Mimicking the Hematopoietic Stem Cell Niche by Biomaterials
Leibniz Institute of Polymer Research Dresden, Max Bergmann Center of Biomaterials, Dresden, Germany
Universität Leipzig, Institute of Biochemistry, Leipzig, Germany
Leibniz Institute of Polymer Research Dresden, Max Bergmann Center of Biomaterials, Technische Universität Dresden, Center for Regenerative Therapies Dresden, Dresden, Germany
Leibniz Institute of Polymer Research Dresden, Max Bergmann Center of Biomaterials, Dresden, Germany; Universität Leipzig, Institute of Biochemistry, Leipzig, Germany
16.1 Introduction
In the adult mammalian organism, hematopoietic stem cells (HSCs) reside primarily in the bone marrow (BM) and are the source for regeneration of all immune and blood cells. In order to fulfill this task, they have the ability to balance proliferation and quiescence as well as differentiation and self-renewal during the entire human life span, giving rise to a daily production of a trillion differentiated blood cells.
For that reason, the hematopoietic systems and especially the origin of their regeneration potential—the HSC—have attracted many efforts to explore regulating mechanisms in vivo and in vitro. Much evidence has been accumulated about the role of extrinsic signals in controlling HSC fate. The so-called niche concept refers to the physical and functional environment responsible for the convergence and integration of such signals [1]. Based on this concept, the HSC microenvironment is thought to encompass several stromal cell types, extracellular matrix (ECM) components, and a multitude of paracrine and endocrine signals. The organizational principles underlying the spatial arrangement of HSCs, osteoblasts, vasculature, and ECM, and in particular, their impact on the dynamics of HSC localization, function, and fate, are still not completely understood.
Recent progress in biotechnology and biomaterials science has fostered engineering approaches to explore the niche concept by means of bioartificial concepts in vitro. Here, specific cues of the in vivo BM are modeled in simple or complex setups with the aim of applying the full range of in vitro bioanalytical tools for the analysis of HSC behavior in such microenvironments. This chapter focuses on an introduction to existing HSC niche concepts and summarizes approaches to mimic them in an experimental manner ex vivo.
16.2 Concepts of HSC Niches
Due to their unique regenerative potential, stem cell transplantations have become an attractive approach for the clinical therapy of numerous diseases such as cancer, heart failure, autoimmune disorders, or diabetes. As a consequence, the regulatory processes underlying stem cell growth and differentiation have gained a lot of interest. The local microenvironment—referred to as a stem cell niche—is widely accepted to play a major role in controlling stem cell fate [2]. The function of the niche is seen as a tight balance between proliferation and quiescence, and self-renewal and differentiation, regulated by cell intrinsic and extrinsic mechanisms, as sketched in Figure 16.1.

Similar to stem cell niches in other tissues, a stem cell niche is thought to control HSC fate decisions and is consequently involved in homeostasis of the blood system (Figure 16.1A). The clinical significance of the unique, local microenvironment surrounding HSC is evident from the involvement of alterations of this microenvironment in many diseases, for example, leukemia [3–5]. However, in contrast to other tissue types with an anatomically defined microenvironment for stem cells, the mammalian HSC niche is still only vaguely defined, as reflected by the ongoing debate regarding its localization and composition [6–10]. To date, there is evidence for the existence of at least two different characteristic regulatory regions within the BM [1]: the osteoblastic niche [11], located close to the endosteum, and a location known as the perivascular niche that is associated with the vasculature [12]. As key players of the niches, multiple stromal cells have been identified, in particular osteoblasts [13], (peri)vascular cells [14,15], and nestin-positive progenitors [16]. Apart from different cell types, the regulatory role of various ECM components has also been shown (Figure 16.1B) [17]. However, it is still unclear how many niches we have to consider when studying HSCs and how HSCs navigate between them. The most proposed model suggests that the osteoblastic niche provides long-term dormancy, and the perivascular niche is an intermediate home for activated HSCs that can generate differentiated progenitor cells or revert them to dormancy [18,19].
The complexity of the niche is reflected by the identification of numerous molecular pathways involved in the cross talk between HSC and its microenvironment (e.g., the notch pathway [13], angiopoietin-1 (ANG-1)-Tie2 [20], stromal cell-derived factor-1 (SDF1)-CXCR4 [21], thrombopoietin (TPO)-Mpl [22], stem cell factor (SCF)-c-Kit [23]). Despite initial reports claiming beta-catenin, the key component of canonical Wnt signaling, to be unnecessary for haematopoiesis [24], a growing number of studies indicate that Wnt signaling does influence HSC function [25–30].
While HSCs were initially assumed to be suspension cells, different adhesion receptors and ligands such as cadherins, integrins, fibronectin, osteopontin, heparan sulfate, and others have been shown to regulate HSC function as well as localization and homing [31–33]. Even though the occurrence of specific ECM components inside the BM was investigated by some groups [17,34], their detailed 3-D distribution is still unclear.
Despite this large amount of data on HSC–niche interaction, the mechanisms controlling HSC fate within different BM regions and cellular environments as well as the systemic interplay of the different niche types are far from being understood. In particular, this refers to the dynamic changes in the niche such as variations in direct cell–cell contacts, the interrelation of ECM components with regulatory molecules secreted by HSC or niche cells, and the spatial organization of niche components. Although little direct evidence has been collected about the effect of niche dynamics on HSC fate [28], niche alterations have been described following BM conditioning, transplantation, and aging [30,35,36]. Moreover, variations in HSC density and HSC progeny should not be underestimated in playing a role in HSC fate regulation. As stem and progenitor cells have distinct metabolic states, and the transition from stem to progenitor cell corresponds to a critical metabolic change, the dynamic balance of energy, oxygen, and redox status, essential for HSC maintenance, must also be considered as an important regulating parameter [37].
16.3 Biomaterial Approaches to Create Biomimetic HSC Niches
The development of artificial microenvironments mimicking important stem cell–niche interactions in vitro has received increased attention during the last decade to gain new insights into the orchestrating and regulatory roles of the niche components. Bioengineered platforms are envisioned to verify hypotheses generated in vivo explicitly under strictly defined conditions. They should help to provide mechanistic insights into HSC regulation, and to explore pronounced effects on HSC fate with minimalistic setups compared with an in vivo situation. Because of the wide range of proven and potential HSC regulators and the complexity of their interactions, high-throughput screening platforms are indispensable in identifying and screening the most relevant components and their multifaceted interrelations in a combinatorial manner.
Engineering approaches for biomimetic HSC microenvironments are based on biomaterial strategies using the full range of available options including decellularized ex vivo matrices, biopolymer-based structures, biohybrid, and fully synthetic materials. For an excellent review on the general topic of biomaterial strategies for engineering extracellular microenvironments, the reader is referred to other recent publications [38]. In this section, we want to highlight topics relevant to the HSC niche concept as given earlier.
Biomaterials have been used to create a suitable microenvironment by providing spatial proximity of cells to each other as well as scaffolding structures, presenting adhesion receptor ligands and growth factors in defined spatiotemporal pattern, producing mechanical signals, and enabling cell-driven matrix reorganization [39]. Mimics of stem cell microenvironments have been developed, which include a wide range of important exogenous cues (see also Figure 16.2):
- spatial and temporal control of the presentation of growth and differentiation factors
- soluble and immobilized gradients of signaling molecules inside scaffolds
- regulation of adhesion ligands by specificity, composition, density, and spacing
- viscoelasticity of the ECM
- scaffold topography ranging from nanometer to micrometer scale
- geometrical constraints and guidance in 2-D and 3-D.

Based on the success in other areas of engineering biomaterials scaffolds, most of these parameters were considered for HSC microenvironments. It has to be mentioned that adhesion-related features are underrepresented in these studies as HSCs were thought to be suspension cells. However, the last 15 years have proven cell–ECM and cell–cell adhesion to be an essential feature of HSC regulation. This change in the understanding of HSC regulation is mirrored by a recent broadening in the range of bioengineering strategies of HSC microenvironments. To date, however, the cues most frequently addressed primarily cover the following:
- growth factor delivery
- adhesion ligand presentation
- growth factor presentation.
Emphasis has been given to the delivery of growth factors, which are often seen as key regulators of the niche. In addition to the common adjustment of levels and combinations of growth factors in conventional cell culture experiments, biomaterials have been used to deliver growth factors from matrices in a controlled manner and to confine the molecules to the surface of cell culture carriers by covalent or noncovalent conjugation. Biomaterial scaffolds have been used to present growth factors in an active orientation to trigger receptor activation in a specific, persistent, and more physiological manner [40,41]. Soft lithography techniques and microfluidics have been applied to present growth factors in spatially and temporally controlled patterns [42]. The gradients of released signaling molecules, like SDF-1, were adjusted by sustainable delivery from biohybrid matrices as an interesting vehicle to control chemotaxis of HSCs to SDF-1 gradients [43,44]. SDF-1 is known as a common signaling molecule binding to the angiopoietin-1 (ANG-1) thrombopoietin (TPO) stem cell factor (SCF) cell receptor. It activates different processes including a guided migration of cells toward the SDF-1 gradient [45]. This signaling pathway HSCs use in vivo to be attracted toward the BM is a process called stem cell homing [14,46].
Although HSCs are considered only weakly adhesive, their adhesion-dependent behavior has been investigated by providing ligand structures of the ECM or cell–cell contacts at biomaterial surfaces. Adsorptive deposition of multicomponent layers of adhesive proteins from culture media (such as fibronectin and vitronectin) or more dedicated modes of immobilization of ECM proteins (e.g., collagen, laminin, or peptide sequences) and receptors of cell–cell contacts were used not only to trigger simple adhesion but also to engage specific receptors of signaling cascades [47–49]. Scaffolds with nanometer substrate features, such as nanofibers or nanoscale-spaced and clustered adhesion ligands, were developed to study the nanotopographical cues in HSC adhesion and regulation [50,51]. These studies suggested an improved adhesion and expansion of HSCs.
Topographical features of the HSC microenvironment in the micrometer range were addressed by in vitro strategies for the presentation of growth factors, ECM components, as well as cell receptors. Microstructures of rigid silicone or soft hydrogel were used to mimic single or multicell microenvironments with an example shown in Figure 16.3 [40,48,49].

Inspired by distinct mechanical properties of the niche, cell culture scaffolds of varying stiffness have been developed to investigate the impact of viscoelastic materials properties on HSC self-renewal [52]. These studies showed that a higher substrate stiffness promotes HSC expansion.
In the context of the design of microstructures for HSC microenvironments, array techniques are currently being developed to implement high-throughput approaches for screening larger sets and combinations of factors in their influence on stem cell dynamics and progeny [53]. These new generations of cell culture platforms build on the rapid progress in robotically controlled microfabrication technologies such as microfluidics, photo, and soft lithography. In this manner, protein microarrays and (a)symmetric immobilized or soluble gradients have been utilized to mimic the spatially controlled display of niche ligands. Recently, a microfluidic platform containing thousands of nanoliter-scale chambers combined with an automated medium exchange enabled the configuration of well-defined culture conditions with respect to cell culture parameters like growth factor concentration [54].
A microfluidic device consisting of an array of hydrodynamic traps hosting single cells was developed to study fate decisions at the single-cell level in combination with screening techniques such as cytometry or on-chip polymerase chain reaction [55,56]. Bio-inspired scaffolds for the expansion of HSCs in combination with novel (semi)automatic time-lapse imaging and single-cell tracking techniques have been applied to microstructured surfaces or in microfluidic devices providing new avenues to analyze mechanisms governing HSC cell growth and fate decisions at the single-cell level [54,56–58].
16.4 HSC Control Ex Vivo: From HSC Expansion to Biomimetic Niches
As outlined above, biomaterial approaches have been developed to explore exogenous cues of the HSC niche ex vivo. In this section, we will illustrate a few examples, showing their ability to mimic relevant features of in vivo stem cell behavior. We begin with early approaches of HSC culture and HSC expansion. Additionally, a more complex setup of adhesion ligands and growth factors presentations as well as coculture systems is discussed.
16.4.1 Ex vivo Expansion
HSCs were one of the first examples showing the regenerative potential of stem cells [59–61]. Within the introduction of BM transplantation by Donnall Thomson, HSCs were used to cure severe conditions in leukemia treatment [62]. Despite this long history of HSC research and therapeutic application, many questions remain in HSC biology and about their clinical use. In particular, it would be highly desirable to transplant a much larger number of HSCs when treating leukemia. At present, an expensive and resource-consuming pooling of several apheresis blood samples is used for collecting sufficient cells for a transplant. One way to overcome this HSC shortage is to use ex vivo expansion strategies or the controlled differentiation of embryonic stem cells toward blood lineages [63,64]. However, these new strategies are awaiting solid evidence for an improved clinical outcome in HSC transplantation [65,66].
Most approaches of ex vivo HSC expansion rely on the knowledge about the tight control of HSC behavior by the local microenvironment of the cells in vivo [1,2,67,68]. As HSCs were long considered to be suspension cells, the main focus has been on soluble cues in their microenvironment, for example, cytokines and oxygen pressure. Consequently, growth factor compositions of the cell culture media in static and perfusion cultures were examined in early expansion strategies [69–72]. To date, various promising growth factor cocktails have been developed and are still being developed on the basis of new screening tools. Besides the growth factors supplemented to the media, cell-released factors were also discovered to be substantial in the expansion of HSCs. A fed-batch approach was developed to control secreted factor concentrations demonstrating an improvement in HSC expansion [73]. Therein, a process of stepwise volume increase of cell culture media was introduced, so-called fed-batch, which dilutes cell-released factors and improves HSC expansion.
16.4.2 Growth Factor Presentation
Apart from identifying and adjusting concentration levels, the precise control over presentation and release of signaling molecules in a temporally controlled and oriented manner has more recently come into focus to improve HSC expansion. Biomaterial surfaces and hydrogel scaffolds were designed with growth factors (e.g., SCF, bone morphogenetic protein 4, and TPO) and cell surface ligands (e.g., Delta-1, Delta-4, and Jagged) being either (non)covalently tethered to the surface or incorporated into the biomaterials. These strategies were, in part, inspired by the function of glycosaminoglycans (e.g., heparan sulfate), which have been found to bind relevant growth factors specifically and present them to HSCs [74,75]. They show promising results in ex vivo
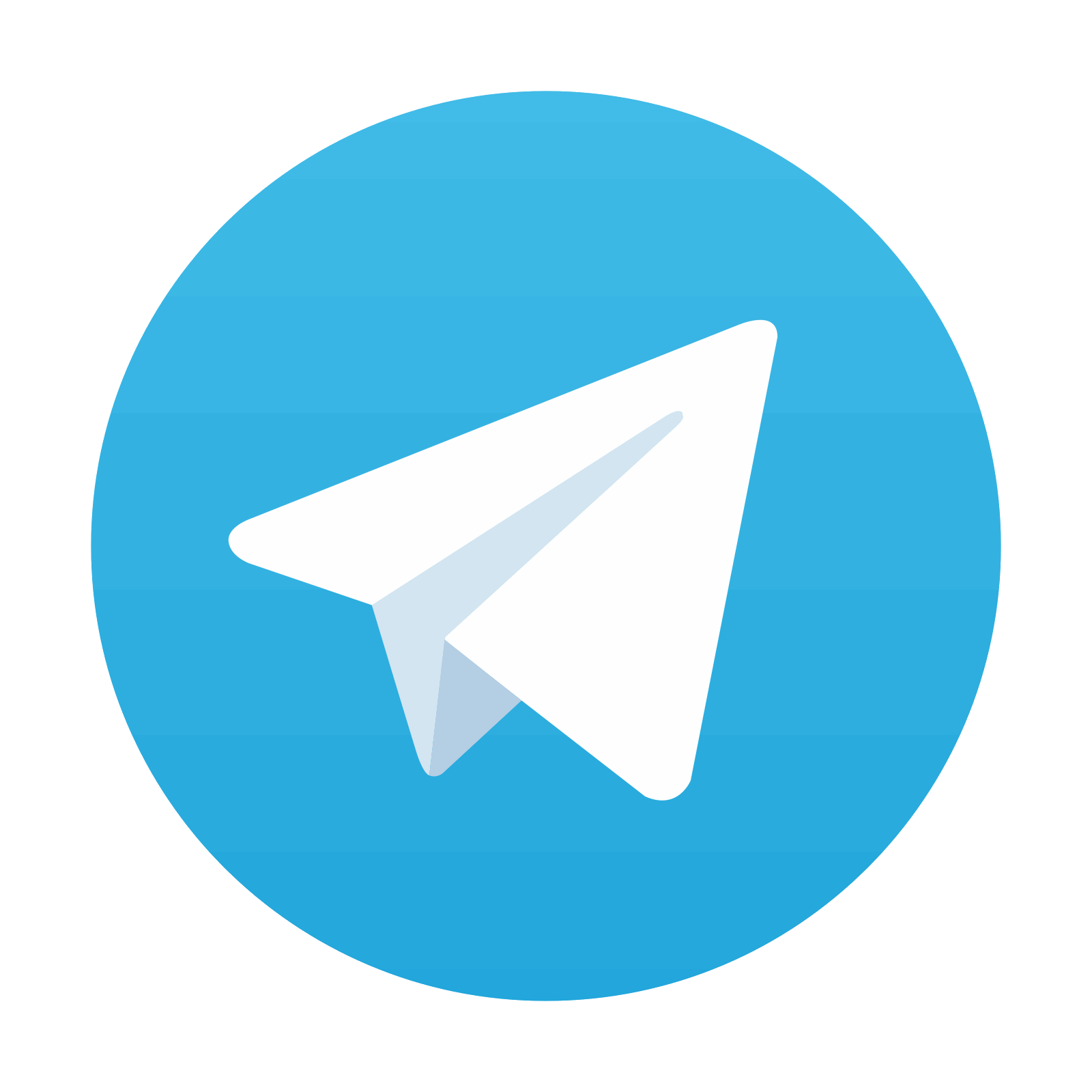
Stay updated, free articles. Join our Telegram channel

Full access? Get Clinical Tree
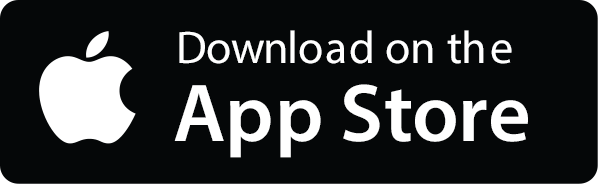
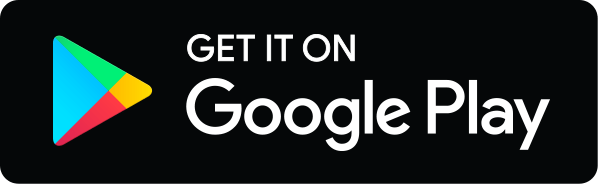