CHAPTER 13 Stephen A. Moser and Jason Wicker University of Alabama at Birmingham, Birmingham, AL, USA Rapid diagnosis of fungal pathogens is crucial for immunocompromised patients, for whom opportunistic infections can often be fatal. The advance of human immunodeficiency virus (HIV) disease, the widespread use of antibiotics, and the growing number of transplants performed each year all contribute to the increased incidence of fungal infections [17]. Most currently available commercial systems are relatively rapid (2–72 h) and widely used; however, in many cases, these methods still lack sensitivity and require substantial laboratory expertise to interpret supplementary tests, identify microscopic features of organisms, or correctly choose isolates for appropriate testing. Clearly, more efforts will be necessary to advance more rapid and sensitive test methods to diagnose fungal disease. The methods described in this chapter are meant to represent those that are commercially available, US Food and Drug Administration (FDA) approved, and published in peer-reviewed journals. Some newer tests yet to be approved by the FDA are included since it is clear that this approval is forthcoming (e.g., MALDI-ToF/MS [matrix-assisted laser desorption ionization with time-of-flight mass spectrometry]). Interest in commercially available solutions for accurate, more rapid and less costly diagnosis has forced a change in the algorithms used for fungal diagnosis and increased the numbers of published comparisons and evaluations of commercial methods and systems. Certainly, publications of this nature will continue to appear at a relatively rapid pace, as the healthcare and clinical-diagnostics industries strive to improve patient care and reduce costs. Accurate and sensitive detection methods for fungal pathogens in clinical specimens are essential for a number of reasons. Many patients infected with fungi are immunosuppressed, and disease in these patients can be severe and progress rapidly. Results from fungal cultures and serological testing often come too late to affect patient management. Direct microscopic examination has traditionally been performed by analysis of KOH preparations with and without Calcofluor white staining, but smears may lack sensitivity [140]. Despite these limitations, the direct recognition of fungi in clinical specimens remains the most rapid means of fungal diagnosis. Unfortunately, only a few pathogens have commercial products specifically designed to enhance their direct detection in clinical specimens. Most available methods target fungal cell-wall antigens or fungal enzymes. There are two species of pathogenic Cryptococci: Cryptococcus neoformans – which has two varieties C. neoformans var. neoformans (serotype D) and var. grubii (serotype A) – and Cryptococcus gattii (serotype B and C) – formerly considered a variety of C. neoformans but has been assigned as a separate species in the genus Cryptococcus (Table 13.1). Using molecular typing C. neoformans var. grubii has been found to be the most common worldwide etiologic agent of opportunistic cryptococcosis in HIV-AIDS infected individuals. Cryptococcus neoformans var neoformans is most common in Europe and C. gattii, originally described in Australia, has migrated to the Pacific Northwest United States and British Columbia and is increasingly reported from many countries and continents [285]. For the remaining discussion in this chapter, the Cryptococcus complex (neoformans var neoformans and var grubii and gattii) will be referred to as C. neoformans. Table 13.1 Two varieties of Cryptococcus neoformans and the second pathogenic species, C. gattii Source: adapted from reference 260. CGB, canavanine–glycine–bromothymol blue medium. Due to its high specificity and increased sensitivity, the Cryptococcus antigen detection tests have, for the most part, replaced the more historic India ink test and have become the “gold standard” for direct Cryptococcus testing [154, 214]. The cryptococcal glucuronoxylomannan (GXM) capsular antigen can be detected via latex agglutination (LA), enzyme immunoassay (EIA), or lateral-flow immunoassay (LFA). Due to the soluble nature of the cryptococcal antigen, most methods can detect antigen in serum or cerebrospinal fluid (CSF) even before viable organisms can be cultivated. Different cryptococcal serotypes (A, B, C, D) are known to be cross-reactive [203], and are detected by these assays. However, one study comparing the sensitivity of the LFA, LA, and EIA assays shows that the LFA is the most sensitive, with less sensitivity by other assays and a wide range of sensitivity for serotype C (Table 13.2; 232]. Table 13.2 CrAg method sensitivity [232] The amount of antigen detected is generally accepted to correlate with disease severity; however, titers may persist for some time after therapy is completed, especially for AIDS patients, whose titers may decline slowly even after clinical improvement has occurred. Results may be difficult to interpret when patients are immunocompromised, since some patients will never clear the antigen. Titers can be helpful for monitoring disease, but care must be taken to compare titers only when and if the specimens have been tested with the same test methods or kits during the same batch testing. For titers to have diagnostic meaning, there must be careful adherence to protocols, and samples must be collected and frozen over time for simultaneous batch testing. Latex agglutination methods were the first tests developed and provide an immunochemical technique for detecting cryptococcal antigen in serum or CSF. Available kits include: Figure 13.1 Cryptococcal Antigen Latex Agglutination System. (Courtesy of Meridian Bioscience, Inc.) Most test kits contain latex particles sensitized with anticryptococcal immunoglobulins. Normal rabbit immunoglobulins are used as the control to test for nonspecific latex agglutination. When the anticryptococcal immunoglobulin-sensitized latex particle comes in contact with the polysaccharide antigen of C. neoformans in the patient’s specimen it forms a complex that results in visible agglutination. The reaction occurs on a slide included in the kit and the test is read by observation. There are both qualitative and semiquantitative protocols for this assay. In the semiquantitative procedure, the titer is the reciprocal of the last dilution to demonstrate agglutination [293]. Despite the sensitive and accurate nature of cryptococcal antigen testing, there are a few pitfalls related to the use of latex test kits. As with any assay, low concentrations of antigen can cause false-negative reactions to occur. False-negative reactions can also be caused by very high antigen concentrations that cause a prozone effect or evident in cases of immune complex formation. The latter can be avoided by the dissociation of such complexes with pronase pretreatment of sera [121, 289]. In addition, false negatives have also been reported in specimens from patients infected with the nonencapsulated variants of C. neoformans [154]. Alternatively, false-positive reactions can be caused by the presence of Trichosporon beigelii [190], Stomatococcus mucilaginosus [45], Capnocytophaga canimorsus [314], and the use of BBL Port-a-Cul anaerobic transport device [319]. Rheumatoid factor may cause nonspecific agglutination [80, 140] as will the presence of some soaps and disinfectants [25], povidone-iodine from skin preparations [62], and syneresis fluid [136]. The Premier Cryptococcal Antigen EIA kit (Figure 13.2; Meridian Bioscience, Inc.) uses anticryptococcal polyclonal antibodies adsorbed to plastic microwells, which can be used for batch assays or snapped off and used for smaller sample runs. The detection system is based on a sandwich EIA using a monoclonal peroxidase conjugate. If cryptococcal antigen is present in the sample, a complex is formed between the adsorbed antibody, the antigen, and the enzyme conjugate. After the wells are washed to remove unbound conjugate, a substrate solution is added, and color will develop in the presence of the bound enzyme and in proportion to the amount of cryptococcal antigen present. With a spectrophotometer, semiquantitative results can be generated, producing titers that can be used to monitor drug therapy or disease severity. Prozone effects, like those that interfere with latex-based testing, are generally not a problem. Cross-reactions can occur, causing false-positive reactions with the yeast T. beigelii [190] and with syneresis fluid [136]. Test endpoints are objective but are more costly to achieve than endpoint titers generated by the latex-based methods [79, 136]. Figure 13.2 Enzyme linked immunoassay for cryptococcal antigen. (Courtesy of Meridian Bioscience, Inc.) The ALPHA Cryptococcal Antigen enzyme immunoassay (CrAg EIA; Immuno-Mycologics, Inc.) is a qualitative or semiquantitative test system for the detection of capsular polysaccharide antigens of C. neoformans in serum and cerebrospinal fluid (CSF). It is a direct immunoenzymatic sandwich microplate assay. The CrAg Lateral Flow Assay (LFA; Immuno-Mycologics, Inc.) is a qualitative or semiquantitative dipstick sandwich immunochromatographic assay, which can be used as a point of care test. It has recently been cleared by the FDA for testing serum and CSF (Figure 13.3). It incorporates two monoclonal antibodies against GXM to cover all serotypes of C. neoformans [115, 232]. The LFA reagents are stable at room temperature, have a 2-year shelf life, a rapid turnaround time, and can be performed with minimal laboratory support. The test is performed by adding 40 μL of serum or CSF to a specimen diluent glycine buffered saline with blocking agent in a microfuge tube or microtiter well, the LFA strip is added and read after incubation at room temperature for 10 min. No pretreatment with pronase is required. Figure 13.3 Cryptococcal antigen Lateral Flow Assay. (Courtesy of IMMY.) In a comparison of the Premier EIA with the CALAS latex agglutination assays, 475 specimens were screened, including 120 positive specimens from serum and CSF. Serum samples that contained rheumatoid factor were tested to determine which methods would be affected and thus generate false-positive results. When the results were compared, the EIA was found to be virtually equivalent to the latex method, with 99% sensitivity and 97% specificity [111]. Another report [105] compared the Premier EIA and the CALAS latex agglutination tests ability to detect cryptococcal capsular antigen in serum (n = 471, with 103 positives) and CSF (n = 123, with 18 positives). The methods were evaluated for the dual purposes of sample screening and quantitation. Good concordance (97.8%) was reported between the tests. However, 12 serum samples and one CSF sample had discrepant screening results. When used for semiquantitative assays for titer determination, the titers obtained with Cryptococcus antigen assays performed by EIA were generally higher than those obtained with latex [105]. The Premier EIA kit was compared to several latex agglutination kits by using CSF and serum specimens. Of the 182 CSF specimens, 19 had positive cultures, and of the 90 serum specimens, 30 cultures were positive. The latex agglutination kits used for comparison were CALAS and IMMY. For CSF testing, the sensitivities for all of the kits were comparable, ranging from 93 to 100%. The specificities were also comparable ranging from 93 to 98%. The specificities of the kits for serum testing were similar, ranging from 97 to 100%; both IMMY and Premier EIA had a sensitivity of 97% [293]. Pronase treatment is known to be beneficial for enhancing the sensitivities of polyclonal antibody-based latex agglutination methods [121, 131, 289]. While it was beneficial in reducing false-positive serum test results, studies have shown that pronase pretreatment of CSF is not efficacious, and it is not recommended [131]. A study performed in Cape Town, South Africa involved 62 patients with laboratory confirmed HIV-associated cryptococcal meningoencephalitis. Serum, plasma, and urine were tested with the CrAg LFA and an enzyme-linked immunosorbent assay (ELISA) using the same monoclonal antibodies used in the LFA test. All patients had GXM detected in all specimen types by the ELISA and 61/62 (one urine negative) by LFA with a sensitivity of 100% for serum and plasma and 98% for urine [147]. A prospective study performed in Sidney, Australia evaluated 92 patient’s serum, CSF, and urine when available, by both CALAS and the CrAg LFA. Twenty-five of the patients were diagnosed with cryptococcosis either by culture (20), histology (three) or molecular methods (two). They found that the LFA and CALAS were equivalent when used qualitatively but suggest that further study is required to correlate titer between latex agglutination and the lateral flow assay [191]. In a study of archived sera from HIV-infected patients the LFA was compared to Premier EIA positive specimens with a 90% agreement at a 5 min reading and 96% agreement when read at 15 min [173]. Two EIA negative specimens were positive by LFA. Seventy percent of urines from EIA serum positive patients were positive. The FDA-approved LFA kit is currently cleared for serum and cerebrospinal fluid and not urine. The presence of an unidentified heat-labile Candida antigen can be detected in serum by the Cand-Tek Latex Agglutination test (Ramco Laboratories, Stafford, TX). In an early study of 911 samples, the reproducibility of titer results was reported to be 90% (exact titer) to 100% (±1 dilution) [40], but the sensitivity for detection of disseminated candidiasis was limited depending on titer used to define a positive (46–95%). The specificity for disseminated candidiasis ranged from 22 to 99% [40, 137, 218]. The Cand-Tek method may be best used as a measure of the severity of disease, since it has been suggested that the antigens detected by this assay accumulate in sera from patients with renal failure. In a study [32] that measured creatinine levels of 22 patients with proven disseminated candidiasis, the Cand-Tek assay yielded positive results for a significantly larger number of patients having disseminated candidiasis than those with simple local colonization. In addition, serum creatinine levels exhibited an increase parallel with that of Cand-Tek titers. Despite this association, results based on disease prevalence and a positive reaction cutoff of a 1:8 titer showed that a positive Cand-Tek assay result had only a 79% probability of being associated with disseminated candidiasis. In an analysis of the Platelia Candida Ag Plus test (Bio-Rad, France (formally Pastorex); not currently available in United States) with the Cand-Tek antigenemia test, the specificities were 100 and 98.3%, respectively. The overall sensitivities of both tests were low, but with multiple testing, a positive antigen test result preceded other indications of candidemia for six of ten Platelia-positive patients and five of nine Cand-Tek positive patients. Multiple sampling increased the sensitivity of the Platelia Candida test to a high of 76.9%; however, this was not the case with the Cand-Tek assay [137]. The Cand-Tek test was compared to methods for the detection of enolase, mannan antigen (Pastorex Candida assay) and to the detection of β-glucan by the Limulus test. In 39 patients with candidemia, the sensitivity of the assays was 76.9%, 25.6%, 84.4%, and 71.2% for enolase, Cand-Tek, Pastorex Candida, and β-glucan assays respectively. Of 10 patients with superficial Candida colonization, 10 patients with deep infections, and 20 healthy subjects, it was found that two patients with superficial infections and one patient with invasive pulmonary aspergillosis were also positive with the Cand-Tek assay. None of the tests in this study appeared satisfactory for the initial diagnosis of candidemia; however, the tests may be helpful in certain diagnostic situations [204]. A meta-analysis of 14 studies of mannan antigenemia determined by the Platelia Candida assay comprised of 453 patients and 767 controls showed an overall sensitivity of 62% (range 31–100%) and specificity of 93% (range 65–100%) [200]. These studies included patients with hematological malignancy and from intensive care units (ICU) that were classified by the 2008 European Organization for Research and Treatment of Cancer-Mycoses Study Group (EORTC-MSG) criteria [65]. Invasive aspergillosis is a serious problem for all immunocompromised patients, especially those undergoing bone marrow transplantation or chemotherapy. The disease is severe, progresses rapidly, and is difficult to diagnose and treat. Fatality rates can approach 100% but can be reduced with early diagnosis and proper therapy. Unfortunately, blood and respiratory cultures may be negative during the early stages of disease [138]. The Bio-Rad Platelia Aspergillus EIA (Bio-Rad Laboratories, Redmond, WA) is a one-stage immunoenzymatic sandwich assay that uses the rat monoclonal antibody EBA-2 to detect circulating galactomannan antigen in human serum and bronchoalveolar lavage fluid (Figure 13.4). The galactomannan antigen is released in the circulation of patients or respiratory fluid during active invasive aspergillosis. Results are expressed in index values and can be obtained in 3 h. This method is now integrated into the EORTC-MSG criteria of definition and classification of invasive aspergillosis [303]. Platelia Aspergillus EIA allows the diagnosis of invasive aspergillosis at an early stage of the disease, often before the onset of clinical signs [182]. Figure 13.4 Platelia Aspergillus Antigen assay kit. (Courtesy of Bio-Rad Laboratories, Inc.) A modified pretreatment technique (MT) was used on consecutive negative Platelia Aspergillus EIA plasma samples from invasive Aspergillus patients in order to improve the sensitivity of the assay [195]. Fifty-two plasma samples were collected from healthy donors, and 174 plasma samples with a galactomannan index (GMI) below 0.5 were collected from 25 unclassifiable and 23 patients with invasive aspergillosis. The reactivity of pretreated samples was determined before (conventional technique [CT]) and after filtration concentration by using a filter with a 50-kDa cutoff (MT). For the MT, the sensitivity of the Platelia Aspergillus EIA increased from 42.9% (CT) to 78.6% (MT) using a cutoff for the GMI of 1.5 in the probable and proven group, whereas specificity slightly decreased from 98.7 to 96.1% in the control group. The tenfold concentration step increased the GMI as high as 121-fold. The MT resulted not only in positive reactivity in samples that tested negative with the CT but also in the earlier detection of antigen by 2–17 days [195]. Soon after the introduction of the Platelia Aspergillus assay it was noted that cross-reactions occurred with patients on antibiotic therapy. The initial association was with piperacillin-tazobactam but was expanded to amoxicillin-clavulanate, cefepime and cefoperazone-sulbactam in both serum and bronchial alveolar lavage (BAL) specimens [26]. Nine of 11 hematological patients with disseminated Fusarium spp. infection tested at least twice for Aspergillus galactomannan (GM) had repeated positive results in the absence of Aspergillus isolation in culture. The centrifuged supernatants of 12 Fusarium isolates were tested by a GM enzyme-linked immunosorbent assay (EIA). All the isolates produced positive reactions when tested undiluted. These results show cross-reactivity of Fusarium spp. with Aspergillus GM that may constitute a drawback with respect to the specificity of the Platelia EIA [300]. In another study [323]cross-reactivity in the Platelia Aspergillus enzyme immunoassay was evaluated using 120 sera from patients with paracoccidioidomycosis, histoplasmosis, or cryptococcosis. At a cutoff value of 0.5, positivity rates were 50%, 67%, and 50%, respectively. An evaluation of HIV-infected patients in Taiwan found that Penicillium marneffei cross-reacts in the GM assay of patients with penicilliosis, cryptococcosis, and controls, 73.3%, 13.6%, and 9%, respectively (P = 0.0001). The use of the GM antigen assay may facilitate earlier diagnosis of P. marneffei infection for HIV-infected patients in areas of endemicity [141]. Twenty-three of 48 serum specimens positive for antigen in the second-generation Histoplasma antigen EIA (MiraVista Diagnostics, Indianapolis, IN) were positive in the Platelia Aspergillus EIA. Seven of 11 BAL fluid specimens that were positive in the Histoplasma antigen EIA were positive in the Platelia Aspergillus EIA. Ten control BAL fluid specimens that were negative in the Histoplasma antigen EIA were negative in the Platelia Aspergillus EIA. Care must be taken to exclude histoplasmosis for patients with positive Platelia Aspergillus EIA results [315]. The Fungitell assay (Associates of Cape Cod, East Falmouth, MA) is based on a modification of the Limulus Amebocyte Lysate assay. The Fungitell reagent is modified to eliminate Factor C (specific for endotoxin) and only react to 1,3-β-D-glucan (BDG) through the Factor G mediated side of the pathway. The reaction uses a protease zymogen-based colorimetric assay for the qualitative detection of BDG in the serum of patients with symptoms of, or medical conditions predisposing the patient to, invasive fungal infection. The serum concentration of BDG, a major cell-wall component of various medically important fungi can be used as an aid in the diagnosis of disseminated mycoses and fungemia such as Candida and Aspergillus [65, 215]. A positive result does not indicate which class of fungi may be causing infection. In addition the Fungitell assay does not detect certain fungal species such as the genus Cryptococcus, which produces low levels of BDG [205], and Zygomycetes [221], which are not known to produce BDG. The yeast phase of Blastomyces dermatitidis produces little BDG and may not be detected by the assay [118]. Difficulties have been reported in interpreting the results of this assay, since plasma glucan activity was found to be increased in hemodialysis patients [151, 153], and subjects who were undergoing chemotherapy for hematologic malignancies but who had no signs of invasive fungal disease [150]. Other false-positive reactions have also been observed in patients after surgery, perhaps due to the presence of BDG in surgical gauze or cellulose dialyzer tubing [216, 259]. A report investigating the interaction between intravenous ampicillin-sulbactam treatment and BDG assay did not observe any significant association of ampicillin-sulbactam administration and positive assays for BDG [199]. Fifteen patients without known risk factors for invasive fungal infections who received a daily dose of ampicillin-sulbactam monotherapy from different batches were included in the study. Thirteen patients had soft tissue infections; five of 13 patients who underwent surgery had surgical dressings. Serum samples were obtained both before and after antibiotic infusion on the first, third, seventh and tenth days of an ampicillin-sulbactam treatment course. The BDG was assayed using the Fungitell kit. A total of 37 of 117 serum samples were positive for BDG at a threshold of 80 pg/mL. After ruling out fungal infections and all known potential causes of false BDG positivity, environmental contamination remained a possible cause of BDG reactivity. False-positive reactions can also occur with patients receiving certain lots of pipericillin-tazobactam or unknown factors [198]. As with many other laboratory tests it is important to apply antigen testing to patient populations with a high probability of invasive fungal infection. Odabasi et al. evaluated 283 patients with acute myelogenous leukemia or myelodysplastic syndrome receiving antifungal prophylaxis by obtaining serial blood specimens for BDG testing. They report a median of 10 days for a positive BDG prior to diagnosis of an invasive fungal infection with a 100% negative predictive value and 90% specificity for a single positive test and 96% for two or more successive positive tests [220]. Onishi et al. in 2012 published a meta-analysis of the utility of the BDG assay in the diagnosis of Pneumocystis jirovecii pneumonia (PJP) and invasive fungal infections (IFI) by Candida spp. and Aspergillus spp. This is an extensive analysis that included 36 studies from many different countries (Japan > United States > others) with both HIV positive and negative patients. The majority of studies were on patients with a hematologic underlining disease. They found that the diagnostic accuracy is high for PJP and moderate for IFI. The sensitivity and specificity for PJP was 96% (confidence interval (CI) 92–98%) and 84% (CI 83–86%) and 80% (CI 77–82%) and 82% (CI 81–83%) for IFI [224]. Comparative studies of the direct stain method to molecular assays are addressed below in the section on molecular diagnostics. Antigen detection tests can be used as part of a diagnostic approach for histoplasmosis, blastomycosis, and coccidioidomycosis. There are three proprietary tests, two offered by reference laboratories: MiraVista Diagnostics (Indianapolis, IN) and ARUP (Salt Lake City, UT) [47]. ARUP developed their assay using ASRs from Immuno-Mycologics, Inc. The reference laboratories have validated the assays in their respective laboratories. The only currently FDA-approved test is the ALPHA Histoplasma EIA test (Immuno-Mycologics, Inc.). The ALPHA Histoplasma EIA test is a sandwich microplate assay which detects Histoplasma antigens in urine. Polyclonal rabbit anti-Histoplasma IgG antibodies are bound to microwell plates and capture antigen, which is detected after washing by biotinylated rabbit anti-Histoplasma IgG antibodies. As with all fungal antigen assays there is significant cross-reactivity. Antigens from B. dermatitidis, C. immitis, and P. brasiliensis were detected while antigens from Aspergillus spp., Candida albicans, and C. neoformans did not cross-react. Many reports in the literature demonstrate the utility of antigen detection in specific patient populations and in cases where clinical suspicion of an infection by one of the systemic mycoses is high. However, there is significant cross-reactivity of each of the assays to the others and to other fungal organisms [7, 73, 165]. The authors of one recent publication regarding the specificity of the MiraVista reference test state that it is not possible to differentiate between histoplasmosis and blastomycosis by antigen detection alone [56]. Increases in cases of fungemia have resulted from greater numbers of immunocompromised patients, the widespread use of invasive medical devices, and the use of broad-spectrum antibiotics. Mortality rates range from 38 to 100%, and early diagnosis is critical. While Candida spp. account for a large percentage of isolates, the range of different fungal species isolated is extensive [117]. Many publications have compared fungemia detection systems prior to the 1990s, and the reader may refer to reviews of those studies elsewhere [117]. Clearly, no system is 100% sensitive or amenable to use in every situation or healthcare facility. A combination of different methodologies may provide the most sensitive culture, but this is not usually feasible. The shifting prevalence of commonly identified species and emerging pathogens may force the use of algorithms to provide guidelines for the most effective use of resources. Many of the blood-culture systems described here are also described and depicted elsewhere in this text in the sections describing bacterial blood-culture systems. The Wampole Isolator System (Alere North America, Orlando, FL) lysis-centrifugation blood-culture method has long been regarded as one of the most sensitive systems for the detection of fungemia. Blood-collection devices are available as 10 mL draws and as 1.5 mL draws for children [236]. The Isolator system (Table 13.3, Figure 13.5) uses saponin to lyse erythrocytes and leukocytes, releasing microorganisms from white blood cells and inactivating inhibitory serum factors and antimicrobial agents. In this system, the blood is lysed before organisms are concentrated by centrifugation. The sediment is subsequently inoculated to solid media [294]. Improvements to and automation of broth-based systems have made them an attractive possibility for the future, but continued studies performed with the Isolator system reconfirm its merit for the detection of fungemia, primarily for the detection of filamentous fungi. Table 13.3 Comparison of Isolator method with other blood-culture methods and systems TTD, time to detection. Figure 13.5 ISOSTAT system (Isolator). (Courtesy of Alere.) The Isolator system has been proven to be more rapid and sensitive than detecting fungi in vented biphasic or broth media, especially for the recovery of Histoplasma capsulatum [117, 213, 294]. It was also found to be superior to the other broth-based systems [31]. In one retrospective study, 23,586 matched pairs of fungal blood cultures were compared, and the Isolator system enhanced the detection of fungi by as much as 30% compared to the Septi-Chek system (Becton, Dickinson and Co., Franklin Lakes, NJ; please check on the availability of this product in your region or country) [124]. Fungal recovery can be enhanced by as much as 15–50% in comparison to broth cultures, and time to recovery is generally decreased [117]; however, newer automated systems have compared more favorably than the traditional broth-based fungal cultures. In one study the Isolator method had the highest recovery rate and shortest recovery time compared to both the BACTEC and Septi-Chek systems [294]. When the Isolator and BACTEC Plus 26 aerobic medium were compared to the BACTEC high-blood-volume fungal medium (HBV) for 93 test isolates, HBV proved to be comparable, except for the recovery of H. capsulatum, where the Isolator system was superior. In contrast, Candida glabrata was detected earlier in HBV than in the Isolator system. In another study the Isolator system proved to be superior to the BACTEC Plus 26 aerobic medium for recovery of H. capsulatum, C. glabrata, and other fungi when 86 cultures positive for fungi were tested [321]. The Isolator system also detected significantly more Candida spp. than the ESP 80A or the Septi-Chek system [54]. A significantly improved recovery rate was also documented for 171 isolates of C. albicans and Candida parapsilosis [159]. The Isolator system was also found to be superior, especially for Candida spp., H. capsulatum, and C. neoformans, to the BacT/Alert system. In 22 cases of candidemia, the Isolator system recovered 100% of the isolates, with a mean time to detection of 2.9 days (range, 0–9 days), while the BacT/Alert system recovered 68.2% of the isolates, with a comparable mean time to detection of 2.1 days (range, 0–5 days). Histoplasma capsulatum and C. neoformans were recovered only by the Isolator system [181]. When the small volume Isolator 1.5 was compared to the BACTEC NR660 system aerobic NR6A blood-culture bottle for detection of fungal infections in pediatric patients, NR6A was found to be comparable to Isolator 1.5 in both yield and recovery time [236]. In another study with 53 isolates of C. neoformans, the Isolator system detected 47 while the BacT/Alert system detected 23. Of 18 isolates of H. capsulatum, the Isolator system detected 18 and the BacT/Alert system detected four. Time to detection was shorter for the Isolator system, 14 days for H. capsulatum and 6 days for C. neoformans, compared to the BacT/Alert with 18 and 11 days, respectively [23]. It should be noted that with current recommendations for holding automated blood cultures for 5 days without terminal subculture would have resulted in negative cultures for both H. capsulatum and C. neoformans. In a 1998 comparison, the BACTEC 9240 system with MYCO/F lytic medium was compared to the Isolator system. Of 24 blood cultures positive for fungi, 12 were positive in both systems, seven were positive only in the Isolator system and five were positive only with the MYCO/F lytic bottle. Of 14 positive blood cultures containing H. capsulatum, seven were positive in both systems and seven were positive only in the Isolator system. For fungal isolates in this study, the mean times to detection were similar at 8–9 days, but the organisms were more quickly identifiable from the Isolator plates. For C. neoformans, the MYCO/F bottle may have an advantage. Of ten blood cultures positive for C. neoformans, five were positive in both systems and five were positive in only the MYCO/F system. The mean time to detection was 4 days in the MYCO/F system and 7 days with the Isolator system [312]. Despite its widespread use, the Isolator system can produce false-positive results due to contamination events related to the multiple-step processing of Iso-STAT tubes; this is observed mostly with bacterial isolates and not with fungi. It is important to monitor false-positive rates in individual laboratories to identify the case definition of fungemia, and to determine the types of organisms isolated in each patient population [206, 207] In one retrospective study, the utility of the Isolator system for the detection of fungal recovery was compared to that of culture in the BACTEC NR660 system. Strict definitions for both positive and false-positive blood cultures were given, specific to the study. Of 66 positive fungal blood cultures, 42 were considered to be due to contaminants, and all except one derived from the Isolator system. The use of these definitions produced summary results with 16 different species of fungal contaminants [58]. Similarly, relatively high contamination rates were reported in other studies and may detract from the cost-effectiveness of a system that is very labor- and cost-intensive [106, 157, 210, 294]. Clearly, the utility and cost-effectiveness of the Isolator system for fungal blood cultures will vary with institutional blood-culture collections, patient population, processing, and contamination rates. A major advancement in the diagnosis of both bacteremia and fungemia was the development of the continuously monitored blood-culture systems. Each of the commercially available systems are effective but vary in the type of media and specimen types, other than blood, that are offered (Table 13.4). Table 13.4 Continuous monitor blood-culture system The BacT/Alert system (bioMérieux, Durham, NC) is a continuously monitored closed blood-culture system for which no special fungal media are currently available. The system is based on the photochromatic detection of CO2 as organisms grow in the broth. In 2004 Horvath et al. [139] used blood-culture bottles with blood from healthy donors and seeded with Candida spp. to compare recovery by the BacT/Alert (FA, SN, and MB+suppl) to the BACTEC 9240 (Plus Aerobic/F, Plus Anaerobic/F, and Myco/F Lytic bottles). Fifty clinical isolates, 10 of each species were used. Six bottles representing each medium received 103 CFU (colony-forming units) of each strain of C. albicans, C. glabrata, Candida krusei, C. parapsilosis or Candida tropicalis and incubated per manufacturers’ instructions. Of the 300 bottles inoculated with Candida spp, 235 (78%) grew the corresponding strain. The remaining 65 bottles not flagged by the instruments by day 12 were subcultured and all of the corresponding organisms were grown. A breakdown of the number of positives detected by the instruments revealed that the BACTEC Myco/F, BacT/Alert FA, and BacT/Alert MB bottles recovered all isolates. This was in contrast to the BACTEC Plus Anaerobic (45 negatives), BacT/Alert SN (15 negatives) media, and the BacT/Alert SA (five negatives). Most laboratories do not include mycology media in the routine blood-culture protocol. When comparing only the aerobic and anaerobic media, the BacT/Alert performed better than the BACTEC in overall detection, time to detection, number of false-negatives, and missed episodes of simulated candidemia [139]. Although the BacT/Alert system had slightly diminished capacity for the detection of some fungi [181], use of the FAN aerobic media isolated significantly more yeasts than standard aerobic medium, with the same mean time to detection [313]. When compared to the standard anaerobic bottle, vented for aerobic use, the FAN bottle recovered significantly more Candida spp. [57]. In contrast, when the FAN anaerobe bottle was compared to standard aerobic medium, more C. glabrata isolates were recovered from the aerobic medium [117]. When the BacT/Alert system was compared to the BACTEC NR660 or 730 system, the BacT/Alert system had a noticeable but not statistically significant advantage in recovery of fungi; however, terminal subcultures of both systems added many of the fungi that otherwise would not have been recovered [322]. Saito et al. compared the FAN BacT/Alert media to standard media in a before-and-after study over a 4-year period. Although not focused on candidemia they did find that the FAN formulations had a higher percentage of positives for Candida spp. [267]. Various models of the BACTEC blood-cultures systems (Becton, Dickinson and Co., Sparks, MD) are used in clinical laboratories worldwide. Older models, based on the radiometric, interval-based detection of CO2, have been replaced by nonradiometric (NR) systems and, most recently, by the BACTEC 9000 and FX systems, which use fluorescent sensor technology to continuously monitor CO2 production. Barcode-scanning and data management systems are also included with these systems. In a study of the BACTEC NR660 and Oxoid Signal systems (Remel), the BACTEC system proved to have the advantage in detection time. The NR660 system isolated 18 yeasts, with median times to detection of 39 h compared to 168 h for the Signal system [277]. In 1992, BACTEC PLUS high-volume resin (BP-HBV) and aerobic BP26 and anaerobic BP27 vials were compared to standard BACTEC aerobic NR6A and anaerobic NR7A vials. BACTEC PLUS vials enhanced the recovery rate for C. albicans for patients receiving antibiotics [184]. The BACTEC system has a slightly diminished capacity for detection of some fungi compared to the Isolator system. When BACTEC HBV was compared to the Isolator system and BACTEC BP26 with 93 isolates tested, HBV proved to be comparable, except for the recovery of H. capsulatum, where the Isolator system was superior. In contrast, C. glabrata was detected earlier in HBV than in the Isolator system. For HBV versus BP26, 68 positive fungal isolates were identified, and HBV was found to be superior for C. glabrata and all fungi combined. No difference was noted in the mean time to detection [321]. The BACTEC 9240 system was evaluated in comparison with the conventional BACTEC NR730 system using two media with the same formulation: NR26 for the BACTEC NR730 system and Aerobic Plus F for the BACTEC 9240 system. Simulated blood cultures were prepared for 41 strains belonging to 18 different fungal species, meant to represent fungi that are responsible for hematogenous dissemination. In a second phase of the study, two different media, HBV and NR26, were both compared on the BACTEC NR730 system. The differences between the two systems and the two media (NR26 and HBV) were not statistically significant, but use of HBV did improve the detection of fungi on the BACTEC NR730 system. In addition, the BACTEC 9240 system had a mean detection time that was significantly shorter than that of the BACTEC NR730 system [109]. In one of two 1998 studies, simulated blood cultures were used to compare the BACTEC 9240 medium (Mycosis IF/C) with the BACTEC standard medium, the Aerobic Plus F. In this study, 43 strains and 10 species of yeast were tested. The median time to detection was 29.03 ± 13.99 h for Mycosis IF/C compared to 73.92 ± 56.74 h for Aerobic Plus F medium. Mycosis IF/C reduced the time to detection [108]. In another study, specifically targeting C. neoformans, the MYCO/F medium appears to have an advantage over the Isolator system. Of ten blood cultures positive for C. neoformans, five were positive in both systems and five were positive in only the MYCO/F medium. The mean time to detection was 4 days in MYCO/F and 7 days with the Isolator system [312]. The VersaTREK (Trek Diagnostic Systems, Inc. Cleveland, OH, now ThermoFisher, Waltham, MA) is the current evolution of what was originally the DIFCO ESP system, which was acquired by Trek Diagnostics and now marketed through ThermoFisher Scientific. The VersaTREK and its predecessor the DIFCO ESP blood-culture system detect changes in bottle headspace gas pressure due to oxygen consumption and CO2 generated by microbes. In 1994, the ESP system with ESP 80A and 80N bottles was compared to the BACTEC NR660 system using BACTEC 6A/7A, 16A/l7A, or pediatric bottles. In this study, ESP 80N anaerobic bottles detected significantly more Candida spp. [209]. In an assessment of neonatal sepsis, the ESP blood-culture system isolated 41 yeasts. By 48 h of incubation, 88% (36 of 4l) of those isolates were identified as positive cultures. There was no difference in the time to positivity in pre-therapy and post-therapy blood cultures [114]. In a comparison of the ESP and the BacT/Alert systems, no significant difference was documented for 31 yeast isolates [327]. Differences in instruments and changes in media formulation make the early studies of this system less relevant. Only a few comparison studies using the current system have been published. Mirrett et al. performed a comparison of the VersaTREK, using REDOX 1 aerobic, REDOX 2 anaerobic media, to the standard aerobic (SA) and anaerobic (SN) media of the BacT/ALERT blood culture systems with patients suspected of having sepsis [202]. The fungal isolates were all Candida spp. (14 C. parapsilosis, 12 C. glabrata, nine C. albicans, four C. tropicalis and one C. krusei). No breakdown of the results for each of the species was provided, so the data are expressed as an aggregate of the total number of 40 isolates. Yields for Candida spp. were the same for both systems (23 both systems, eight VersaTREK only, nine BacT/ALERT only). This was also true when selecting only clinically significant isolates (14 both systems, six VersaTREK only, three BacT/ALERT). The mean times to detection were 35.8 h and 37.5 h respectively for VersaTREK and BacT/ALERT. They conclude that the systems are not significantly different in their ability to detect Candida spp. with the same time to detection. A second study compared the VersaTREK REDOX 1 medium to the BACTEC FX Plus Aerobic/F medium using bottles seeded with 10–100 CFU of clinical isolates and ATCC reference strains of C. albicans (10), C. glabrata (4), C. tropicalis (3), C. parapsilosis (2), C. krusei (1), C. neoformans (3) and C. laurentii (1) [265]. All isolates tested susceptible using Sensititer YeastOne system (TREK) to amphotericin B, fluconazole, voriconazole, and caspofungin with the exception of C. glabrata, which were susceptible–dose dependent (S-DD) to fluconazole. A total of 721 seeded blood-culture bottles were incubated with and without antifungal agents in each of the systems. Candida krusei, C. neoformans (3) and C. laurentii (1) failed to be detected, were negative on terminal subculture, and therefore not included in the analysis. In the absence of antifungals both systems had comparable recovery rates, with VersaTREK detecting 99.1% versus 97.4% for BACTEC (p = 0.154). VersaTREK had the advantage in time to detection (2.2 h faster, p = 0.001) in the absence of antifungals but the BACTEC system had a significant advantage when antifungals were present (10.8 h faster, p = 0.001). Available media include: Figure 13.6 ChromID Candida agar. (Courtesy of bioMérieux.) Figure 13.7 Different appearance of C. albicans, C. tropicalis, C. glabrata, and C. krusei. (Courtesy of Bio-Rad Laboratories, Inc.) CHROMagar is a chromogenic differential and selective medium for isolation and differentiation of C. albicans, C. tropicalis, and C. krusei directly from clinical specimens. The suggested incubation temperature is 35 ± 2°C. Colony color and morphology are used for identification purposes. Candida albicans grows as light to medium green colonies with slight green halos due to the presence of the enzyme β-N-acetylgalactosaminidase [1]. Candida tropicalis grows as dark blue to metallic blue colonies. Candida krusei, the isolation and identification of which are important because of its resistance to the common antifungal fluconazole, grows as large light mauve to mauve colored crenated colonies with white edges. CHROMagar supports the growth of yeasts and most fungi while inhibiting most bacteria due to the presence of chloramphenicol. In another study of CHROMagar [222], the presumptive identification of C. albicans, C. krusei, and C. tropicalis exceeded 99% for all three species when blinded reading tests were performed by four different personnel. Several occurrences of potential misinterpretation are noteworthy, although such instances are rare. For example, rare isolates of C. norvegensis produced colony morphology similar to that of C. krusei. In addition, some Geotrichum spp. and Pichia spp. formed colonies with a grey, blue, or green color or a dark halo in the agar. In addition, Candida dubliniensis has been reported to grow as dark-green-colored colonies upon primary isolation [160, 275]; however, the presence of this phenotype is variable. When compared to identification by DNA sequencing, only 56% of all C. dubliniensis isolates tested (n =53) displayed the green phenotype [297]. In one study [269], in which 1537 yeast isolates were tested and read after 48 h of incubation, sensitivity and specificity were 99% and 100% for C. albicans, 93.8% and 99.1% for C. tropicalis, and 100% and 100% for C. krusei. A large sample size makes this comparison noteworthy, with 970 C. albicans, 165 C. parapsilosis, 131 C. glabrata, 62 C. guilliermondii, 35 C. krusei, 32 C. tropicalis, 31 Rhodotorula rubra, and 23 Trichosporon spp. and a variety of other species. The accuracy of CHROMagar is reported to be similar to those of germ-tube tests and chlamydospore development for the identification of C. albicans [268]. Additionally, CHROMagar was evaluated for 618 yeast isolates and 128 direct specimen inoculations. After 2 days at 37°C, the sensitivities for identifying species were as follows: C. albicans, 99.4% (n = 341); C. glabrata, 98.9% (n = 99); and C. krusei (n = 35) and C. tropicalis (n = 73) 100%. The specificities were 100%, 99.8%, 99.6%, and 99.6%, respectively. The authors also report CHROMagar to be useful for the identification and determination of mixed cultures [22]. In another study [21] detection rates for C. albicans were reported to be as much as 20% higher than those reported with Sabouraud-chloramphenicol plates (n = 951), with 92.2% correct identification after 72 h. The specificity was reported to be 100%. Another study [107] compared CHROMagar to two other commercial media for identification of Candida spp., albicans ID and CandiSelect (Sanofi Diagnostics Pasteur), both of which contain fluorogenic substrates in the agar. All were compared with isolation on traditional Sabouraud-chloramphenicol agar medium followed by standard yeast identification. A total of 192 clinical specimens were tested. For all yeast species, CHROMagar yielded the largest colonies. The identification rates for C. albicans were nearly equivalent at 86.59%, 83.79%, and 83.24%, respectively. At 72 h, albicans ID, Candi Select, and CHROMagar identified 92.75%, 91.3%, and 88.57% of the specimens, respectively, but at 24 h the sensitivities for growth and specific pigment were substantially lower at 56.6%, 37.68%, and 11.59%, respectively. Although CHROMagar did not identify C. albicans as quickly as the other two commercial identification media, the agar allowed correct identification of C. tropicalis, the main source of false-positives on most other chromogenic media. In addition, the authors report that morphology is easier to read with CHROMagar. In 1998, two studies were reported. In one [1], 21 yeast isolates from neutropenic and AIDS patients were tested, both on CHROMagar and Sabouraud dextrose agar. The overall sensitivities for detecting C. albicans were the same for both media. The primary isolation on CHROMagar was found to be 100% sensitive and 100% specific for C. albicans. For identification purposes, CHROMagar proved to be the most economical and the least time-consuming method. The authors of the study suggest its use for blood-culture plating when yeasts are seen in microscopy or when early therapy is imperative. In another study [253], CHROMagar Candida was tested with a total of 262 yeast isolates: C. albicans (173); C. tropicalis (21); C. krusei (8); C. glabrata (49); and other yeasts (12). Compared to conventional identification C. albicans was correctly identified 98% of the time. In addition, CHROMagar helped identify 37 of 46 mixed cultures that were otherwise not detected. The authors suggest that it is reasonable to include CHROMagar for identification of C. albicans and as an aid in identification of mixed cultures. Recently CHROMagar performance for clinical specimens was compared with Candi Select 4 (current formulation of Candi Select, Bio-Rad), which identifies C. albicans by detecting hexasaminidase resulting in pink to purple-colored colonies [278]. Phosphatase activity was used to presumptively identify C. tropicalis, C. glabrata, and C. krusei based on turquoise colonies associated with a typical morphologic appearance (Figure 13.8). Chloramphenicol and gentamicin were included to suppress bacteria present in the specimen. A total of 502 cultures were positive, 37 of these were mixed, with a total of 542 yeast isolates. The species distribution was 392 C. albicans, 60 C. glabrata 25 C. tropicalis, 12 C. krusei, and 53 others as identified by standard phenotypic methods. Correct identification of C. albicans was equal at 24 h (31.2%; 31.9%), 48 h (82.9%; 82.9%) and 72 h (92.1%; 91.1%). Presumptive identification of C. glabrata, C. tropicalis, and C. krusei was evaluated at 48 h. Percentage of strains with typical colonies was 80, 68, and 84.6% respectively for CandiSelect 4, and 75, 76, and 76.9% for CHROMagar. Eraso et al. [82] tested 100 isolates each of C. albicans and C. dubliniensis on CHROMagar Candida (Paris, France), reformulated CHROMagar Candida (BD, BBL Cockeysville, MD), and Candida ID2 (now chromID Candida). They found that Candida ID2 gave the best resolution with a positive predictive value of 91.7% and negative predictive value of 100% for identifying C. dubliniensis. Several studies have evaluated bismuth sulfite glucose glycine yeast (BIGGY) agar (Remel Microbiology, Lenexa, KS) for isolation and identification of yeasts. One [325] comparing CHROMagar to BIGGY agar examined 169 C. albicans, 33 C. tropicalis, 24 C. glabrata, 18 C. parapsilosis, 12 C. krusei and other Candida and yeast. They found CHROMagar sensitivity and specificity for C. albicans of 99.4%, 100% and for BIGGY 87%, 78.2%. The sensitivity for C. tropicalis, C. glabrata, and C. krusei on CHROMagar was 97%, 90.9%, and 100% and on BIGGY agar 66.6% (C. tropicalis) and 100% (C. krusei). In a different study [326] by the same authors examining 215 yeast isolates on BIGGY agar, CHROMagar, and albicansID2 found the same difference in sensitivity when comparing CHROMagar and BIGGY agar. In 2007 a study compared BIGGY agar to albicans ID2 and Sabouraud agar with chloramphenicol for isolation and direct identification from vaginal cultures [143]. They did not find any difference in the number of yeasts isolated on each of these media. They found that BIGGY agar had a sensitivity of 86.7% and specificity of 56.3% compared to albicans ID2 sensitivity of 80% and specificity of 64.6% for C. albicans. An additional chromogenic agar, HardyCHROM Candida (Hardy Diagnostics), is available, however, as of this writing there are no publications indicating performance relative to the other chromogenic media. In testing with 92 isolates, all C. glabrata isolates grew better on eosin-methylene blue agar plates than on blood agar plates at 24 h. The criterion of better growth on EMB agar is used as an identification method for C. glabrata and is combined with the germ tube test as a cost-effective method for identification of C. glabrata and C. albicans [16]. Traditionally, C. albicans has been identified by the germ tube test. In 1987, Perry and Miller evaluated the use of 4-methylumbelliferyl-N-acetyl-β-galactosamine (NAG) to differentiate between C. albicans and C. tropicalis [233]. Later, the enzymes L-proline aminopeptidase and β-galactosaminidase further increased the specificity of the test [234]. Candida albicans produces both enzymes, while other yeasts produce only one or neither. The MUREX Candida albicans Test Kit (Remel Microbiology) test uses the enzyme substrates p-nitrophenyl-N-acetyl-β-D-galactosamide (NAG) and L-proline-β-naphthylamide, incorporating them onto a paper disk in a kit-provided test tube. The disk is moistened with distilled or deionized water, and a heavy inoculum of yeast is placed on it with an inoculating loop. The tube is capped and incubated at 35–37°C for 30 min, after which 0.03% sodium hydroxide is added. If one or both of the enzymes are present, the substrates will be hydrolyzed and a colored reaction will be noted. Candida albicans is positive for both enzymes; other yeasts may be positive for one or the other. The BactiCardTM Candida (Remel Microbiology) contains NAG and L-proline-β-naphthylamide; the latter is the same substrate found in the Murex system. These substrates are also dehydrated on a packaged test card, which is rehydrated prior to testing and inoculated with a thick paste of yeast cells. If the substrates are cleaved after 5 min of incubation at room temperature, one fluorescent reaction product, 4-methylumbelliferone, and one colorimetric reaction product will be produced. The fluorescent product is viewed under longwave UV light. The test can be performed on pure cultures that are 18–72 h old. These tests were compared to the traditional germ tube test for presumptive identification of C. albicans [59]. The API 20 C AUX test (bioMérieux) and conventional methods were used as the gold standards. A variety of yeasts were tested, including C. albicans (303), C. glabrata (153), C. tropicalis (70), C. parapsilosis (36), Cryptococcus spp. (5), S. cerevisiae (3), and other yeasts (13). Murex C. albicans, BactiCard, and the germ tube test identified 98.7%, 99.3%, and 94.7% of C. albicans isolates, respectively. One false-positive each was observed using the Murex and BactiCardTM Candida (Figure 13.8) tests, while two false-positives were seen with the traditional germ tube test. All of the enzymatic methods were noted to be rapid and accurate alternatives to germ tube testing of C. albicans. In another study [135) these tests were also used to test 133 yeast isolates in comparison with the germ tube test and the API 20 C AUX test and were found to correlate 100%. All of the assays generated some false-positive results for the non-Candida yeasts. The assays were compared for cost and other parameters. Figure 13.8 Thermo Scientific™ Remel™ BactiCard™ Candida Kit. (Courtesy of ThermoFisher Scientific, copying is prohibited.) The Murex C. albicans test was also compared with standard 2-h germ tube tests (n = 502). In this study, the number of C. albicans isolates tested was 316; the number of non-albicans yeasts tested was 186. Discrepant reactions were confirmed with the API 20 C AUX test and by chlamydospore production. Two yeast isolates, C. albicans and Candida lusitaniae, gave incorrect results with the Murex test. Compared to the germ tube test in fetal bovine serum and Fetal Clone II, the sensitivity and specificity of the Murex test were 94.6% and 97.8%, respectively [100]. The AlbiQuick (Hardy Diagnostics, Santa Maria, CA; Figure 13.9) is an assay that measures the presence of enzymes that will hydrolyze the proline-p-nitroanilide (PRO) and NAG compounds on a card with two reaction wells for the identification of C. albicans. No peer-reviewed studies have been reported as of this writing. Figure 13.9 AlbiQuick. (Courtesy of Hardy Diagnostics.) In a 1996 study, a screening protocol for C. glabrata based on trehalose fermentation at 42°C for 24 h (Trehalose Fermentation Broth; Hardy Diagnostics) was compared to identifications by the API 20 C AUX and Microscan Rapid Yeast Identification Panel. Trehalose Fermentation Broth was found to have 97.8% sensitivity and 95.8% specificity when used for presumptive identification of C. glabrata [170]. A follow-up study [98] comparing three commercial methods for the rapid identification of C. glabrata included the 24 h Trehalose Fermentation Broth, the 3 h Remel Rapid Trehalose Assimilation Broth, and the 24 h Remel Yeast Fermentation Broth (Remel Laboratories) to the API 20C AUX. All of the methods were compared for accuracy, cost, and turnaround time. Sensitivities and specificities were 91.5 and 96.3% (Remel assimilation), 95 and 89% (Remel fermentation), and 96 and 100% respectively. A rapid assimilation panel, GlabrataQuick (Hardy Diagnostics) includes maltose and sucrose in addition to trehalose, which reduces false-positive results (Figure 13.10). Figure 13.10 GlabrataQuick. (Courtesy of Hardy Diagnostics.) Identification of C. dubliniensis is a relatively new diagnostic dilemma, and few commercial systems have been tested. In one such study [112], 66 isolates of C. dubliniensis and 100 isolates of C. albicans were tested with the API 20 C AUX and the Vitek Yeast Biochemical Card (YBC) systems. The results were specifically focused on the abilities of the respective systems to identify xylose and α-methyl-D-glucoside (MDG) utilization by C. albicans and lack of utilization by C. dubliniensis. Growth at 45°C was also examined. The results suggested that clinical laboratories could use the lack of growth at 45°C and negative xylose utilization with either the API 20 C AUX or Vitek system to presumptively identify C. dubliniensis. Less correlation existed with a negative MDG test on either system, and misclassification may occur if this result is used, especially with the API 20 C AUX system. Ells et al. published a review of the reliability of methods for distinguishing C. dubliniensis from C. albicans [77]. Methyl blue Sabouraud agar contains 0.01% methyl blue as a supplement to Sabouraud agar [119]. This medium has been reported to distinguish between C. albicans and C. dubliniensis based on the yellow fluorescence of C. albicans under a Woods lamp [275]. In 1962 Staib observed that when yeasts were grown on media containing Guizotia abyssinica seeds that Cryptococcus neoformans formed brown colonies while other yeasts were nonpigmented [287]. Subsequently it was determined that the seeds contain caffeic acid (3,4-dihydrocinnamic acid), which is the melanin-producing component. Phenol oxidase activity results in the brown pigment of C. neoformans and C. gattii colonies. Incubation requires up to 7 days before considering it negative. An attempt to circumvent this limitation was undertaken using a disk containing caffeic acid, which is placed on a glass slide, moistened with water or a nonglucose-containing agar, and inoculated with 5–6 colonies and incubated at 35°C in the dark for up to 4 h. The production of a brown pigment should be considered presumptive and the identification verified by another test. Also, it does not differentiate between C. neoformans and C. gattii. Both the caffeic agar and disks are available from Hardy Diagnostics and Remel. The majority of clinical disease in immunocompromised patients is due to C. neoformans var. neoformans and C. neoformans var. grubii. The recognition of C. gattii as an emerging pathogen in immune competent individuals makes the differentiation of the two species important. Kwon-Chung et al. in 1982 reported a selective medium which differentiated between C. neoformans and C. gattii based on the ability of C. gattii to grow in the presence of canavanine, and uses glycine as a sole carbon and nitrogen source resulting in a shift in pH detected by bromothymol blue (CGB agar; Figure 13.11) [168]. This did not receive broad acceptance in clinical diagnostic laboratories outside areas where C. gattii had been initially described, e.g., tropical and subtropical regions [167]. More recent studies utilizing mostly molecular methods have demonstrated a much wider distribution of C. gattii in North America [167], making it more important to differentiate the species. In 2009 Klein et al. [163] compared the ability of CGB to differentiate between C. neoformans and C. gattii and a number of other yeasts. They found that 100% of C. gattii grew and turned the media blue (positive) while 100% of C. neoformans did not. They also found that other Cryptococci, Trichosporon spp., and some Candida spp. also would give a positive reaction on CGB agar. Figure 13.11 CGB agar for the differentiation of C. gattii (positive) from C. neoformans. (Courtesy of S. Moser.) A recent study evaluated Remel rapid urea broth and BD BBL urease test broth, caffeic acid disk, CGB, and internal transcribed spacer (ITS) sequencing in 147 reference and clinical strains of Cryptococcus spp., Candida spp., Trichosporon spp., and other non-Cryptococcus species [192]. All of the cryptococci were urease positive as well as Rhodotorula and Trichosporon spp. Addition of the caffeic acid result eliminated all of the noncryptococci with 85 of 86 (99%) C. neoformans positive and 26 of 27 (96%) C. gattii positive. Utilizing these two rapid tests, identification of C. neoformans/gattii was made in 4 h. Within 48 h further separation of the species was achieved using CGB and/or ITS sequencing. A positive CGB was found in 25 of 27 (93%) of C. gattii and none of the C. neoformans. It required ITS sequence to find the urea positive, caffeic-acid positive, and CGB negative C. gattii. The CGB agar is available from Hardy Diagnostics and Remel. The advantages of the nonautomated and automated identification systems discussed here include the fact that identification is based on databases that include a variety of substrate utilization patterns and that contain many yeast biotypes. The Uni-Yeast-Tek (Remel) system was the first widely used commercial system for yeast identification. It consists of a sealed multiwell plate, with each well containing medium for testing carbohydrate utilization, nitrate utilization, urease production, and morphology on cornmeal agar. The kit also includes supplementary tests, such as the C-N screen, glucose beef extract, and sucrose assimilation medium tubes. Evaluations of this product have shown it to be generally satisfactory for the identification of commonly isolated yeasts [254]. However, the system may require up to 7 days for complete identification. The API 20 C AUX (bioMérieux) test is a micromethod for carbohydrate assimilation of clinically important yeasts. Yeasts are inoculated into basal medium in test strips containing dehydrated substrates for assimilation testing. The API 20 C AUX system consists of a strip that contains 20 microcupules; all but two contain dehydrated substrates for determining substrate utilization profiles. As the yeasts utilize various substrates, the microcupules will appear cloudy (Figure 13.12). The reactions are compared to the first reaction cupule, which does not contain a carbohydrate substrate. The test strips are incubated at 30°C for 24–72 h. The results of assimilation reactions are read and converted to a seven-digit biotype profile number. In addition, the system requires that the microscopic morphology be documented on cornmeal agar containing 1% Tween 80 and that this information be used in conjunction with the substrate utilization data for yeast identification [254]. This yeast identification system is perhaps the most widely evaluated and well published of all the current commercial systems. It is frequently used as the gold standard against which comparisons are made. Figure 13.12 API 20 C strip for identification of yeast isolates. Close-up view of substrate utilization of a yeast isolate. Positive reactions appear cloudy in comparison to the negative control (0). (Courtesy of bioMérieux.) In early studies [328] performed to compare the API 20 C AUX test with conventional methods, 98% correlation was documented (n = 45). The system was considered to be an acceptable substitute for conventional carbohydrate fermentation and assimilation tests. In a more recent study [276], 178 yeasts were tested. After 72 h, correct identification occurred for 86.5% of those isolates tested by the API 20 C AUX system compared to 86% with Auxacolor (Sanofi Diagnostics Pasteur), 68% with Mycotube (Roche Diagnostics), and 51.1% with Candifast (International Microbio, Milan, Italy). When 100 common yeasts were tested, a correct identification was obtained for 98% of the isolates with Vitek YBC (bioMérieux) and 100% with the API20 C AUX [126]. In another study, 123 common and 120 rare clinical yeast isolates were tested, and the API 20 C AUX test correctly identified 97 and 88%, respectively [256]. A further evaluation reported 90.8% of 120 yeast and yeast-like isolates were correctly identified by the API 20 C AUX test [132]. The RapIDTM Yeast Plus system (Innovative Diagnostic Systems; sold as IDS YeastPlus Panel from Remel/ThermoFisher) is a qualitative method that uses conventional and chromogenic substrates to identify carbohydrate utilization, hydrolysis of fatty acid esters, and constitutive enzymes of yeast and yeast-like organisms. It combines conventional tests and single-substrate chromogenic tests with 4-h incubation. A suspension of pure yeast culture is made in the RapID inoculation fluid, turbidity is standardized, and the suspension is used to rehydrate the biochemicals in the RapID plastic reaction panel (Figure 13.13). With a one-step inoculation, the suspension will disperse in the panel by way of the plastic inoculating trough. After a 4-h incubation at 30°C, the RapIDTM Yeast Plus system is interpreted. Some reactions require additional reagents to be added to the reaction panel. The resulting pattern of positive and negative reactions is used to compile a profile number based on the organism’s individual reactions in the panel. The RapIDTM Yeast Plus Code Compendium supplies species identification for the biochemical profile numbers, which are generated based on the results of biochemical reactions. The RapIDTM Yeast Plus method correctly identified 94.1% of 304 clinical yeast isolates within 5 h [162]. Figure 13.13 Thermo Scientific™ RapID™ YEAST PLUS System. (Courtesy of ThermoFisher Scientific, copying is prohibited.) In a study of 201 yeasts [311], when no further tests were performed, identification to the species level was 96%, 83%, and 86% for the RapIDTM Yeast Plus system, the API 20 C AUX system and the Vitek YBC respectively. The API 20 C AUX system did not correctly identify any C. krusei isolate without supplemental testing, and this organism accounted for most of the differences seen between the API 20 C AUX system and the RapIDTM Yeast Plus system. In another study [135] the RapIDTM and API 20 C AUX systems were compared with 57 C. albicans isolates, 26 C. tropicalis isolates, 23 C. glabrata isolates, and 27 isolates from other species. The RapIDTM system correctly identified 125 (94%) of all yeasts tested and 99% (105 of 106) of the isolates of the three most common species, C. albicans, C. tropicalis, and C. glabrata. Discrepancies were resolved with Vitek 2 Yeast ID cards (bioMérieux), germ tube production, and microscopic morphology and confirmed that the RapIDTM system compared favorably with the API 20 C AUX system but had a more rapid identification process. In another study, the identification rate for yeasts tested with this system was 78–84% [38]. In a similar study, good correlation was observed between the two systems, with 95.7% agreement with the API 20 C AUX system for Candida and Cryptococcus. Lower agreement was noted with emerging Candida spp. and with other, yeast-like pathogens (79.1 and 75.2%) [85]. In another study, the RapIDTM Yeast Plus system was compared with the Uni-Yeast-Tek system (Remel). A total of 117 fresh and frozen yeast isolates were tested. The bioMérieux Vitek system and morphology were used to resolve discrepant results. The RapIDTM system correctly identified 96.6% of the isolates in 4 h [207]. Traditional microbiological techniques have long relied on the phenotypic characteristics of an organism including morphology, biochemical profiles, and culture patterns. Over the past several years, molecular techniques including genetic probes, polymerase chain reaction (PCR), and sequencing have become increasingly prevalent in microbial identification algorithms. Recently, MS has been adapted to determine the physical protein expression profile of an organism and compare it to known profiles in a database to identify organisms in patient samples. Mass spectrometry was previously used for bacterial identification in 1975 in a process that focused on lipid analysis. In the 1990s, MALDI-ToF/MS was used to identify surface proteins of bacteria. A change of matrix composition allowed the analysis of bacterial ribosomal proteins that is considered more reliable for microbial identification [274]. MALDI-ToF works by embedding analytes in a crystalline matrix. A laser is utilized to vaporize the analytes, which are then ionized and detected. MALDI-ToF/MS measures the mass-to-charge ratio of a given sample and compares it to a library of reference spectra to establish identification. Results can be obtained either from subculture or directly from blood cultures, and a protein extraction step may be utilized depending on the platform, especially for fungal organisms. This technology is useful for the identification of cultures containing a single species but is currently insufficient to detect mixtures of organisms [74]. The sample is placed with the matrix on a conductive metal plate that can then be inserted directly into the mass spectrometer. Obtained spectra results are compared to reference spectra within the database, thereby leading to identification of the organism. Currently, two commercially available MALDI-ToF/MS platforms have received the Conformité Européenne (CE) mark for in vitro diagnostic use in Europe. The Bruker Biotyper system, utilizing the Microflex LT mass spectrometer and Biotyper database (Figure 13.14; Bruker Daltonics), and the bioMérieux Vitek MS system (Figure 13.15), utilizing the MS-ID database (bioMérieux, previously developed by AnagnosTec and Shimadzu Corporation), are currently available for the identification of bacterial and fungal pathogens [307]. An additional Andromas database (Andromas SAS, Paris, France) can be utilized in conjunction with the Bruker system. Interpretation of MS data is conducted by algorithms such as the Bruker Main Spectrum analysis or the bioMérieux Advanced Spectra Classifier for the profiling of the presence or absence of peaks. At the time of this writing, the majority of published studies relating to fungal identification utilize the Bruker MALDI-ToF platform coupled to the Biotyper database. The Sepsityper system (Bruker Daltonics) can be utilized to identify organisms directly from blood culture [148]. For fungal organisms, a protein extraction step substantially improves identification for the Bruker system in combination with the Biotyper database; however a protein extraction step is not required for the Vitek MS system [144]. At the time of this writing, the bioMérieux Vitek MS system has received FDA approval for the diagnosis of 193 clinical bacterial and yeast organisms, while the Bruker platform has FDA approval for an aerobic Gram-negative organism database including 40 species and species complex (100 total identifications). Advantages of MALDI-ToF/MS include low cost of consumables, fast analysis turnaround times, and easy interpretation of identifications. The reader should consult current publications and vendor web sites for up-to-date information since this is an extremely rapidly changing field of study. Figure 13.14 Bruker BioTyper. (Courtesy of Bruker Daltonics.) Figure 13.15 (a) Vitek MS MALDI-ToF instrument. (b) Loading of specimen slide for analysis. (Courtesy of bioMérieux.) A 2009 study compared MALDI-ToF/MS (Bruker Daltonics, Bremen, Germany) versus conventional identification methods including the API ID 32C (bioMérieux) for identification of 267 clinical yeast isolates composed of Candida, Cryptococcus, Saccharomyces, Trichosporon, Geotrichum, Pichia, and Blastoschizomyces species subcultured on Sabouraud agar [187]. Utilizing the provided Biotyper software, MALDI-ToF identified 247 isolates (92.5%) on the first round compared to 244 isolates in the first round for the API ID 32C. Following the addition of complementary reference spectra to the database for the remaining isolates, MALDI-ToF was able to identify all 267/267 isolates while the API ID 32 C identified 256/267 isolates in the second round. Another study in 2009 found that MALDI-ToF/MS showed promise in identifying fluconazole resistance in C. albicans isolates by assaying the minimum concentration of fluconazole that induces a detectable mass spectrum profile change. Results correlated with the Clinical Laboratory Standards Institute (CLSI) methodology of minimum inhibitory concentration (MIC) by broth microdilution [185]. Further studies need to be conducted to better define the efficacy and usefulness of MALDI-ToF in this context. A 2010 study [308] compared MALDI-ToF/MS (Bruker Daltonics) to conventional techniques (Vitek2, API, and biochemical ) in the identification of 80 subcultured yeast isolates (19 retrospective and 61 prospective) covering seven genera and 12 species. In the prospective study, MALDI-ToF achieved 85.2% species identification and 96.7% correct genus identification compared to 77.0% species and 98.4% genus identification for routine biochemical methods. Misidentifications for MALDI-ToF were largely the result of an incomplete database that could be resolved with the addition of appropriate reference spectra. Stevenson et al. [288] conducted a study in 2010 where 192/194 (99%) of subcultured clinical yeast isolates were correctly identified using a Bruker MALDI-ToF with BioTyper software. One C. rugosa isolate (1/7) and one Cryptococcus neoformans isolate (1/3) could not be identified in this study. Low MALDI-ToF scores requiring repeat testing were observed commonly for isolates of C. guilliermondii, C. krusei, C. lusitaniae, and C. neoformans. Another 2010 study established a proof of concept study where various Candida species could be identified directly from blood cultures with appropriate sample preparation, thus allowing identification 1–2 days more quickly than if the isolates were subcultured [186]. Ferroni et al. [102] conducted a 2010 study in which the Bruker MALDI-ToF/MS system combined with the Andromas database (Andromas SAS) successfully identified 20/20 (100%) spiked blood cultures composed of 10 C. albicans isolates and 10 non-albicans Candida species in addition to 11/11 patient blood cultures containing C. albicans. Also in 2011, Bader et al. [13] analyzed 1192 clinical yeast and yeast-like isolates and compared two MALDI-ToF/MS platforms, the Bruker system/Biotyper database versus the AXIMA Assurance system/Saramis database (AnagnosTec/Shimadzu) along with traditional biochemical techniques (API 20 C AUX and ID 32 C systems from bioMérieux). With the Biotyper database, 97.6% of the 1192 isolates were correctly identified compared to 96.1% with the Saramis database and 96.9% for classic methods. The two MALDI platforms performed comparably and had lower rates of misclassification (Biotyper: 8 misclassifications, 21 unknown; Shimadzu: 2 misclassifications, 44 unknown) than classical techniques (30 misclassifications, 7 unknown). With supplementation of the MALDI databases, the rate of misidentifications and unknown assignments could be decreased. The MALDI-ToF/MS was much better at resolving C. orthopsilosis/metapsilosis/parapsilosis and C. glabrata/bracarensis than traditional biochemical approaches. Analysis with the Biotyper database required a protein extraction step while analysis using the Saramis database did not. Alanio et al. [2] constructed a MALDI database including the reference spectra of 28 clinically relevant species from seven Aspergillus sections comprised of five common and 23 unusual species. They then utilized the database to identify 140 previously characterized isolates (124 clinical and 16 environmental) following processing on a Microflex MALDI-ToF/MS spectrometer (Bruker Daltonics) with flex control software along with comparative data analysis using the Andromas SAS software. Out of the 140 samples, 127 were identified with one run, 11 were identified with two runs, and two samples could not be identified. Identified species included Aspergillus species from the Fumigati, Flavi, Terrei, Nigri, Nidulantes, Usti, and Circumdati sections. The two unidentified samples were ultimately identified as atypical Aspergillus fumigatus isolates (absence of conidiogenesis or atypical sporulation) by multilocus sequencing. With no misidentifications, MALDI was able to correctly identify 98.6% of tested isolates with 100% sensitivity utilizing the constructed database. A 2011 study [284] compared the MALDI Biotyper MALDI-ToF MS system (Bruker Daltonics) to conventional techniques directly from blood cultures with the utilization of a protein extraction step. MALDI-ToF correctly identified 95.9% of C. albicans and 86.5% of non-albicans Candida species. MALDI exhibited difficulty in the identification of C. guilliermondii, C. krusei, and C. lusitaniae isolates as well as polyfungal bloodstream infections. Dhiman et al. [68] conducted a 2011 study comparing a Bruker MALDI-ToF to traditional phenotypic methods using 138 common and 103 archived yeast strains. MALDI-ToF correctly identified 96.3% of common and 84.5% of uncommon isolates using a score threshold of 1.8. Unreliable identifications were noted for C. guilliermondii, Aureobasidium/Hormonema, C. neoformans, Blastoschizomyces capitatus, C. colliculosa, C. inconspicua, C. albidus, and Trichosporon asahii. Low identification scores for Cryptococcus neoformans were thought to result at least partially from the organism’s thick capsule, and it was noted that reference spectra for C. gattii were not in the database. McTaggart et al. [193] addressed the issue of identifying Cryptococcus species in a 2011 study that analyzed 137 Cryptococcus strains and 23 non-Cryptococcus strains subcultured on Inhibitory Mold Agar by MALDI-ToF using intergenic spacer DNA sequencing as the reference method. Following supplementation of the Biotyper 2.0.1 database with C. gattii reference spectra, 27/27 C. gattii isolates were correctly identified. Overall, all 137 isolates were correctly identified to species level. A single C. neoformans var. neoformans (1/4) isolate was misidentified to subspecies level as C. neoformans var. grubii. Another 2011 study conducted by Pinto et al. [251] utilized the Bruker Biotyper MALDI-ToF/MS system to retrospectively analyze 30 reference and 167 clinical isolates as well as prospectively analyzing 67 clinical isolates subcultured to Sabouraud’s dextrose agar in parallel with biochemical methods, where discrepancies were addressed by sequencing of the internal transcribed spacer region. MALDI-ToF/MS successfully identified 80% of the 30 isolates to species level. Out of 167 clinical isolates, 140/167 (84%) were identified to species level (threshold score > 2.0), and 160/167 (96%) were identified to genus level (threshold score > 1.70). Prospective analysis of 67 clinical isolates yielded successful species-level identification for 79% of isolates. Protein extraction of yeasts was found to be necessary for the generation of reliable mass spectra as compared to the direct colony preparation method. MALDI-ToF exhibited difficulty with identification of C. ciferrii, C. zeylanloides, C. nivariensis, C. bracarensis, Pichia kluyveri, Arxiozyma telluris, Debaryomyces carsonii, and Cryptococcus neoformans. Another study conducted in 2011 [324] investigated the Bruker MALDI Sepsityper kit (Bruker Daltonics) designed for the processing of blood cultures with a dedicated lysis step that disrupts red blood cells while leaving the bacterial and yeast cell walls intact. A total of 42 blood cultures containing yeast were isolated with correct identification of all samples. Serial dilutions of a C. parapsilosis culture revealed the limit of detection of this MALDI-ToF system is 5.9 × 105 colony-forming units (CFU). In 2011 Bille et al. [24] investigated the Bruker MALDI-ToF/MS system along with the Andromas SAS database for the identification of yeast and Aspergillus species. MALDI-ToF successfully identified 96.3% of yeast and 92.2% of Aspergillus species following a single acquisition, with identification of 98.8% of yeasts and 98.4% of Aspergillus species from the second acquisition. Difficulty was found with identification of C. lambica (not in database). Utilization of MALDI-ToF with the Andromas SAS database could successfully classify 63/64 morphologic Aspergillus species into Fumagati, Terrei, and Flavi sections. Another study in 2011 [43] utilized the Bruker MALDI-ToF/MS system and a variety of extraction protocols for the identification of mold isolates. The most effective method was to grow molds on Sabouraud–chloramphenicol–gentamicin agar and conduct a formic-acid-based extraction step following mechanical scraping. Utilizing this process, this group identified 87% (154/177) of isolates. Identification failed in 12% (21/177) of isolates due to the absence of reference spectra in the database. Appropriate reference spectra were lacking for Alternaria, Penicillium, Scedosporium, Paecilomyces, A. clavatus, A. melleus, A. oryzae, A. sydowi, A. alliaceus, Geotrichum, and Fusarium species. One Beauveria bassiana isolate was not identified, and one Rhizopus oryzae isolate was misidentified as Mucor circinelloides. MALDI-ToF/MS is also a promising technology for the identification of dermatophytes. Theel et al. [296] utilized the MicroFlex LT MALDI-ToF/MS system (Bruker Daltonics) to identify 100 well-characterized, archived dermatophyte isolates and 71 fresh dermatophyte cultures with either the supplied MALDI Biotyper library (MBL version 3.0) or a supplemented library containing an additional 20 dermatophyte species. Dermatophyte specimens were submerged in 70% ethanol, pelleted, and dried prior to suspension in 70% formic acid and 50% acetonitrile with subsequent matrix (HCCA, α-cyano-4-hydroxycinnamic acid) application and analysis. Out of 171 isolates, the provided Biotyper library identified 37.4% of isolates to the genus level and 20.5% to the species level while the supplemented database identified 93% of isolates to the genus level and 59.6% of organisms to the species level. It was also found that lowering cutoff score values to at least 1.5 for genus and 1.7 for species from the manufacturer-recommended values of 1.7 for genus and 2.0 for species increased the number of obtained identifications without increasing misidentifications. With the manufacturer-provided database, two isolates of Trichophyton mentagrophytes were misidentified as T. tonsurans while passing the 10% criterion for species identification. With the supplemented database, seven isolates of Trichophyton rubrum were misidentified as T. soudanense while passing the 10% criterion, however all T. rubrum isolates were correctly identified following deletion of the single T. soudanense reference spectrum from the supplemented database. A 2012 study [5] utilized the Andromas SAS software to identify 381 isolates of clinical dermatophytes and Neoscytalidium following culture on Sabouraud’s dextrose agar. Dermatophyte colonies were harvested in 70% formic acid and applied directly on a plate with subsequent matrix application. A reference database was constructed from a set of 50 reference strains from 12 clinically relevant species of dermatophytes and two species of Neoscytalidium. Utilizing MALDI-ToF/MS and the constructed database, 331/360 dermatophytes (91.9%) and 18/21 Neoscytalidium isolates (85.7%) were correctly identified. Two T. mentagrophytes var. interdigitale isolates were misidentified as either T. rubrum or T. tonsurans. In addition, spectral acquisition failed in 19% of Microsporum langeronii, 28% of T. soudanense, and 14% of Neoscytalidium isolates. Utilizing the Andromas SAS software, the authors were able to obtain higher levels of dermatophyte identification than observed results from a previous study utilizing the Bruker Biotyper database [296], and no colony extraction step was needed with the Andromas SAS software. Thus, with appropriate database reference spectra, MALDI-ToF/MS is a powerful method for the identification of dermatophytes. A 2012 study [280] compared the predecessor to the Vitek MS (bioMérieux, SA, Marcyl’Etoile, France), the AXIMA Assurance MALDI-ToF/MS system (Shimadzu Biotech) with Saramis database (AnagnosTec) to the API ID 32C system (bioMérieux) in identifying yeasts from dermatological patients. Sequencing of the internal transcribed spacer (ITS) regions of the ribosomal DNA was used for confirmation, and no extra protein extraction step was utilized. From a total of 83 isolates, MALDI-ToF results concurred with ITS sequencing in 94% of cases (78 isolates), while API ID 32C results concurred in 84.3% of cases. MALDI-ToF misidentified 1/35 isolates of C. albicans as C. glabrata and misidentified 1/10 C. glabrata isolates as C. tropicalis. One C. colliculosa isolate (1/1) was not identified by MALDI-ToF as there was no reference spectrum in the Saramis database. One Rhodotorula mucilaginosa isolate (1/1) and one Trichosporon mucoides isolate (1/1) were insufficiently identified to genus only. The API ID 32C was especially susceptible to the repeated misidentification of C. krusei isolates (5/7 isolates misidentified). In this study, MALDI-ToF/MS compared favorably to the API ID 32C system for the identification of dermatological yeasts. Iriart et al. [144] utilized the Vitek MS coupled with the “advanced spectra classifier” algorithm and version 1.0 of the MS-ID database for the identification of yeast-like and Aspergillus isolates compared to either the Vitek 2 (bioMérieux, St. Louis, MO) or routine laboratory identification techniques. Yeast-like isolates were applied directly from a Sabouraud agar plate to the Vitek MS disposable target with no protein extraction step. Following lysis with 25% formic acid and drying, α-cyano-4-hydroxycinnamic acid (CHCA) matrix was applied to the spot prior to analysis. For Aspergillus isolates, CHCA matrix was applied directly to the fungal spot under laminar flow with no protein extraction or formic acid lysis step. Out of 192 yeasts and yeast-like isolates, Vitek MS correctly identified 184 isolates (95.8%) to species compared to 183 isolates (95.3%) by routine laboratory tests and 169 (88.0%) by Vitek 2. For yeast organisms present in the database, Vitek MS failed to identify 1/60 (1.7%) of C. albicans isolates, 1/45 (2.2%) of C. parapsilosis isolates, and 2/2 (100%) of Geotrichum candidum isolates for an overall identification rate of 98.2%. In addition, Vitek MS failed to identify C. norvegensis and C. orthopsilosis isolates, as these reference spectra were not in the database. A single misidentification (major error) was made for an isolate of C. palmioleophila (not in database) being identified as C. haemulonii. Out of 44 Aspergillus isolates, Vitek MS correctly identified 36 (81.8%) and 39 (88.6%) were correctly identified by routine morphological identification. For Aspergillus species in the database, all A. fumigatus (33/33), A. flavus (2/2), and A. niger (1/1) isolates were properly identified. Isolates of A. sydowi (0/2), A. terreus (0/1), A. tubingensis (0/1), A. calidoustus (0/1), A. nidulans (0/2), and A. puniceus (0/1) could not be identified as these organisms were not in the Vitek MS database. The Vitek MS system allows for rapid high-quality identification of yeasts and Aspergillus species with only a single sample deposit and no protein extraction step. At the time of this writing, the Vitek MS system has received FDA approval for the identification of 193 clinical bacterial and yeast isolates. Continued supplementation of the provided MS-ID database and validation for additional organisms will be required to improve performance in the identification of clinically relevant fungal isolates. Overall, MALDI-ToF/MS represents a new and promising technology for the identification of clinically important fungal organisms. Results can be obtained within approximately 1 h of culture, and the assay requires minimal consumable resources and technical manipulation. It is important to obtain more comprehensive databases to fully utilize the potential of this technology. MALDI-ToF/MS will likely replace many of the current frontline biochemical techniques, however, conventional methods will be required for antibiotic susceptibility profiling for the foreseeable future. The MALDI-ToF/MS technology exhibits difficulty in mixed specimens thereby requiring subculturing of specimens containing multiple organisms. The Microscan Rapid Yeast Identification panel (Siemens Healthcare, West Sacramento, CA) is composed of chromogenic and modified conventional tests for identification of yeasts and yeast-like organisms, such as Prototheca spp., from clinical isolates. The 96-well plate format contains 27 dehydrated chromogenic substrates, which are designed to identify yeasts without the need for ancillary tests. A heavy suspension of a fresh yeast isolate, prepared in water and standardized to a known turbidity standard, is subsequently used to hydrate the plates. The plates are incubated for 4 h at 35–37°C before the reactions can be interpreted. The chromogenic readings can be made manually, read by eye, or with the Autoscan instrument. Interpretation of the biochemical profile occurs as the results are transformed into a nine-digit biotype number, which is compared with the Microscan cumulative database via the Autoscan instrument or manually, using the Microscan Rapid Yeast Biotype Codebook. The database identifies at least 42 yeasts and yeast-like organisms. In each case, profile identifications are assigned a cumulative relative probability of identification. When the probability of identification is not sufficient to confirm identification, supplemental testing of morphological and phenotypic characteristics is recommended. When the Rapid Yeast Identification Panel was compared to the API 20 C AUX system, early studies reported 94.1% correlation in identifying species of Candida, Hansenula, Pichia, Rhodotorula, and Saccharomyces when results were determined after 4 h. A lower correlation (65%) was observed for slower-growing species, such as Blastoschizomyces spp., Cryptococcus spp., Trichosporon spp., and yeast-like organisms, such as Prototheca spp. The overall correlation, using the first limited database was approximately 85% [171, 286]. The same system was also tested with results obtained after 72 h. The results were again compared to those with the Microscan Rapid Yeast Identification Panel at 4 h and the API 20 C AUX system with cornmeal-Tween 80 agar at 72 h. Both visual and Autoscan readings of the plates correctly identified 78% of the 357 yeast isolates tested with no supplementary tests required. With supplementary tests, Autoscan identified 96.6% (99.5% of common strains; 92.1% of less common strains, like C. glabrata, C. fumata, C. lusitaniae, C. lambica, C. rugosa, C. albidus, C. laurentii, and C. uniguttulatus), and the API 20 C AUX system identified 98.9%. Autoscan was shown to be reliable for the identification of common yeasts when supplementary tests were performed [286]. More recently, when the results were compared to those obtained with the API 20 C AUX system and from morphology on Tween-80 agar, the Microscan Walk-Away-96 correctly identified 67–82% of the 150 yeasts tested. The most commonly misidentified yeasts were C. tropicalis, C. glabrata, and C. parapsilosis [264]. The Vitek YBC system (bioMérieux) is an automated system for yeast identification. The system uses the Vitek test card, which is composed of microwells containing various identification substrates. The YBC is used for the yeasts that are most commonly encountered in hospitals. Yeast suspensions are prepared in sterile water. Tubes are placed in the Vitek filler-sealer, which uses a vacuum to load the liquid into the Vitek test card through a sterile straw. The sealer module removes the straw and seals the test card. The card contains dehydrated medium for a variety of biochemical tests. The reader–incubator unit maintains a controlled environment while the cards incubate; it rotates the cards so that readings can be measured by the laser unit and photodetector. The yeast database contains at least 36 species of commonly encountered yeasts. Results are maintained so that cumulative reports can be generated and can be formatted according to the user’s needs. Add-on software is available for purchase to maintain workflow and quality control data. The maximum capacity for the Vitek Junior model is 30 cards per run. The Vitek Senior comes in models that can analyze 60, 120, and 240 cards simultaneously. In an early study [76], the Vitek yeast database was compared to the API 20 C AUX system. The Vitek system compared favorably for the identification of common yeasts but less favorably for identifying others. For all yeasts identified, most of the identifications were made within 24 h of incubation. In another report [70], the YBC was compared to the API 20 C AUX system with additional biochemicals for the identification of 222 germ-tube-negative yeast isolates. The YBC identifications of commonly isolated yeasts compared favorably; however, the 48-h identifications of less common yeasts were not as successful: 55% were correctly identified, and the remaining isolates were either misidentified or not identified. In a separate study [126] the system was not able to identify an isolate of S. cerevisiae or C. tropicalis but provided correct identification for 98% of the common yeasts (n = 100). Similarly, the Vitek YBC, with 24–48-h results, was compared with the API 20 C AUX system and morphology on Tween-80 agar [263]. Of 150 yeast isolates, 85–95% were correctly identified with the Vitek YBC. The most common misidentified yeasts were C. tropicalis and C. glabrata. In another study [99], 409 germ-tube-negative yeasts and Geotrichum spp. were also tested. The results were compared with identification using the API 20 C AUX system and yeast morphology agars. Both systems identified most yeast isolates within 24 h. The YBC correctly identified 89.7% of 409 germ-tube-negative yeasts. The API 20 C AUX system correctly identified 99.3%. Of the remaining isolates, some profiles provided no identification of the organism, some profiles converted to misidentifications (the YBC incorrectly identified 7.3% of the isolates tested; the API 20 C AUX system misidentified only 0.7%). Results were comparable for C. glabrata, C. parapsilosis, and C. neoformans. The Vitek YBC had difficulty with C. tropicalis, C. krusei, Trichosporon, and some Cryptococcus spp. [97, 98, 100, 101]. A current instrument, the Vitek 2 system, allows identification of medically important yeasts and yeast-like organisms in 15 h by using a compendium of 47 biochemical reactions that are monitored by a sensitive fluorescence-based chemistry. The new database with 51 taxa was evaluated using the Vitek lD-YST card. Compared to the ID32C strip (bioMérieux) for 241 strains from 21 species, the Vitek 2 system correctly identified 92.1% of the isolates [120]. A second-generation yeast identification card (YST), the currently available card – converted from fluorescence-based to colorimetric chemistry, was introduced with the Vitek 2. The card has 64 wells and an expanded database of 53 species and 14 genera. There are several comparisons of the ID-YST and the new YST cards [9, 133, 177, 273, 304]. Loiez et al. [177] tested 172 strains representing 19 Taxa by the ID-YST, YST, and the API 32C system, which was considered the reference. The recorded concordance between the Vitek 2 cards and results with different identifications that could not be resolved by simple additional phenotypic testing were submitted for sequencing of the 18S–28S rDNA. Correct identifications to the species level were 93.6% for ID-YST and 83.7% for the YST card. A different outcome was reported in another 2006 publication [9]. Ninety-seven isolates from CHROMagar Candida were compared with the ID-YST, YST, and API 20C as the reference identification. Their results show a superiority of the YST over the ID-YST, with correct identifications of 94.8% and 83.5% respectively. A 2007 study [273] evaluated the YST card against the RapID Yeast plus (Remel) system. A total of 750 clinical isolates were tested by both systems and rRNA ITS region sequencing as the reference standard identification. The Vitek 2 YST card and the RapID correctly identified 98.2% and 95.5% isolates, respectively. The BD Phoenix Yeast ID Panel (Becton Dickinson, Sparks, MD) is a recently introduced panel for their Phoenix Microbiology System. It utilizes modified, conventional, fluorogenic, and chromogenic substrates for the identification within 4–18 h. Early studies demonstrate high accuracy compared to other systems. The reader should refer to recent publications for this relatively new system. In the YT Microplate test (Biolog, Hayward, CA), yeast cells are suspended in sterile water. The YT Microplate is configured with both of its carbon source oxidation tests using tetrazolium violet as the colorimetric indicator. Carbon-based assimilation tests are scored turbidimetrically and can only be read with the automated Biolog MicroStation. A few wells contain two carbon sources and a test for colorization of various carbon sources with D-xylose. Software support exists for the Biolog Yeast Database. In addition, the SF-N and SF-P Microplates can be used for metabolic testing of sporulating and filamentous microorganisms, such as the actinomycetes and fungi. Special culture medium is used to enhance sporulation, and a gel-forming colloid, which keeps the organisms more evenly suspended, is used to prepare the organism suspension. The SF Microplates are identical to the GN and GP microplates used for aerobic bacteria except they do not contain the tetrazolium redox dye, which is toxic to many of these species. The plates are incubated at 25–27°C for several days and read with the automated MicroStation. Sand et al. [272] compared the Biolog YT to the YBC and API 20 C AUX system with 171 germ-tube-negative yeasts; the Biolog system identified 48% of the isolates. The YBC and the API 20 C AUX system correctly identified 97% of the 171 strains tested. Both systems required repeat testing less than 10% of the time and required supplemental testing with 28% of the strains. With the Biolog system, there is a need for considerable expertise and a battery of supplemental reagents for correct identification of germ-tube-negative yeasts. The Microbial Identification System (MIS; Microbial ID, Inc., Newark, DE) relies on fatty acid analysis to identify yeast isolates. Cellular fatty acid chromatograms are analyzed and compared to the fatty acid profiles in the system’s database. Prior to analysis, yeast isolates must be subcultured, with subsequent saponification, methylation, and extraction of fatty acids. In one study using MIS (with the yeast clinical library version 3.8), 477 isolates and 23 different species of yeast were tested. The MIS generated results with a performance of 75% correct identifications compared to a combination of identification methods, including the Vitek YBC, morphology on cornmeal agar, and the API 20 C AUX system. Predictive values for different species ranged from 47 to 100%, depending on the species [155]. In another similar study [60], the MIS was compared to the API 20 C AUX system and conventional methods. The results indicated that 374 (68%; n = 550) yeasts were correctly identified to the species level. After repeat testing, 18 more were identified, bringing the overall correct identification to 71.3%. Of all yeasts tested, 15.8% were incorrectly identified and 16.2% were given no identification. The isolates tested represented a variety of species: C. albicans (294), C. glabrata (145), C. tropicalis (58), C. parapsilosis (33), and other yeasts (20). Candida glabrata was the most commonly misidentified yeast, and it was misidentified as S. cerevisiae 32.4% of the time. Another study performed with the MIS provided evidence that the results can be dependent on the supplier of the agar on which the yeast isolates are grown [156]. Because of the slow growth of dimorphic fungi and the potential difficulty and safety risks involved in their confirmatory identification, molecular identification techniques are extremely useful for their detection. Nucleic acid hybridization tests are based on the complementary alignment and binding of nucleic acid strands to form stable double-stranded complexes. The AccuProbe System (Gen-Probe, San Diego, CA) uses a single-stranded DNA probe with a chemiluminescent acridinium label as the complement of the rRNA of the target organism. Lysates of an organism are prepared by sonication with glass beads and heat treatment. The rRNA is released from the organism, and the labeled DNA subsequently binds with the target organism rRNA to form a stable labeled DNA-RNA hybrid, the presence of which can be detected in the Gen-Probe luminometer. The selection reagent, provided in the kit, provides for differentiation of hybridized and nonhybridized probes during readings taken by the luminometer. Positive reactions are based on cutoff values of relative light units derived from the chemiluminescence. FDA-approved kits for in vitro diagnostic use are available for the identification of Coccidioides immitis, Histoplasma capsulatum, and Blastomyces dermatitis. Molds can be tested from growth in both liquid and solid media; however, the assay is not reliable for formalin-killed cultures of C. immitis [123].
Commercial Methods for Identification and Susceptibility Testing of Fungi
13.1 Direct examination and detection methods
13.1.1 Cryptococcus spp.
Characteristic
C. neoformans var. grubii
C. neoformans var. neoformans
C. gattii
Serotype (s)
A
D
B, C
Main geographic distribution
Worldwide (major agent in AIDS patients)
Northern Europe
Tropical and subtropical; also in British Columbia and the Pacific Northwest United States
CGB medium
No growth
No growth
Growth
Immunoassay method
Serotype sensitivity (ng CrAg/mL)
A
B
C
D
Immy LFA Strip
1
1
9
8
Immy Latex
28
47
380
62
Meridian CALAS Latex
19
37
940
54
Crypto-LA latex
38
64
1600
50
Meridian Premier ELISA
28
23
>2000
770
13.1.1.1 Latex agglutination tests
13.1.1.2 Enzyme immunoassays
13.1.1.3 Lateral flow assay
13.1.1.4 Comparative studies
13.1.2 Candida spp.
13.1.3 Aspergillus
13.1.3.1 Platelia Aspergillus EIA
13.1.3.2 Cross-reactivity
13.1.4 1,3-β-D-glucan
13.1.5 Endemic mycoses
13.2 Culture and detection
13.2.1 Fungal blood cultures
13.2.2 Manual systems
Assay
Method
Comparison
n
Sensitivity (%)
Comments
Reference
Isolator
Compared system
Isolator 10
Lysis
centrifugation
Bactec HBV
93
Isolator provides better detection of H. capsulatum; HBV better for C. glabrata
321
Bactec 26B
86
Isolator superior for H. capsulatum, C. glabrata, others
321
Bactec 9240 with MYCO/F lytic bottle
24
79.2
70.8
Isolator provides advantage for C. neoformans
312
BacT/Alert
22
100
Improved TTD with Isolator; H. capsulatum and C. neoformans from Isolator only
181
BacT/Alert
22
68.2
181
BacT/Alert for C. neoformans
53
88.7
43.4
Shorter TTD with Isolator; both systems costly
23
BacT/Alert for H. capsulatum
18
100
22.2
Shorter TTD with Isolator, both systems costly
23
ESP 80A (VersaTREK)
72
85
56
Isolator superior; detected significantly more Candida spp.
31,54
Septi-Chek
72
85
57
Isolator superior; detected significantly more Candida spp.
54
Conventional broth methods
20 (true positive)
70
90
False negatives in 30% (6 of 20) with Isolator vs. 20% with broth method
211
42 (false positive)
97.6
2.4
Routine use of Isolator was not advocated
211
Isolator 1.5
Bactec NR660 Aerobic 6A
89
85.4
82
Comparable systems for Candida spp. in pediatric population; similar TTDs
236
13.2.3 Continuously monitored automated blood-culture systems
Instrument
Bottle type
Media
Specimen type
Maximum volume (mL)
BacT/ALERT
Standard aerobic (SA)
40 mL suppl TSB
Blood or normally sterile body fluid
10
Standard anaerobic (SN)
40 mL suppl TSB
Blood or normally sterile body fluid
10
FAN aerobic (FA)
30 mL peptone-enriched TSB, supplemented with BHI solids and activated charcoal
Blood or normally sterile body fluid
10
FAN anaerobic (FN)
40 mL peptone-enriched TSB, supplemented with BHI solids and activated charcoal
Blood or normally sterile body fluid
10
Pediatric FAN (PF)
20 mL peptone-enriched TSB, supplemented with BHI solids and activated charcoal
Blood
4
BACTEC
Standard/10 aerobic/F
Enriched soybean–casein digest broth
Blood
10
Standard anaerobic/F
Pre-reduced enriched soybean–casein digest broth
Blood
10
Plus aerobic/F
Enriched soybean–casein digest broth with cationic resin
Blood
10
Plus anaerobic/F
Pre-reduced enriched soybean–casein digest broth with cationic resin
Blood
10
Lytic/10 anaerobic/F
Pre-reduced enriched soybean–casein digest broth
Blood
10
Peds plus/F
Enriched soybean–casein digest broth with cationic resin
Blood
3
Mycosis-IC/F
40 mL BHI broth, soybean–casein digest broth
Blood
10
Myco/F lytic
40 mL supplemented 7H9 and brain heart infusion broth
Blood and body fluids
10
VersaTREK
REDOX 1 EZ Draw, aerobic with stir bar
40 mL
Blood and sterile body fluids
5
REDOX 2 EZ Draw, anaerobic
40 mL
Blood and sterile body fluids
5
REDOX 1, aerobic with stir bar
80 mL
Blood and sterile body fluids
10
REDOX 2, anaerobic
80 mL
Blood and sterile body fluids
10
13.2.3.1 BacT/Alert 3D
13.2.3.2 BACTEC
13.2.3.3 VersaTREK (ESP) System
13.3 Identification systems
13.3.1 Media for isolation and identification
13.3.1.1 Bismuth sulfite glucose glycine yeast agar
13.3.1.2 Eosin methylene blue agar
13.3.2 Rapid identification and screens
13.3.2.1 Identification of unique enzymes
13.3.2.2 Methods for identification of C. glabrata
13.3.2.2.1 Rapid trehalose
13.3.2.3 Identification of C. dubliniensis
13.3.2.4 Identification of C. neoformans and C. gattii
13.3.3 Commercial kits for identification of yeasts and yeast-like organisms
13.3.3.1 Uni-Yeast-Tek
13.3.3.2 API 20 C AUX
13.3.3.3 RapID Yeast Plus
13.3.4 Automated systems
13.3.4.1 MALDI-ToF/MS
13.3.4.2 MALDI biotyper
13.3.4.3 Vitek MS
13.3.4.4 Rapid yeast identification panel
13.3.4.5 Vitek YBC/Vitek 2 YST
13.3.4.6 BD Phoenix Yeast ID Panel
13.3.4.7 Biolog YT Microplate
13.3.4.8 Microbial Identification System
13.4 Molecular testing
13.4.1 Accuprobe
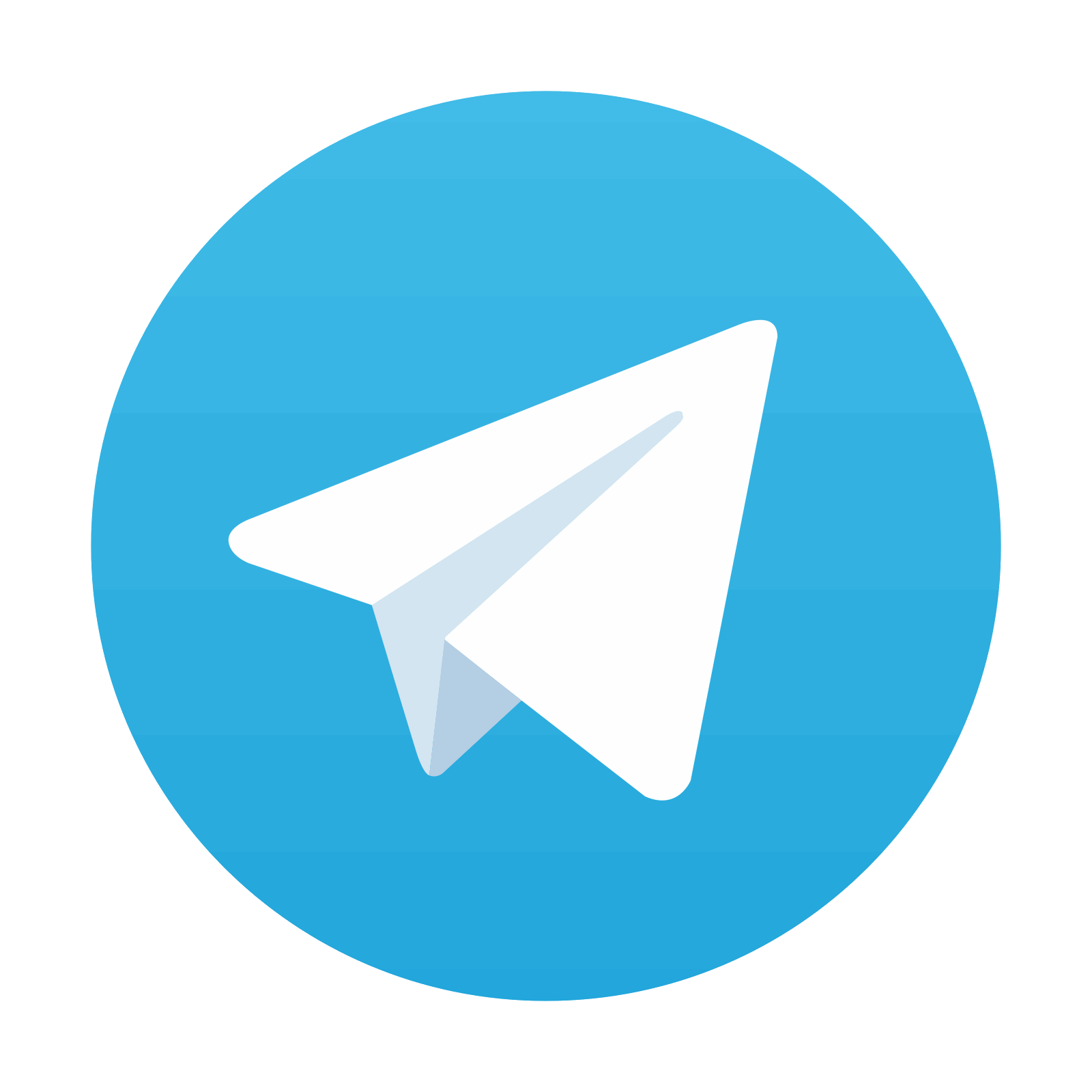
Stay updated, free articles. Join our Telegram channel

Full access? Get Clinical Tree
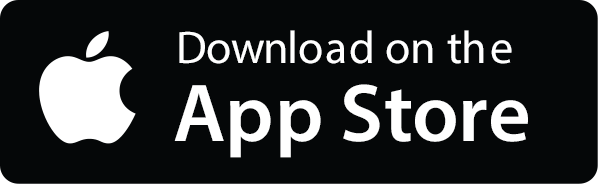
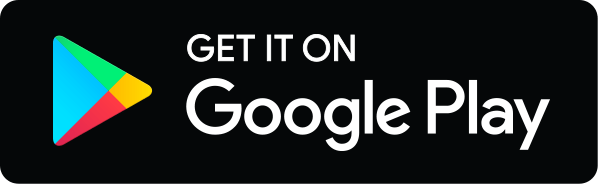