Evaluation of Bio-inspired Materials for Mineralized Tissue Regeneration Using Type I Collagen Reporter Cells
Reconstructive Sciences, University of Connecticut Health Center, Farmington, CT, USA
14.1 Introduction
Materials that enhance bone regeneration have a wealth of potential clinical applications. These applications are causing the market for biomaterials-based treatments in orthopedics to grow at a rapid rate. In the past, materials intended for implantation were designed to be bioinert; however, materials scientists have shifted toward the design of deliberately bioactive materials that integrate with cells or biological molecules and regenerate tissues. It is desirable that these new bioactive materials promote osteogenesis, that is, they should be able to influence and support the cells around them or the cells seeded directly on them. As bio-inspired biomaterials get more sophisticated and are being designed to interact with the biological environment and influence cell behavior, methods are needed to monitor the effects of biomaterials, particularly in a way that gives spatial information that can be related to the structure of the biomaterial. Stem cells are of increasing importance, and bio-inspired materials can influence stem cell behavior, particularly proliferation and differentiation. In the field of mineralized tissue regeneration, there is an emerging technology that greatly simplifies the analysis of osteogenic differentiation known as type I collagen/green fluorescent protein (GFP) reporter technology. In this chapter, we explain the basics of how the technology works, the methodology, and provide examples of use and advantages of this technology when applied to several types of bio-inspired biomaterials and cell therapy.
14.2 Collagen 1 Promoter/GFP Reporter Technology
14.2.1 Reporter Gene Systems
The use of a reporter gene system allows for the ability to track, monitor, and visualize cellular response to a given material or environment. A reporter gene is used to study the activity of a particular gene of interest called the promoter gene (Figure 14.1). A reporter gene system relies on the fusion of a promoter gene to a specific reporter gene and can be used to determine the promoter’s activity under specific conditions or within a particular cell. In more simple terms, the reporter gene is attached to the coding region of the promoter gene within the DNA; when the promoter gene is biologically activated, it synthesizes not only its own regulatory proteins defined by that gene, but it activates the reporter protein expression. Ideally, the reporter gene should cause the production of a protein that is easily identified and monitored such as a protein that does not occur within the normal system of that animal. A protein that is widely used for this purpose is GFP that is normal in jellyfish, but absent in mammals. The expression of the GFP allows scientists to detect when and where the promoter gene is active in the genome. The reporter system allows for the study of the promoter gene at various developmental stages, or allows visualization of when the promoter gene is regulated by an external stimulation.

Shimomura et al. discovered GFP in the jellyfish Aequorea victoria in 1962 [1]. GFP is a protein that exhibits bright green fluorescence when exposed to light in the blue to ultraviolet range. Since then, the GFP gene has been widely used as a reporter to study transcriptional activities within a broad range of hosts. If the cells are expressing GFP, the researcher knows that his or her promoter gene of interest also successfully made it into the reporter system and is being expressed. This is because GFP will be expressed only if the promoter is functionally active. GFP as a reporter can be used in vitro through the gene transfection or transduction process, or it can be used in vivo in transgenic animals. There are numerous benefits associated with the use of GFP as a reporter; however, the main benefits are the ability to visually monitor the promoter gene’s activity, the broad host applicability, and the absence of cell lysis or necessary substrate addition that are common with other reporter genes [2].
Bioactive materials that enhance bone regeneration interact with and positively effect bone progenitor cells, also known as bone marrow stromal cells or mesenchymal stem cells (MSCs). In the field of orthopedic research, it is of particular interest to know if implanted biomaterials influence the osteogenic differentiation of progenitor cells. To track osteogenic differentiation of bone progenitor cells, there are several marker genes associated with osteogenesis, and each marker’s promoter gene can be used to drive a reporter for event-specific imaging. Osteocalcin, type I collagen (Col1a1), and alkaline phosphatase (ALP) are important markers associated with matrix maturation at different stages of progenitor differentiation into the bone tissue [3]. Transgenic mice with GFP expression attached to a variety of promoter genes associated with musculoskeletal regeneration have been produced by Dr. David Rowe’s lab of the University of Connecticut Health Center [4]. As seen in Figure 14.2, the bone progenitor cells within the mouse bones fluoresce depending on their level of differentiation, thereby allowing researchers to monitor the progression of bone progenitor cells as they differentiate into mature bone cells. This chapter will focus on the use of the Col1a1 promoter to drive GFP expression to identify various stages of osteogenic cell differentiation on biomimetic biomaterials.

14.2.2 How to Make a Reporter Gene System
14.2.2.1 Retrovirus
There are two general categories of gene delivery systems: viral and nonviral. The majority of gene delivery systems use virus vectors. The reason why viral delivery is most commonly used is because viruses have evolved to be very efficient at replication and survival. One of the most crucial aspects of a virus is its ability to transport its genomic DNA to the nucleus of the host cells without degradation by lysosomes. Nonviral gene delivery systems include, for example, direct DNA delivery, liposomes, and DNA–protein complexes. The advantage of these nonviral systems is that they can be easily prepared in a uniform fashion and can be made in larger quantities; however, when compared to viral vector delivery systems, there is a reduced efficacy of expression [5]. Here we will focus on viral vectors, specifically retroviral vectors.
Retroviruses are enveloped RNA viruses that are members of the family Retroviridae, and they are widely distributed in various vertebrate species. These types of viruses are utilized because they contain the enzyme reverse transcriptase. Reverse transcriptase is essential for the conversion of single-strand RNA to double-strand DNA in the process of forming a provirus that is integrated into cellular DNA. Retrovirus vectors also integrate into the chromosomes of the host cells; therefore, they provide an opportunity for prolonged gene expression [5]. An essential step in the development of a virus vector is the creation of a recombinant virus that is capable of infecting a cell but is replication-defective. For retroviruses, this has been accomplished by deleting all the viral structural protein genes and inserting either a therapeutic gene or a marker gene (often called the transgene) [5]. Many retrovirus vectors are introduced in vitro into autologous stem cells, and then the cells are transplanted directly into the host for analysis in situ. It is expected that some of the transplanted cells will remain in an undifferentiated state and will act as a permanent reservoir of gene-corrected cells [6]. When the appropriate signals are received, the promoter gene will be activated and will cause expression of the reporter gene.
14.2.2.2 The Process of Creating Transgenic Mice
Genetically manipulated mouse models make it possible to study gene functions in whole animals. Traditional gene knockout mice that represent a loss-of-function strategy have been widely used in research for many years. The transgenic mice discussed here represent a newer concept with a gain-of-function approach to define molecular and cellular functions of a gene of interest. This approach can be used to analyze tissue-specific or developmental stage-specific gene expression by introducing reporter genes, (such as GFP as previously discussed), under the control of a specific gene promoter. If the functional domain of a gene of interest is well characterized, transgenic mouse strains can be created [7].
Transgenic mice are most commonly generated by microinjecting the transgenic construct into the male pronuclei of a fertilized mouse egg. This method has five basic steps: (a) purifying the transgenic construct, (b) harvesting the donor eggs, (c) microinjecting the transgenic construct into the male pronuclei of the egg, (d) implanting the microinjected eggs into a surrogate female mouse, and (e) genotyping and analyzing the transgene expression in the offspring [8]. Usually, dark coated males and light coated females are selected to produce the initial fertilized egg because the researcher will be able to visualize which offspring contains the transgene; remember, the transgene is microinjected into the male pronuclei, therefore, mice with dark spots on their coats will contain the construct. The offspring of the surrogate are referred to as progeny or chimeric mice and will contain genetic material with and without the transgene and their coats will appear spotted (Figure 14.3 [9], mother). The progeny that screen positive for containing the transgene will be selected to mate. The screening is done by removing a small piece of tissue from the tail and examining its DNA for the desired gene, or by simply looking for GFP expression if GFP is the reporter and expressed in tissues near the skin surface. Mice that have the gene will be heterozygous (spotted) and are mated with wild-type mice. One out of four pups of the new litter will be homozygous for the transgene (will have a completely dark coat) (Figure 14.3, pups), and subsequent crosses (matings) of this mouse will produce the transgenic strain. Other ways to introduce the transgene into an egg is the use of a retrovirus vector as previously discussed, or to transfect a transgenic construct into mouse embryonic stem cells (ESCs). These ESCs can then be injected into mouse blastocysts. This method is useful to obtain a low copy number of the transgene in the mice, and it is also useful when embryonic death is expected in the resultant transgenic mice [7]. With both the retroviral and embryonic stem cell method, once the embryo has been implanted into a surrogate, the steps for producing the transgenic line are the same with the microinjection method.

Kalajzic et al. have developed transgenic mice by the use of a transgenic construct, in which GFP expression is under the control of the 3.6- and 2.3-kb Col1a1 promoter fragments [4]. GFP-driven 2.3-kb Col1a1 promoter fragment of the type I collagen gene (referred to as 2.3Col/GFP) is expressed in mature osteoblasts, harvested from the bony tissue of transgenic mice pups as described below, at the onset of mineralization. 3.6-kb Col1a1 promoter fragment driving a cyan variant of GFP (3.6Col/GFP) is expressed in preosteoblast cultures 5–7 days before the colonies develop into mineralizing multilayered nodules. The use of multiple promoters and fluorescent isomers of GFP makes it possible to monitor developmental stage-specific differentiation of the osteoblast lineage. Distinctly, different populations of cells within the osteoblastic lineage can be recognized—3.6Col/GFP indicating early osteoblast progenitors and 2.3Col/GFP indicating mature differentiated osteoblasts [4]. As a result of these transgenic mice, various stages of osteoblastic differentiation in cell culture and in intact bone can be assessed when evaluating novel, bioactive materials. This chapter is focused on describing the application of this technology to evaluate osteogenic differentiation.
14.3 Primary Cell Harvest and Image Analysis of the Collagen Reporter Cells from Transgenic Mice
14.3.1 Harvesting Primary Osteoprogenitor Cells From Transgenic Mice Calvarium
In order to conduct in vitro studies with primary type I collagen/GFP reporter primary osteoblast cells, the cells must first be harvested from the bony tissues of transgenic mouse pups. Osteoprogenitor cells can be harvested from the bone marrow or the bones. The use of young mice greatly increases the yield of the progenitor cells, which are typically harvested from the calvarial bones. To obtain the cells from the calvaria, pups that are 3–6 days old are sacrificed with carbon dioxide. In a sterile environment, the euthanized pups are cleaned with 70% ethanol. The calvarial bones are gently cut out from the skull and removed as one piece, including both parietal bones and the sutures. Nonbony tissue is carefully scraped away and the sutures are then cut away and discarded. To release the cells from the bony matrix, the matrix and other tissues associated with the calvaria must be digested enzymatically using an enzyme solution prepared from a combination of collagenase I, phosphate buffered solution (PBS), and trypsin. Multiple digestions are performed to extract the cells. The first extract is discarded but the second through fifth solutions are kept. The digestion solution is added to equal parts medium with 10% fetal bovine serum to stop the enzymatic reaction and is stored on ice. Once the digestion steps are completed, the resulting cell solution is centrifuged and the cell pellet is resuspended in proliferative medium. Cells are counted and plated typically at 15,000 cells/cm2. That is a suitable density to achieve confluency by day 7 and robust mineralization over the 21-day mineralization assay [10]. If the biomaterial degrades rapidly in culture and is intended for short-term use only, the 3.6Col/GFP reporter system is preferred since the readout occurs earlier in the culture.
14.3.2 In Vitro Imaging and Analysis
Nondestructive fluorescence imaging can be utilized to view type I collagen/GFP-expressing cells during experiments without having to terminate plates. Not only can osteogenic cells be viewed, but the use of a fluorescent calcium chelating dye that binds to CaP mineral crystals, allows one to visualize the mineral associated with the GFP positive cells and estimate the calcium content in living cell cultures. One of the most commonly used dyes for assessing mineralization nondestructively is xylenol orange (XO). Calcium deposition or mineralization is one of several features that characterize bone formation or osteoblastic function/differentiation. In some cases, calcium deposition may be unrelated to osteoblast activity if extensive cell death occurs in the cell cultures, or if high amounts of osteogenic medium components are used that lead to artifactual precipitation. Distinguishing between calcium deposition associated with osteoblast-produced mineral and that from pathological or artifactual deposition requires additional structural and chemical characterization of the mineralized matrix and biological characterization of the cell. The use of the type I collagen/GFP reporter cells facilitates distinguishing mineral produced by osteoblasts. Using fluorescence imaging, one can determine if the stained mineralized nodules correspond, or overlap with, GFP expressing mature osteoblast cells. An example of colocalization of the GFP and XO is demonstrated in Figure 14.4. The stained mineralized matrix (red) overlaps with the green fluorescence representing osteoblast cells indicating that the GFP+ cells have formed the mineralized matrix. Using this reporter technology, one can also observe the increase in cell GFP expression with time without needing to terminate the cultures or apply any dyes or antibodies to the cultures. This is one key benefit of the technology.

In addition to visualizing cells and mineral deposition, using image analysis software allows the researcher to determine quantitative results such as percent GFP/XO-positive area, GFP/XO intensity, and calcium content. These values can be normalized to cell number by measuring total DNA content; however, DNA content is a destructive assay, therefore, it will require additional control plates. The imaging and analysis practice involves (a) collecting fluorescent microscopy images of the cells and (b) conducting image analysis of thresholded images of the standards and the samples to determine the area percentage and the mean intensity of the fluorescent areas. The use of a standard allows for the comparison between different samples and/or different time points.
To perform this type of imaging and analysis, the following equipment must be available:
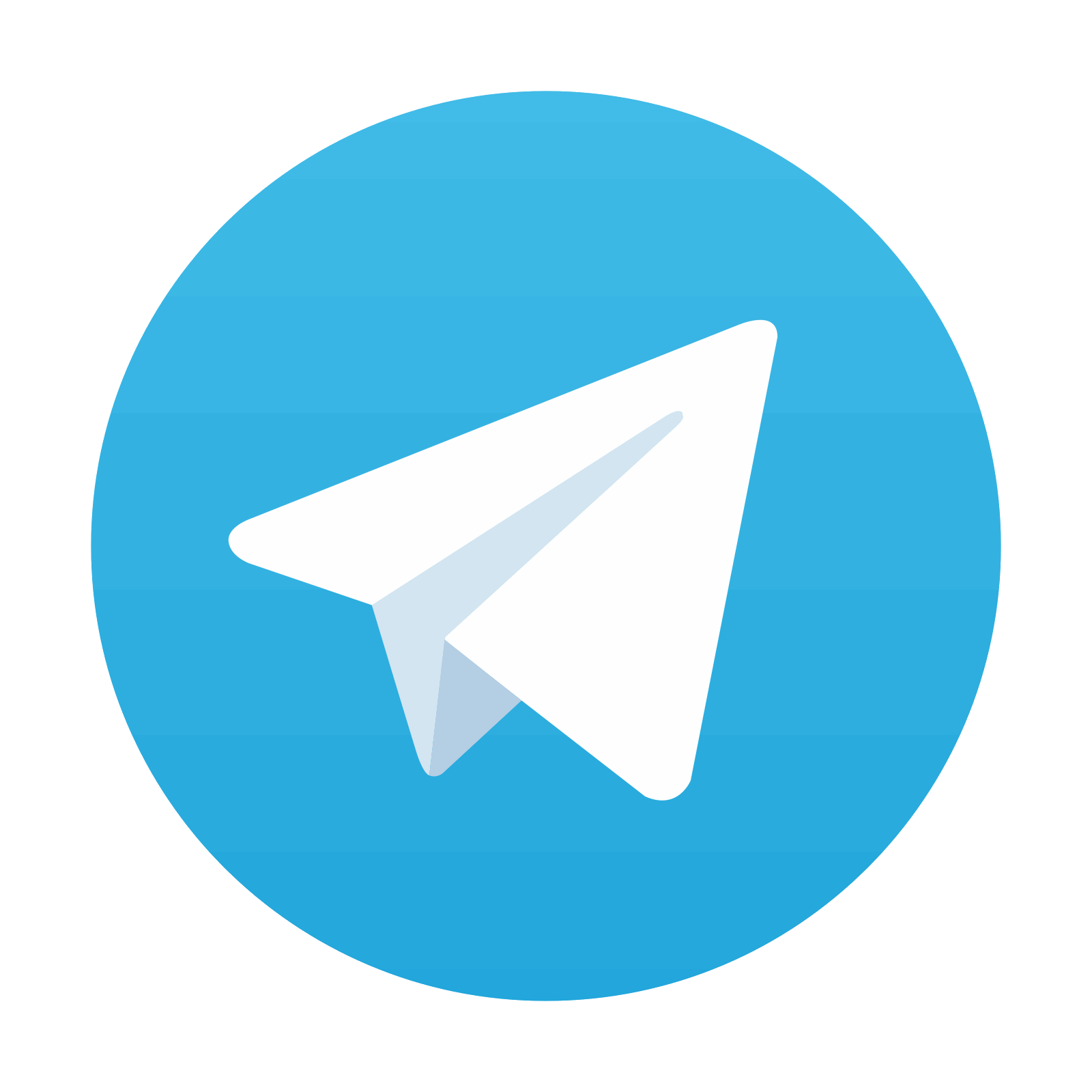
Stay updated, free articles. Join our Telegram channel

Full access? Get Clinical Tree
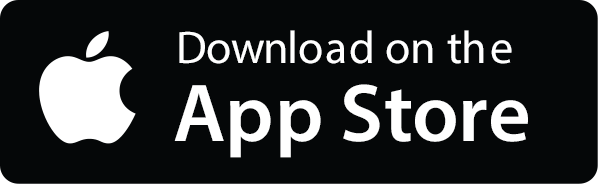
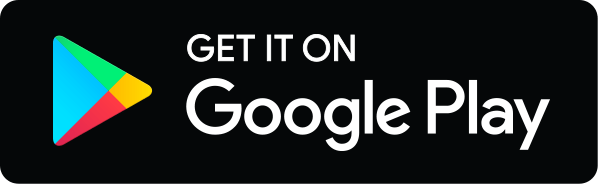