulin use in type 2 diabetes Immunotherapy for type 1 diabetes OBSTACLES TO ACHIEVING GLYCAEMIC CONTROL Intercurrent illness, ‘sick day rules’ and stress CHRONIC COMPLICATIONS OF DIABETES Hyperosmolar hyperglycaemic state MANAGEMENT OF DIABETES IN THE HOSPITAL SETTING The rise in prevalence of diabetes presents some of the greatest medical and economic challenges of the 21st century. Despite advances in our understanding and management of diabetes mellitus, it continues to exact a huge toll both in terms of morbidity and mortality. In this chapter, we consider dietary, lifestyle and macrovascular risk optimization measures for people with diabetes. Glucose-lowering treatment is discussed, followed by obstacles patients face in order to achieve their glycaemic goals, particularly hypoglycaemia. Later sections discuss the management of chronic and acute complications of diabetes, and its management in particular circumstances, for example in critical illness and during pregnancy. The management of patients with diabetes should involve a multidisciplinary team composed of diabetologists, diabetes specialist nurses, dietitians, podiatrists, the patient’s general practitioner and other specialists if specific complications are present. In addition to therapies that specifically target glycaemic control, nutritional management, lifestyle modifications such as adequate physical exercise and smoking cessation, and structured patient educational programmes are key components in the management of patients with diabetes. Patients with diabetes should follow a healthy diet and make appropriate nutritional choices to reduce cardiovascular risk and improve glycaemic control. A dietitian with specialist knowledge in diabetes should take the lead role in providing nutritional care. Dietary advice should be tailored specifically for each patient, considering their personal and cultural preferences, beliefs, lifestyle and the changes that the patient is willing and able to make. The principles of diet include eating regular meals with a reduction in simple carbohydrate intake with an increase in complex carbohydrates, a high fibre content and an energy intake to achieve or maintain an ideal body weight, or in those who are overweight or obese, to achieve and sustain modest weight loss. The key aim is to balance energy input and expenditure and ensure a good quality diet. Contrary to the general perception that a diet in diabetes needs to be unique or special, there is no special ‘diet for diabetes’. People with diabetes, except perhaps in specific circumstances such as renal failure, should eat the same healthy diet as recommended to those who do not have diabetes. Thus, no more than 30% of total energy intake should be in the form of fat, and the ratio of unsaturated to saturated fats should be increased with saturated fat intake ideally making up < 7% of total calories and trans fatty acid intake minimized. Replacement of saturated fats with mono- or polyunsaturated fats aids lowering of cholesterol. Carbohydrate (preferably in the form of slowly absorbed complex carbohydrate with a low ‘glycaemic index’ rather than simple sugars) should provide approximately 55% of total energy intake with protein providing the remaining 15%. Foods that improve glycaemic control and might reduce cardiovascular risk include whole grains and green vegetables. Conversely fried foods, high glycaemic index diets and red and processed meats may increase cardiovascular risk. Carbohydrate intake can significantly affect postprandial blood glucose concentrations. In type 1 diabetes, matching insulin doses to the amount of carbohydrate consumed (carbohydrate counting) is effective in improving glycaemic control. Patients who are on fixed insulin doses or biphasic insulin regimens should be advised to aim for consistency in carbohydrate and glycaemic index dietary intake. In patients treated with diet only or diet and oral hypoglycaemics, total energy intake in terms of carbohydrate consumption should be monitored as this is important in achieving glycaemic control. Strategies employed may include carbohydrate counting, exchanges or food portions based on past experience. However, as each individual is different, there is no fixed recommended ideal macronutrient composition of carbohydrate, fat and protein in the diet. Nevertheless, some studies have shown improved body weight and glycaemic control with low carbohydrate diets through a reduction in energy intake over the short term. Sodium intake should not generally exceed 6 g/day and plentiful fruit and vegetables (‘five portions a day’ being the current mantra) should be consumed, mindful of the fact that some fruits (e.g. grapes) may contain large amounts of sugar and should not be taken in excess. A total daily dietary fibre intake of 40 g is ideal but rarely achieved in practice. Except in specific and unusual circumstances, it is a general principle that the pharmacological treatment of diabetes should be tailored to the dietary intake and lifestyle of the individual and not vice versa. Fructose reduces postprandial glycaemia when it is used as a replacement for sucrose or starch, but it may have adverse metabolic effects including causing dyslipidaemia and insulin resistance. Non-nutritive sweeteners are safe when consumed within recommended daily limits. It is currently recommended that patients with new onset type 2 diabetes should be managed with dietary and lifestyle modification with consideration for early introduction of metformin (discussed later). The degree of adherence to a particular diet will affect the outcome and a diet that is both palatable and acceptable is more likely to be followed. Patients with diabetes should be encouraged to participate in regular exercise of the kind that would benefit the rest of the population. Regular low-intensity exercise, such as brisk walking (so as to be slightly breathless while talking at the same time), swimming or cycling for 30 min three to five times a week should be a realistic goal for many individuals. Exercise improves glucose disposal (by increasing the expression of type 4 glucose transporters, GLUT4) in skeletal muscle, among other mechanisms), prevents progression from impaired glucose tolerance to type 2 diabetes (by ~ 50%), increases basal metabolic rate and has convincingly been demonstrated to reduce the risk of cardiovascular events. Some patients with cardiovascular disease require detailed assessment (e.g. electrocardiogram, review of medications) before taking part in exercise programmes, but the few absolute contraindications to exercise in patients with diabetes (e.g. tight aortic valvular stenosis) apply equally to individuals without diabetes. In insulin-treated patients, exercise may be associated with either an increased frequency of hypoglycaemia or hyperglycaemia as blood glucose concentrations are influenced by the timing, type and quantity of insulin, carbohydrate intake and intensity and duration of physical activity. Careful monitoring of blood glucose concentrations is necessary to guide the adjustment of insulin therapy and carbohydrate intake, and the strategy adopted may depend on whether the exercise is planned or unplanned. Aerobic exercise improves glycaemic control and lowers low density lipoprotein (LDL) cholesterol in patients with type 2 diabetes, but has little effect on other lipid parameters. Resistance training has effects on both glycaemia and cardiovascular risk factors such as systolic blood pressure, fat mass and insulin resistance. Studies have shown that it is safe for individuals with type 2 diabetes who are treated by diet alone or in conjunction with oral hypoglycaemic agents to exercise in both the fasting and post-meal states with the most beneficial effects on blood glucose concentrations observed postprandially. As with dietary modification, exercise programmes must be individually designed in order to encourage compliance. Smoking is hazardous to all, but is particularly so in people with diabetes. The macrovascular risks of smoking are compounded with those of diabetes itself. Smoking is associated with increased mortality and predisposes to microvascular complications of diabetes such as retinopathy and nephropathy. Smoking is potentially diabetogenic: it is a risk factor for the development of type 2 diabetes, and can influence glycaemic control by impairing insulin sensitivity and glucose tolerance. Smoking is at least as prevalent in patients with diabetes as in the general population and young women in particular may perceive the habit as an adjunct to weight management. Many approaches to the supportive management of patients have been described. These include smoking-cessation clinics and nicotine replacement therapy. Such forms of therapy apply equally to people with and without diabetes. Structured educational programmes have been developed in Europe and North America. In the UK, a five-day intensive educational course called the Diet Adjustment For Normal Eating (DAFNE) programme provides opportunities for support and learning for patients with type 1 diabetes. This course teaches those with type 1 diabetes how to adjust their insulin doses according to carbohydrate portion intake, and allows participants to learn about aspects of lifestyle and management related to their diabetes. For patients with newly diagnosed type 2 diabetes, the Diabetes Education and Self Management for Ongoing and Newly Diagnosed (DESMOND) programme offers support to patients in identifying their health risks and enables goal-setting, developing confidence in self-management of their condition. Despite the fact that patients with diabetes are uniquely susceptible to microvascular complications, around 80% of patients with type 2 diabetes will die of macrovascular disease. Ischaemic heart disease, stroke and peripheral vascular disease are some 2–4 times more common in patients with diabetes than in the non-diabetic population. Many available risk tables do not even include powerful diabetes-related risk factors such as microalbuminuria. Type 2 diabetes is associated with dyslipidaemia. There are elevated concentrations of small, dense LDL particles that are more prone to oxidation, which increases their ability to damage the vascular endothelium. Reduced high-density lipoprotein (HDL) concentrations result in reduced protection against atheroma: increased triglyceride-rich lipoprotein concentrations predispose to atheroma formation. The risk of mortality from cardiovascular disease is higher in patients with diabetes and increases further with additional cardiovascular risk factors such as hypercholesterolaemia, hypertension and smoking. Diabetes is so powerful a risk factor that patients should, in most cases, be treated as if they had already suffered a cardiovascular event, rather than have their treatment assessed on the basis of primary prevention studies. Apart from recommendations regarding the dietary and other lifestyle measures detailed above, there is a degree of uncertainty about which patients should receive preventative treatment with agents such as aspirin, lipid-lowering therapy or angiotensin-converting-enzyme (ACE) inhibitors, and what targets to pursue at any specific stage of the disease. Guidelines have been prepared by various organizations in countries with populations of differing genetic background and socioeconomic structures. None can obviate the need for individualized risk–benefit assessment, and it is important to involve patients in setting their own treatment goals, and management plans. It is likely that future guidelines will recommend a lifetime risk calculator that uses a range of risk factors to calculate the absolute risk of an event over the rest of the patient’s lifetime. This has the effect of promoting more aggressive intervention to modify risk factors from an early age. Risk calculators can also be used to help patients to understand their personal risk and how changes can affect it, in order to improve compliance. Aspirin (75 mg daily) is recommended for secondary prevention of cardiovascular disease in patients with diabetes. It is effective in reducing cardiovascular morbidity and mortality in high-risk patients with previous cardiovascular disease. The risk of adverse effects associated with this dose of aspirin depends on many factors, including the age of the patient, intake of other drugs (corticosteroids and non-steroidal anti-inflammatory drugs), presence of other disease (e.g. untreated proliferative retinopathy, uncontrolled hypertension, asthma) and medical treatment (e.g. forthcoming surgery). The risks of major gastrointestinal haemorrhage appear to be increased by a factor of 1.5–2 with approximately one additional event per 500 patient years under trial conditions (approximately 0.2% per patient per year). Intracranial haemorrhages are increased by one event per 3–5000 patient years. The use of aspirin for secondary prevention of macrovascular disease in all patients in whom it is not contraindicated is well established. However, in primary prevention in patients with diabetes with no prior cardiovascular events, the use of aspirin has been more contentious. The current Joint British Societies guidance (JBS2) recommends aspirin 75 mg daily in patients with diabetes aged > 50 years, those who are younger but have had the disease for more than 10 years, and those who are already receiving treatment for hypertension, with controlled blood pressure < 150 mmHg systolic and < 90 mmHg diastolic. The UK National Institute for Health and Care Excellence (NICE) guidelines have stated that low-dose aspirin should be offered to patients aged > 50 years if blood pressure is below 145/90 mmHg and to those aged < 50 years who have significant cardiovascular risk (features of the metabolic syndrome, strong early family history of cardiovascular disease, smoking, hypertension, existing cardiovascular disease or microalbuminuria). However, a more recent meta-analysis found no significant beneficial effects of using aspirin for primary prevention of cardiovascular disease in diabetes, and the Prevention of Progression of Arterial Disease and Diabetes (POPADAD) study demonstrated that aspirin did not significantly reduce the risk of major cardiovascular events even in patients with asymptomatic peripheral arterial disease. At present, there is little evidence to support the use of aspirin in patients aged < 30 years and, in those < 16 years, aspirin should be avoided because of an increased risk of Reye syndrome. Effective management of dyslipidaemia reduces the risk of cardiovascular disease; plasma lipid concentrations should be monitored at least annually in patients with diabetes. Most people in the developed world, with or without diabetes, would benefit from a less atherogenic lipid profile. Lifestyle modifications such as dietary restriction of saturated fat, cholesterol and trans-unsaturated fat, increased intake of ω-3 fatty acids, viscous fibre and plant sterols, achievement and maintenance of an ideal body weight and taking adequate exercise are recommended, whether or not further treatment is required. Secondary causes of dyslipidaemia such as alcohol excess, hypothyroidism or liver disease should be considered in all patients prior to initiating any lipid-lowering therapy. For patients with diabetes, poor glycaemic control (denoting inadequate insulin action) may also cause excess very low density lipoprotein (VLDL) production and hypertriglyceridaemia. Treatment with metformin, pioglitazone and insulin have all been shown to improve this, either via increasing insulin action or by other specific mechanisms (e.g. by reducing the flux of non-esterified fatty acids to the liver in the case of pioglitazone). Many primary and secondary prevention trials have convincingly demonstrated the benefits of lipid-lowering therapy in reducing macrovascular risk in subjects with and without diabetes. Many of these studies have involved the use of 3-hydroxy-3-methylglutaryl-CoA (HMG-CoA) reductase inhibitors (statins), and have shown that lowering LDL-cholesterol is approximately linearly related to risk reduction down to concentrations of 2.2 mmol/L or even below. The American Diabetes Association (ADA) currently recommends statin therapy, in addition to lifestyle factors, as secondary prevention for patients with diabetes who have cardiovascular disease, with a goal for LDL-cholesterol of < 1.8 mmol/L. Treatment is recommended for primary prevention in all patients with diabetes over the age of 40, and in younger people who have additional cardiovascular risk factors, with an LDL-cholesterol target of < 2.6 mmol/L. Statins should be used with caution, and only on specialist advice, in children and are contraindicated in pregnant women. The typical diabetic dyslipidaemia comprises raised triglycerides, intermediate density lipoprotein (IDL) and small, dense LDL concentrations together with low HDL concentrations. These abnormalities are particularly atherogenic, with the result that the significance of dyslipidaemia in diabetes is underestimated by routine measurements of total lipid subfractions. Fibric acid derivatives have a role in the management of the raised triglycerides in diabetic dyslipidaemia. The ADA currently recommends targets for triglycerides of < 1.7 mmol/L, and for HDL-cholesterol of > 1.0 mmol/L in men and > 1.3 mmol/L in women. Both the JBS2 and NICE guidelines (NICE Clinical Guideline 66) recommend a target total cholesterol < 4.0 mmol/L and LDL < 2.0 mmol/L. Alternatively, JBS2 recommends a 25% reduction in total cholesterol with a 30% reduction in LDL-cholesterol, if this achieves lower absolute concentrations. Both guidelines recommend statins for those with diabetes who are aged > 40 years and for those aged 18–39 years with diabetes complications (retinopathy and nephropathy), poor glycaemic control, hypertension, features of the metabolic syndrome or family history of premature CVD in a first degree relative. Combination lipid-lowering therapy, to achieve additional lowering of LDL, may be considered in order to reach desired targets if these are not reached with maximum tolerated statin doses. To date, however, there is little evidence to show that combination therapy to lower LDL produces significant cardiovascular risk reduction over and above statin therapy alone. We would advocate a strategy of: (1) assessing overall risk and choosing targets for LDL, triglyceride and HDL; (2) excluding secondary causes of hyperlipidaemia and optimizing glycaemic control; (3) optimizing lifestyle options, and (4) targeting LDL-cholesterol with a statin. These drugs may in addition produce minor favourable effects on HDL-cholesterol and triglycerides. It should be noted that therapy targeting HDL or triglycerides does not have the evidence base of statin treatment. Severe hypertriglyceridaemia requires lifestyle and pharmacological therapy. A fibrate or ω-3 fatty acid-rich marine oils may be used to reduce the risk of pancreatitis associated with high triglycerides. Any statin–fibrate combination requires care because of the potentially increased risk of rhabdomyolysis, and monitoring of plasma creatine kinase activity may be appropriate. Assiduous control of blood pressure (BP) has been shown to reduce major cardiovascular events and minimizes progression of diabetes complications. In the UK Prospective Diabetes Study (UKPDS), intensive treatment of hypertension to a median of 144/82 mmHg was compared with conventional BP treatment (median BP in the control group was 154/87 mmHg). Intensive treatment resulted in reductions in multiple diabetes-related endpoints, including death. The majority of subjects in the tight control arm of the study required three or more agents in order to achieve this. The ADA has adopted an even more aggressive target of 130/80 mmHg on the basis of epidemiological risk studies showing an inflexion in the relationship between BP in patients with diabetes and cardiovascular risk at 115/75 mmHg. Apart from more rigorous treatment goals, management of hypertension in patients with diabetes is similar to that in patients without the condition. Initially, lifestyle changes are recommended including weight loss, exercise and dietary modifications, such as a reduction in sodium and alcohol intake and increased consumption of fruit and vegetables. However, if blood pressure remains inadequately controlled despite lifestyle modifications, pharmacological therapy may be commenced. Some patients will have comorbid conditions that will direct the choice of drug therapy. The presence of microalbuminuria, proteinuria, mild to moderate renal impairment, diabetic retinopathy, ischaemic heart disease, stroke and left ventricular systolic dysfunction would, for example, indicate a specific role for an angiotensin-converting-enzyme (ACE) inhibitor or angiotensin-II receptor antagonist (blocker) (ARB). Given the potentially beneficial effects of ACE inhibitors and ARBs on glycaemia and in the prevention of diabetic complications such as diabetic nephropathy (see below), they are generally considered as first-line agents in patients with diabetes if lifestyle changes do not improve blood pressure. β-Blockers, diuretics, and calcium channel blockers have all been shown to reduce cardiovascular events in patients with diabetes, and all may be used in combination with an ACE inhibitor or ARB. Most patients will require multiple agents to manage their hypertension. Generally initial treatment will be with either an ACE inhibitor or ARB, and if required, a calcium channel blocker, then a thiazide diuretic, β-blocker, α-blocker or potassium-sparing diuretic. It is important to monitor renal glomerular function and plasma potassium concentration whenever ACE inhibitors, ARBs or diuretics are used. Thiazides are effective, but unwanted effects including hyperglycaemia, erectile dysfunction, gout and dyslipidaemia have reduced their use as first line agents. β-Blockers are specifically indicated in some situations, for example stable heart failure and ischaemic heart disease, and were found to be safe and effective in the UKPDS. However, they may promote weight gain and, in some patients with impaired awareness, hypoglycaemia may be a problem. In the Anglo–Scandinavian Cardiac Outcome Trial (ASCOT), treatment with an atenolol–thiazide-based regimen was associated with 30% greater incidence of new type 2 diabetes than treatment with an amlodipine–perindopril-based regimen, and was less effective at reducing BP and cardiovascular events in patients with established diabetes. In the past, there were concerns about the safety of the dihydropyridine group of voltage activated calcium channel blockers. However, results from the Antihypertensive and Lipid Lowering Treatment to Prevent Heart Attack Trial (ALLHAT) and ASCOT have been reassuring, and it now seems reasonable to recommend amlodipine as a second-line agent in combination with an ACE inhibitor or ARB (although amlodipine and other calcium channel blockers inhibit the metabolism of simvastatin, which must not be co-prescribed at high dose). α-Blockers appear effective and have favourable effects on insulin sensitivity and LDL-cholesterol concentrations. However, ALLHAT showed an apparent doubling in the incidence of heart failure (a secondary outcome measure) with an α-blocker compared with a thiazide diuretic. For this reason, α-blockers are generally reserved for use where treatment with at least two other classes of hypotensives in combination has failed to achieve the target BP. Angiotensin II (ATII) increases hepatic glucose production and decreases insulin sensitivity. The use of ACE inhibitors or ARBs consistently increases insulin sensitivity by some 15–20% in pharmacological studies. The Heart Outcomes Prevention Evaluation (HOPE) study was terminated early after interim analysis demonstrated that 4.5 years of treatment with the ACE inhibitor ramipril, 10 mg daily, reduced major vascular events and death in subjects with preserved left ventricular function, and either pre-existing vascular disease or those with diabetes and an additional vascular risk factor. The latter group also had a reduced risk (relative risk 0.84) for the development of diabetic complications. In an extension to the study to a total of 7.2 years follow-up, there was a 31% relative risk reduction for new diagnosis of type 2 diabetes. Results of other studies and meta-analyses have, in general, not shown such large benefits in patients who already have diabetes (unless with other clearly established indications), although they have generally been restricted to relatively small subgroup analyses. While it is not clear whether all (non-pregnant) patients with diabetes should be offered these agents, ACE or AT-II inhibition is clearly indicated for subjects with diabetes and hypertension, microalbuminuria, proteinuria, mild to moderate renal impairment, diabetic retinopathy, ischaemic heart disease, stroke or left ventricular systolic dysfunction. Caution must be exercised with the use of these agents when renal artery stenosis is present (e.g. in patients with impaired renal function and absent distal pulses or a renal bruit) and in subjects with hyporeninaemic hypoaldosteronism (type 4 renal tubular acidosis), in whom dangerous hyperkalaemia may result. Trials in both type 1 Diabetes Control and Complications Trial (DCCT) and type 2 (UKPDS) diabetes have confirmed that microvascular, and, to a lesser extent, macrovascular, complications of diabetes may be delayed or even prevented by tight glycaemic control. The UKPDS (1998) trial in type 2 diabetes found that a 10 mmol/mol (0.9%) reduction in HbA1c from 63 to 53 mmol/mol (7.9–7.0%) over a median follow-up of ten years from diagnosis led to a reduction of 12% for any diabetes-related endpoint, 25% for microvascular endpoints and 24% for cataract extraction, and of 21% for retinopathy and 33% for albuminuria at 12 years. The improvements in macrovascular disease were less convincing and of borderline statistical significance. The DCCT (1993) trial in type 1 diabetes showed a risk reduction of 26–63% in microvascular disease with intensive insulin therapy, but at the cost of a three-fold elevation in the incidence of severe hypoglycaemia (fits, unconsciousness) and some additional weight gain compared with the control arm of the study. However, the use of more modern insulin analogues has been associated with lower incidence of hypoglycaemia. Patients who have been diagnosed with type 1 diabetes will require insulin therapy along with general measures such as dietary and lifestyle changes. Although it is often considered that patients with type 1 diabetes have no useful islet cell function, this is not entirely true. In fact, β-Cell function is approximately 10% of normal at disease presentation, and may double after the initiation of insulin therapy, resulting in a reduced requirement for exogenous insulin and stabilization of glucose metabolism (‘honeymoon effect’). This effect does not last and, in the majority of patients, insulin secretion declines again over the next 2–5 years. The ‘honeymoon effect’ may reflect, in part, the amelioration of glucotoxicity or lipotoxicity (see Chapter 15) on the reduced numbers of metabolically stressed β-Cells. After initiation of insulin therapy, the toxic effects of hyperglycaemia are removed, thereby relieving this metabolic stress. In some studies, as many as 10% of patients with newly diagnosed type 1 diabetes have been maintained off insulin altogether for the first year, but this is clearly not recommended in routine clinical practice because of the risks of ketoacidosis. In a subset (~ 15%) of patients, there is retention of some detectable islet function, as assessed by C-peptide secretion, for at least 40 years. These individuals also appear to have reduced susceptibility to severe diabetic ketoacidosis (DKA), less risk of retinopathy, a more robust glucagon response to hypoglycaemia and lower average insulin requirements. A number of trials have attempted to augment residual insulin secretion with immunosuppressive drugs (e.g. steroids, azathioprine, antithymocyte globulin and ciclosporin) or by plasmapheresis, and these measures have been met with some success but, on current evidence, their benefits have not been clearly outweighed by their risks. Studies using strict metabolic control in order to mitigate any effects of lipotoxicity or glucotoxicity on the β-Cell (e.g. with continuous subcutaneous insulin infusion, CSII) have been able to demonstrate transiently preserved insulin secretion, but only for a period of a few months, with rapid regression on discontinuation of strict metabolic control. Regular human insulin (e.g. Actrapid®, Humulin S®) has an onset of activity within 30–60 min after subcutaneous injection, a variable peak concentration at around 2–4 h, and a duration of action of 5–8 h, contrasting with its duration of action of only a few minutes when administered intravenously. This difference may be explained by the tendency for hexamerization in solution. The hexameric form of regular insulin has to dissociate into dimers or monomers in order to be absorbed into the bloodstream. This is rate-limiting for its absorption and influences the pharmacokinetic profile. As such, in order to treat prandial glucose peaks, regular insulins have to be injected about 30 min before each meal so that the onset of action coincides with the rise in prandial glucose concentrations. More recent developments in insulin technology have focused on the design and production of insulin analogues whose pharmacokinetic profiles are determined by modifications to non-receptor-binding regions of the insulin molecule. Insulin aspart (Novorapid®) is homologous with regular human insulin with the exception of a single substitution of aspartate for proline in position B28 of the B chain: it is produced by recombinant DNA technology in Saccharomyces cerevisiae (baker’s yeast). Insulin lispro (Humalog®) is identical to normal human insulin except that the positions of the amino acids proline and lysine at B28 and B29 are reversed. In insulin glulisine (Apidra®), asparagine is replaced by lysine at position B3, with glutamic acid replacing lysine at B29. As a result of these changes in amino acid sequence, the rapid insulin analogues (aspart, lispro and glulisine) have a lower tendency to form hexamers, permitting more rapid absorption and a faster onset of action (< 15 min), enabling injection immediately before, or just after, eating. There is a sharper peak, more closely resembling first-phase insulin secretion in the non-diabetes state, and a shorter duration of action (3–5 h), which may reduce the incidence of hypoglycaemia before the next meal is ingested. Isophane (NPH, Neutral protamine Hagedorn) is an intermediate-acting insulin that is a combination of soluble insulin and protamine zinc. Protamine delays the onset of action and prolongs the effects of the soluble insulin. The onset of action is within two hours, with a duration of action of 10–20 h. Most patients require two subcutaneous injections daily to provide adequate glycaemic control. Isophane has fallen out of favour because of increased risks of nocturnal hypoglycaemia and fasting hyperglycaemia. The most commonly prescribed premixed insulins are a mixture of quick acting analogue and an insulin analogue protamine complex (e.g. Novomix 30® is composed of insulin aspart-protamine complex and insulin aspart in a ratio of 70:30). These are usually given as a twice-daily regimen. Longer-acting insulin analogues (insulin glargine and insulin detemir) are produced by genetic engineering. The onset of action is within two hours and they have a longer duration of action of up to 24 h. These insulins provide a steady basal insulin profile with minimal peak action and are injected subcutaneously once daily. In insulin glargine, asparagine is replaced by glycine at position 21 of the α chain of the insulin molecule and the carboxyl terminal of the β chain is changed with the addition of arginine. This insulin is slowly released into the circulation because of its acidic pH, which causes it to precipitate when injected subcutaneously. In insulin detemir, tyrosine at position B30 is lost with acylation at B29 that results in a fatty acid side chain (tetradecanoic acid) becoming linked with lysine. With these alterations, insulin detemir forms hexamers with slower dissociation and a longer duration of action. Most patients with type 1 diabetes use an insulin replacement regimen that comprises multiple-dose subcutaneous injections (three to four injections per day) consisting of basal and prandial insulin. Insulin is injected subcutaneously. Short-acting insulin (regular soluble or analogue) acts to control prandial blood glucose concentrations; long-acting insulin provides a constant background basal insulin concentration. This combination of short-acting and long-acting basal insulin is called the basal bolus regimen or multiple daily injection therapy. Most people take their long-acting insulin at bedtime in order to provide optimal glycaemic control overnight. Some patients learn how to adjust insulin doses according to carbohydrate intake (in the UK often through attendance at a DAFNE (dose adjustment for normal eating) course). An alternative is a twice daily insulin regimen consisting of a premixed or biphasic mixture of longer- and shorter-acting insulins in ratios of 75:25, 70:30 or 50:50. Such regimens are commonly used in type 2 diabetes and in patients with type 1 diabetes before they progress to a basal bolus regimen. Some patients with type 1 diabetes prefer twice daily insulin to the basal bolus regimen, particularly those who dislike frequent injections, but it is not suited to those whose diet and activities tend to differ on a day-to-day basis, as there is less scope for dose adjustment in relation to carbohydrate intake, with attendant risks of hypoglycaemia. The longer-acting component may not provide effective glycaemic control overnight because of a waning of the effect of the previous evening’s dose, a decrease in insulin sensitivity in the early hours of the morning, and increases in growth hormone and cortisol concentrations, all of which will increase the risk of early morning hyperglycaemia (the ‘dawn’ phenomenon). Premixed insulin preparations are, therefore, not recommended for long-term treatment in type 1 diabetes and most patients are eventually recommended to convert to the basal bolus regimen for optimal glycaemic control. The relative merits and disadvantages of these two regimens, and a comparison with continuous subcutaneous insulin infusion, are outlined in Table 16.1. Continuous subcutaneous insulin infusion pumps (‘insulin pumps’) are portable devices that deliver a continuous subcutaneous infusion of short-acting insulin at a set rate. The pump is battery-driven and comprises a reservoir containing about 3 mL of insulin in a syringe. A syringe driver delivers insulin via a cannula that is inserted subcutaneously into the abdominal wall. The cannula is re-sited every 1–2 days to avoid skin infections. In addition to the continuous basal infusion, the patient triggers the delivery of an additional dose at mealtimes to provide prandial cover. The advantages of a pump include having a basal insulin rate that is personalized to each individual, allowing more flexibility with regards to mealtimes as the basal rate can be supplemented with a corrective mealtime insulin dose according to the carbohydrate consumed. Studies have shown improved glycaemic control in pump users. However, in the event of pump failure, there is the risk of diabetes ketoacidosis occurring. Clinical indications for pump therapy include poor glycaemic control despite high levels of care, disabling hypoglycaemia on multiple daily injection regimens, recurrent unpredictable hypoglycaemia, especially in patients with severe dawn phenomenon and pregnant women without acceptable glycaemic control with subcutaneous insulin. Before each patient is commenced on the insulin pump, they must attend a comprehensive educational pump course. They must be sufficiently motivated to use the pump, with a commitment to regular blood glucose monitoring, attendance at pump clinics and adherence to carbohydrate counting so that they will be able to manage their pump. Close liaison with the diabetes pump team is essential for the success of this form of therapy. It should be stressed that when deciding which regimen to use, each patient’s specific circumstances will need to be carefully considered in light of different needs, goals and capabilities and the level of support available. An important factor is the risk of hypoglycaemia that comes with insulin therapy. There should be a balance between achieving optimal glycaemic control whilst at the same time ensuring each patient’s safety, in terms of hypoglycaemia risk, when deciding on which insulin and regimen is to be used. The recommended subcutaneous injection sites include the abdomen, upper outer thighs, upper outer arms and buttocks. It is important to avoid injecting insulin intramuscularly. This commonly occurs in the limbs of slim individuals or children and can influence insulin absorption and hence blood glucose profiles. As a result of the trophic action of insulin, an accumulation of fat, called lipohypertrophy, may occur at sites of repeated subcutaneous insulin injections. Lipohypertrophy can affect insulin absorption, causing problems such as hypoglycaemia, but can be avoided by rotating injection sites. Insulin injection sites should be inspected when patients attend for their diabetes review. When type 2 diabetes is diagnosed, lifestyle modification should be advised initially, and metformin commenced in combination with lifestyle if HbA1c does not reach target after three months. If glycaemic control remains poor after 3–6 months, further oral agents, or a glucagon-like peptide 1 (GLP-1) receptor agonist or insulin may be added. Some patients with marked hyperglycaemia may be commenced on insulin at diagnosis if it is deemed that non-insulin agents will not provide the necessary control of blood glucose. Metformin belongs to a class of drugs called the biguanides that act to reduce insulin resistance, therefore improving insulin sensitivity. Metformin is the only biguanide currently available. It has an established role in the treatment of type 2 diabetes in combination with diet and lifestyle measures. The UKPDS study showed that obese patients treated with metformin had reduced myocardial infarction (by 6%), stroke (by 3%) and mortality (by 7%). These effects were independent of improved glycaemic control. Metformin has the clear advantage over many other drugs of improving glycaemic control without causing weight gain. Metformin does not cause hypoglycaemia and may even lead to weight loss by suppressing appetite. As such, it is often prescribed as first line treatment in patients who are overweight. Several of the adverse features of the metabolic syndrome, including raised circulating concentrations of non-esterified fatty acids (NEFAs), triglycerides and plasminogen activating inhibitor-1 (PAI-1), are improved by metformin. Metformin has an effect on blood glucose that is additive to, and broadly equivalent to, that of sulfonylurea monotherapy. In type 2 diabetes, metformin can be used as monotherapy, or combined with insulin or with sulfonylureas, dipeptidyl peptidase IV (DPP-4) inhibitors, GLP-1 analogues or thiazolidinediones. In type 1 diabetes it is sometimes used together with insulin for obese adults, although this usage is currently unlicensed. Metformin may also be considered in patients who are not overweight. Metformin has a useful role in diabetes prevention; a 31% relative reduction in the progression of diabetes was observed in the Diabetes Prevention Programme (DPP) placebo-controlled study, with greatest effect in younger and more obese subjects. Metformin also has a possible role in the treatment of polycystic ovary syndrome (PCOS), alone or in combination with drugs acting on sex steroid metabolism, particularly in those patients who are also glucose intolerant. Metformin decreases hepatic gluconeogenesis and glucose output, improves peripheral glucose uptake and utilization in insulin-sensitive tissue such as muscle and adipose tissue, and may reduce intestinal glucose transport. Its actions depend on the presence of adequate β-Cell function, and therefore insulin in the circulation. The exact mechanism of action of metformin is not clear but it may work through the activation of an intracellular AMP-activated protein kinase. Lactic acidosis is a rare but serious complication that can occur whilst taking metformin. Lactic acidosis was more frequently reported with phenformin, another biguanide that was subsequently withdrawn in most countries in 1977 after 306 documented cases. While phenformin excretion relies upon hepatic hydroxylation (pharmacogenetically deficient in approximately 10% of Caucasians), metformin is subject to renal tubular secretion, and its excretion depends only on renal function. Lactic acidosis presents with non-specific symptoms such as lethargy, nausea, vomiting, altered level of consciousness and abdominal pain. Biochemical features of lactic acidosis are those of an elevated anion gap metabolic acidosis with high blood lactate concentrations. There appears to be no direct correlation between blood concentrations of metformin and lactate, and the overwhelming majority of cases of lactic acidosis have occurred in the setting of major comorbidities (Table 16.2). These include conditions associated with increased lactate formation (e.g. cardiac failure, pulmonary disease), reduced lactate metabolism (e.g. hepatic failure) and reduced metformin and lactate excretion (e.g. renal failure). TABLE 16.2 Cautions with and contraindications to the use of metformin There is an increased risk of lactic acidosis in patients treated with metformin who receive radiological contrast media. This may be avoided by withholding metformin for a few days before and after the intended radiological procedure. A systematic review has shown that there is no evidence from prospective comparative trials or from observational cohort studies that metformin is associated with an increased risk of lactic acidosis, or with increased plasma concentrations of lactate, compared with other antihyperglycaemic treatments, if prescribed as licensed. Patients with significant cardiac, renal or liver dysfunction should not receive metformin. As metformin is renally excreted, there is the attendant risk of accumulation in acute or chronic kidney disease, resulting in an increased risk of lactic acidosis. It is recommended by NICE that prescription of metformin should be reviewed in patients with a glomerular filtration rate (GFR) < 45 mL/min/1.73m2 and it is to be avoided in those with a GFR < 30 mL/min/1.73 m2. Some physicians recommend that metformin should not be used if the patient’s plasma creatinine concentration is raised to any extent; others argue that guidelines have been progressively tightened with no reduction in the incidence of lactic acidosis, which has anyway always been very low throughout the history of metformin usage. Although some formularies suggest avoiding metformin when plasma creatinine concentration is raised above certain thresholds, cardiac dysfunction is probably a more important, but less well recognized, contraindication to its use. The major side-effects of metformin include gastrointestinal problems such as nausea, abdominal discomfort, metal taste and diarrhoea. Many patients will experience these symptoms after commencing metformin but will eventually develop tolerance. These side-effects are generally dose dependent, and can be minimized by commencing at a low dose and then slowly titrating the dose upwards, or by using a slow-release preparation. Approximately 80% of the total benefit of metformin is seen with doses of around 1.5 g/day, which is close to the median tolerated dose. Metformin induces malabsorption of vitamin B12 and folate but this is rarely clinically significant. First generation sulfonylurea (sulphonylurea) derivatives were first used to treat diabetes in the 1950s, following the observation in 1942 that certain sulphonamide antibiotics could provoke severe hypoglycaemia in patients treated for typhoid fever. They act as insulin secretagogues, reducing blood glucose concentrations by augmenting the first-phase release of immediately releasable (reserve) insulin from β-Cells. Unlike glucose-stimulated insulin release, there is little direct effect on insulin production. First generation sulfonylureas, such as chlorpropamide, were beset with unwanted effects including the syndrome of inappropriate antidiuresis (SIADH) and flushing. These drugs have largely been replaced by more potent and shorter acting second and third generation drugs (e.g. gliclazide) that lack these unwanted effects. Central to the release of insulin from secretory granules (but not the only mechanism involved) is the closure of an ATP-sensitive membrane-bound inwardly rectifying 140 kDa K+ channel, KATP. In β-Cells, this channel is a hetero-octamer of pore-forming units (KIR 6.2) together with regulatory ATP binding cassette (ABC) protein sulfonylurea receptor 1 (SUR-1) molecules. The regulatory subunit, SUR-1, contains 13–17 transmembrane domains with a large number of possible protein kinase A or C phosphorylation sites, together with a number of distinct binding sites for other molecules (including the A and B sites to which sulfonylureas and other exogenous compounds attach, as discussed below). The existence or nature of the endogenous ligand at sulfonylurea binding sites is not clear. Closure of KATP channels depolarizes the plasma membrane, causing rapid influx of calcium ions via voltage dependent calcium channels. The resultant increase in free ionized cytosolic calcium concentration ([Ca2 +]i) triggers cytoskeletal trafficking of secretory granules to the plasma membrane and release of insulin by a process of exocytosis. Amplification of the signal generated by KATP closure occurs via a number of incompletely understood mechanisms, including direct mobilization of calcium from intracellular stores (e.g. by the action of glucagon-like peptide (GLP)-1 analogues). The effects of KATP channel opening are terminated by extrusion of K+ via a voltage gated K+ channel. The principal mechanism of action of the sulfonylurea drugs is to close the KATP channel by binding to the A and B sites on SUR-1. Conversely, diazoxide, used in the treatment of certain hyperinsulinaemic conditions, is a potent opener of KATP channels, and thiazide diuretics also open β-Cell KATP channels (causing impairment of glucose tolerance). Concern has existed for many years that sulfonylurea drugs may have harmful cardiovascular effects (mainly arrhythmias). This concern was first raised in relation to tolbutamide (the University Group Diabetes Programme, UGDP). The action of sulfonylureas on cardiac KATP channels suggests a plausible mechanism for adverse cardiac effects.
The clinical management of diabetes mellitus
INTRODUCTION
GENERAL ASPECTS OF MANAGEMENT
Nutrition
Exercise
Smoking cessation
Education about diabetes
Pharmacological management of cardiovascular risk
Aspirin
Lipid-lowering agents
Hypertension
Angiotensin-converting-enzyme inhibitors and angiotensin-II receptor antagonists
GLUCOSE-LOWERING THERAPY IN DIABETES
Background
Insulin use in type 1 diabetes
Regular insulin
Insulin analogues
Intermediate-acting insulin
Premixed insulin analogues
Long-acting insulin analogues
Insulin regimens
Continuous subcutaneous insulin infusion
Insulin administration
Glycaemic management in type 2 diabetes
Metformin
Mechanism of action
Lactic acidosis
Caution or contraindication
Reason
Old age
Renal and other organ impairment
Tissue hypoxia
Increased lactate production via anaerobic glycolysis, reduced gluconeogenesis
Pulmonary disease
Increased lactate production via tissue hypoxaemia and anaerobic glycolysis
Liver failure, ethanol
Reduced lactate clearance via inhibition of gluconeogenesis
Iodinated radiological contrast media
Risk of acute kidney injury, competition for renal tubular secretion
Cardiac impairment
Increased lactate production via poor tissue oxygen delivery
Shock states, severe dehydration
Increased lactate production via poor tissue oxygen delivery
Advanced microvascular disease
Risk of chronic kidney disease
Severe infection
Increased lactate production
Drugs affecting tubular secretion of metformin
Contrast media, cimetidine, digoxin, others
Type 2 diabetes itself
Microcirculatory disturbances, peripheral vascular disease, increased incidence of cardiac and renal disease, reduced activity of pyruvate dehydrogenase in insulin resistance favouring anaerobic metabolism
Other unwanted effects of metformin
Sulfonylureas (and related insulin secretagogues)
Mechanism of action
Adverse effects of sulfonylureas
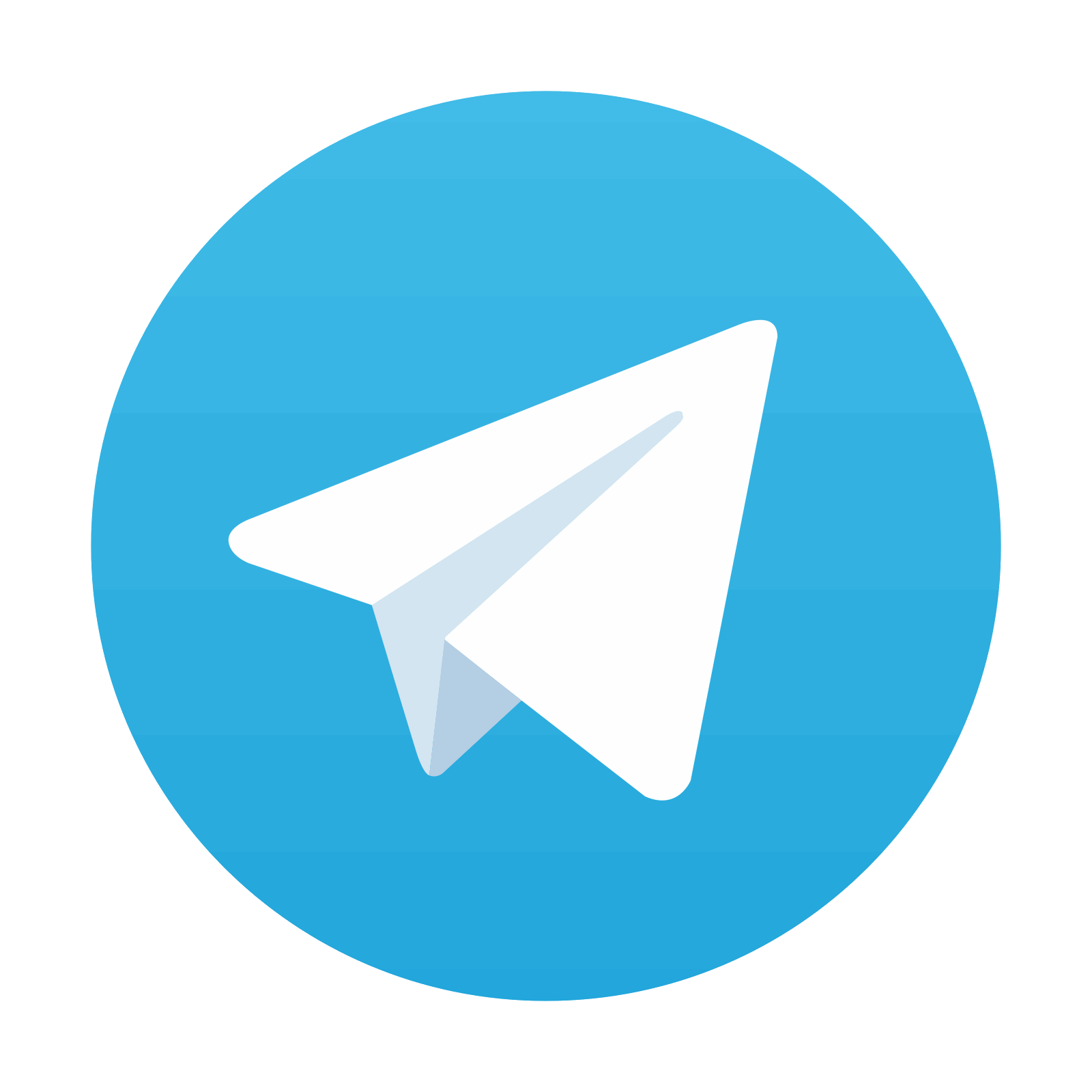
Stay updated, free articles. Join our Telegram channel

Full access? Get Clinical Tree
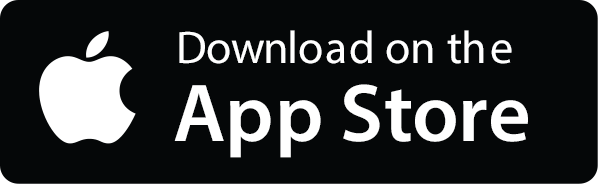
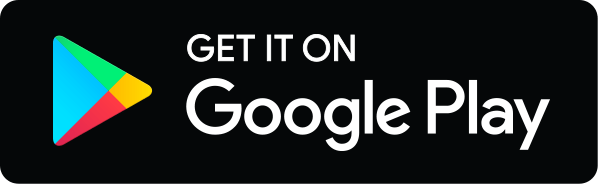