CHAPTER 11 Natalie Williams-Bouyer, Donald H. Bouyer, and Michael J. Loeffelholz Department of Pathology, University of Texas Medical Branch, Galveston, TX, USA Tick-borne diseases (TBDs) are increasing in prevalence and represent a growing occurrence of arthropod-borne infectious diseases. Possible reasons for this phenomenon include shifts in the distribution of animal reservoirs and tick vectors, as well as more human presence in areas where there is increased contact with ticks and their associated pathogens. From a public health point of view, the complex interactions of ticks, pathogens, hosts, and the environments involved in this changing pattern of disease are not completely understood [14, 49, 50, 58, 59]. However, the reporting of these illnesses and diseases to health department agencies allows for the gathering of information and statistics, in support of establishing trends related to these illnesses [11]. Tick-borne illnesses occur in distinct geographic locations, which is a key factor in determining specific tick-borne infections to be considered in diagnosis. Patients with TBD often present with nonspecific symptoms, including various rashes or skin lesions, which are often accompanied by fever and influenza-like symptoms [4]. Travel history, recent locations, and potential for tick bite are important. However, individuals are usually unaware of tick exposure and less than 50% present with a positive history of a tick bite [12, 26, 33]. Nonetheless, the absence of a tick-bite history should not exclude a diagnosis given the correct clinical presentation. Severe illness and mortality can occur, if there are delays in diagnosis and treatment. Since the organisms transmitted by ticks are infrequently encountered in clinical specimens, diagnosis of tick-borne diseases can pose a challenge in that the etiologic agents of these illnesses can be both difficult and hazardous to culture in laboratories. Therefore, clinical specimens for culture, molecular analysis, and some serologic assays are commonly referred for diagnosis at specialized laboratories utilizing specific testing methodologies [4]. This chapter discusses the most common agents associated with TBD and details the testing methodologies currently available for laboratory diagnosis. Ticks are excellent vectors for disease transmission, with more than 800 recognized species [7, 27]. These arthropods, of the class Arachnida, are second only to mosquitoes in worldwide transmission as vectors of human disease. Of the recognized families of ticks, only hard ticks (Ixodidae) and soft ticks (Argadidae) are of medical importance. TBD are transmitted by tick vectors such as the deer tick, dog tick, wood tick, and lone star tick [12]. In the United States, ticks are the most common vectors of vector-borne diseases. Ticks can carry and transmit a vast number of pathogens, such as bacteria (including rickettsiae), spirochetes, protozoa, viruses, nematodes, and toxins. A single tick bite can transmit multiple pathogens, which can also lead to atypical presentations of classic tick-borne diseases [27]. Species of Rickettsia comprise a genera of small, obligately intracellular Gram-negative bacteria within the family Rickettsiaceae. The genus is divided by their phylogenetic clustering into the spotted fever group (SFG) and typhus group (TG). The SFG contains bacteria that are generally recognized as human pathogens, including R. rickettsii, R. conorii, and R. africae, which are respectively the causative agents of Rocky Mountain spotted fever (RMSF), Mediterranean spotted fever (also referred to as boutonneuse fever) and African tick-borne fever [2, 12, 85, 89]. The TG consists of only two members, namely R. prowazekii and R. typhi. As part of their life cycle, Rickettsia spp. reside in an arthropod host, during which they are maintained by transovarian transmission and/or cycles and horizontally transmitted to vertebrate hosts. It is important to note that the SFG organisms are transmitted by ticks, whereas those of the TG are transmitted by fleas, lice, and mites [8, 49, 64]. Due to the TBD focus of this chapter, only rickettsial diseases transmitted by ticks will be further described. RMSF can become a life-threatening illness for previously healthy individuals. Approximately 500 cases of RMSF are documented annually, with Dermacentor andersoni and Dermacentor variabilis being the most common vectors for this disease [17, 82]. In the United States, these infections occur from April through October. An average of 7 days after arthropod bite inoculation of R. rickettsii, patients with RMSF develop fever, severe headache, malaise, and myalgia, frequently accompanied by nausea, vomiting, and abdominal pain, and sometimes cough [12, 32, 40]. The major diagnostic sign of infection is a characteristic maculopapular rash that typically appears on the palms and soles of 83–90% of infected patients, following 3–5 days of illness [32]. The organism infects endothelial cells, frequently leading to increased vascular permeability and focal hemorrhages. In severe cases, noncardiogenic pulmonary edema and rickettsial encephalitis with coma and seizures are grave conditions that often presage death [37, 84]. Rickettsia parkeri, transmitted by Amblyomma maculatum (Gulf Coast tick), occurs in the southeastern region of the United States. Rickettsia parkeri causes a mild illness at the tick inoculation site eschar, fever, headache, myalgia, usually a maculopapular or vesiculopapular rash, less frequently tender regional lymphadenitis, and no reported deaths [17, 61, 63]. In southern Europe, Rhipicephalus sanguineus is the primary tick vector of R. conorii, which causes Mediterranean spotted fever. Disease is usually a benign self-limited exanthematous febrile illness, however, it can have a fatal outcome in patients who are elderly or have underlying diseases or other risk factors [2, 29, 71]. Fatal cases more frequently have acute renal failure, hyperbilirubinemia, obtundation, tachypnea, petechial rash, gastrointestinal symptoms, and coagulopathy [18]. African tick bite fever (ATBF) is broadly distributed across most of the African continent. The disease pathogen, R. africae, has been detected in Amblyomma spp. ticks [2]. Of note for the epidemiology of ATBF is the acquisition of the disease by North American tourists, hunters, or deployed military staff returning from areas where R. africae is endemic [31, 52, 60]. The disease begins approximately 5–7 days following tick bite, with sudden onset of fever, fatigue, headache, and myalgia. Inoculation eschars are reported inconsistently and are identified in roughly 50–100% of cases. However, the occurrence of multiple eschars has been reported to frequently occur. Additional clinical manifestations include regional lymphadenopathy and generalized maculopapular or papulovesicular rash. No cases of fatal disease resulting from these bacteria have been reported, although more severe manifestations, including myocarditis and neuropathy, are sometimes described [28, 63]. For most clinical microbiology laboratories, assays for antibodies to rickettsiae are the only tests performed. These assays are utilized principally for serologic confirmation of the diagnosis in convalescence. Such testing is not useful in situations of life threatening, or acutely incapacitating, rickettsial disease. For serologic diagnosis, blood is collected as early in the course of disease as possible, a second sample is collected after 1 or 2 weeks, and if a fourfold rise in antibodies has not occurred, a third sample is collected 3 or 4 weeks after onset. The serum may be stored for several days at 4°C but should be stored frozen at −20°C or lower for longer periods to avoid degradation of the antibodies. Serologic assays focus on the indirect immunofluorescence assay (IFA). Other approaches include indirect immunoperoxidase assay, latex agglutination, enzyme immunoassay (EIA), line blot, Western immunoblotting, and rapid lateral flow assays [35, 38–42, 70, 83]. Only some of these assays are available as commercial kits or developed for use in reference laboratories. The IFA contains all the rickettsial heat-labile protein antigens and group-shared lipopolysaccharide antigen and, thus, provides group-reactive serology. The IFA reagents are available commercially for SFG and TG rickettsiae from Scimedx Corp., Denville, NJ; Focus Technologies, Cypress, CA; Fuller Laboratories, Fullerton, CA. In cases of RMSF, IFA detects antibodies at a titer of ≥ 64, usually in the second week of illness. For boutonneuse fever, an IFA titer of ≥ 40 occurs in 46% of patients between days 5 and 9 of illness, in 90% of patients between 20 and 29 days, and in 100% of patients thereafter [12]. Indirect immunoperoxidase assays for boutonneuse fever (research-use only, RUO) and presumably other rickettsioses yield results similar to those of IFA when the IgG diagnostic titer is set at 128 and that of IgM is set at 32 [42]. Advantages include the use of a light microscope rather than a fluorescence microscope and the production of a permanent slide result. Latex agglutination test reagents are available commercially from Scimedx Corp.: only for R. rickettsii in the United States. Latex beads coated with an extracted rickettsial protein–carbohydrate complex containing rickettsial lipopolysaccharide are agglutinated mainly by IgM antibodies, with reports of a sensitivity of 71–94% and a specificity of 96–99% [35]. A diagnostic titer of 128 is often detected early in the second week of illness. The EIAs have been developed in various formats, including antigens coating microtiter wells or immobilized on nitrocellulose or other sheets for use in the commercial reference laboratory setting. Dot-EIA kits are commercially available in the United States from Scimedx Corp., for detecting antibodies against R. rickettsia and R. conorii. Standard EIAs for detecting IgG or IgM antibodies against SFG rickettsiae are also available from Panbio Diagnostics (Brisbane, Australia) internationally, but are not currently available for purchase in the United States. The utilization of these tests for paired sera from populations with clinical (fever, headache, or rash) and epidemiologic (vector exposure) features consistent with rickettsiosis would most likely yield useful information. The most useful methods for establishing a diagnosis during the acute stage of illness when therapeutic decisions are critical include immunohistochemical and molecular diagnostic testing. Such testing is presently available in only a few reference laboratories. The diagnoses of RMSF, R. parkeri infection, boutonneuse fever, and African tick-bite fever, have been established by immunohistochemical detection of rickettsiae in formalin-fixed, paraffin-embedded sections of biopsy specimens of rash and eschar lesions [37, 40, 83]. Monoclonal antibodies that are specific for lipopolysaccharides of SFG rickettsiae have been used to detect rickettsiae by immunohistochemical staining. These antibiodies are commercially available through Fuller Laboratories (Fullerton, CA, USA). The sensitivity and specificity of immunohistochemical detection of R. rickettsii in cutaneous biopsy specimens are 70 and 100%, respectively. Eschar biopsies yield sensitive specimens for the diagnosis of SFG rickettsioses [40, 83]. Polymerase chain reaction (PCR) has been applied to the amplification of the DNA of R. rickettsii, R. parkeri, R. conorii, R. prowazekii, R. africae, and other species. This methodology provides a higher diagnostic yield when applied to biopsy specimens of rickettsia-infected lesions [18, 43]. If whole blood, plasma, buffy coat, or tissue cannot be processed for PCR within several days, it should be stored at −20°C or lower. Serum has a lower sensitivity for PCR, but is often diagnostic in fatal cases [48, 58]. Nested PCR applied to skin biopsy specimens, particularly of eschars prior to treatment, has a sensitivity of 78% [30]. For all pathogenic Rickettsia spp., the 17 kDa lipoprotein gene is a target. The gltA, rrs, groEL, ompA, and ompB genes have also been amplified diagnostically, with the Rickettsia being identified through either restriction fragment length polymorphism analysis using AluI and XbaI or sequencing of the PCR product. Recent advances in technology such as real time PCR allow for increased sensitivity in the detection of rickettsiae, during the early or acute phase of disease before the development of antibody [55, 69, 72, 77, 86]. Lyme disease or Lyme borreliosis (LB) is a multisystem disease, and is the most common vector-borne disease in the United States, with an annual incidence of 20–100/100,000. Cases occur throughout the year, with peak incidence occurring in the summer. The highest incidence of disease in the United States occurs in the northeastern and midwestern states [11, 34]. Borrelia burgdorferi sensu lato, the Gram-negative spirochete that causes this disease, is divided into three separate species, which are pathogenic in humans: B. burgdorferi sensu stricto (United States and Europe), B. garinii, and B. afzelii (Europe and Japan). Lyme borreliosis usually presents with fever, headache, malaise, fatigue, and weight loss. There are three stages of clinical Lyme disease (primary, secondary, and late persistent), although patients do not necessarily progress through all stages [6, 16, 87]. Early localized disease occurs 3–30 days after a tick bite. Approximately 60% of patients develop erythema migrans, which is a skin lesion at the site of the Ixodes tick bite and is the best clinical marker of Lyme disease [16, 87]. The lesion begins as a macule, which expands to form an annular erythema with partial central clearing. Involvement of the heart, joints, and central nervous system may occur in the late stages of disease. Arthritis of the knees is the most common long-term disease manifestation [6, 53, 88]. The Centers for Disease Control and Prevention (CDC), Infectious Diseases Society of America (IDSA), and the Public Health Agency of Canada recommend serologic antibody testing as the initial diagnostic test of choice for Lyme disease [1, 11, 88]. However, such testing should be reserved for patients with a clinical picture highly suggestive of Lyme disease, as it can have a poor positive predictive value even in endemic areas. Both steps can be done using the same blood sample. The first step is a screening enzyme immunoassay (EIA) or indirect fluorescent-antibody (IFA), which is followed by confirmatory Western immunoblot testing for positive or equivocal EIA or IFA result [19, 57]. Both commercial and reference laboratory assays are available for this purpose. For an up-to-date listing of US FDA-cleared assays, refer to http://www.accessdata.fda.gov/scripts/cdrh/cfdocs/cfPMN/pmn.cfm; type “LSR” at “Product Code” prompt. The EIA assays incorporate the testing of serum for the presence of antibodies reacting to whole-cell sonicates of low-passage B. burgdorferi antigens immobilized to a solid surface. Some commercial assays incorporate variations to the antigen preparation through utilization of the addition of recombinant proteins or purified antigens (Vidas Lyme IgG and IgM EIA, bioMérieux, Durham, NC; ImmunoWELL Borrelia (Lyme), GenBio, San Diego, CA; PreVue B. burgdorferi, Chembio Diagnostics Systems, Stoneybrook, NY) and synthetic C6 peptide, derived from the VlsE protein of B. burgdorferi (Immunetics, Inc., Boston, MA). Outside the United States, Borrelia burgdorferi IgG and IgM enzyme-linked immunosorbent assay (ELISA) test kits are available through Immunolab GMBH, Kassel, Germany. Western blot analysis detects B. burgdorferi IgM and IgG antibodies. Amongst commercial sources of these Western blot tests are Marblots (Trinity Biotech, Jonestown, NY) and Virablots (Viramed Biotech, Oceanside, CA) [19, 53, 88]. It should be noted that variability of B. burgdorferi antigen and stage of disease may affect serological results [1, 16]. Serologic testing lacks sensitivity in early Lyme disease, as IgM antibodies typically do not develop until 3–4 weeks after infection onset. The sensitivity of IgM blots is approximately 40% in acute phase, and 60–80% during convalescence. Sensitivity increases for patients with disseminated disease [19]. If a patient with suspected early Lyme disease has a negative single serology, serologic evidence of infection is best obtained by testing of paired acute- and convalescent-phase serum samples. IgG antibodies develop at 6–8 weeks following illness onset. Therefore, serum samples from persons with disseminated or late-stage Lyme disease almost always have a strong IgG response to B. burgdorferi antigens [34, 88]. PCR testing is recognized by the IDSA as having the highest sensitivity for Lyme disease in synovial fluid samples from patients with untreated late Lyme arthritis, as well as those with untreated neurologic disease [56, 87]. Tick-borne relapsing fever (TBRF) is a spirochete disease caused by at least 16 different Borrelia spp. and is present worldwide [7, 27]. The vector is the soft-bodied argasid tick of the Ornithodoros genus, of which rodents are commonly infected [7, 75]. Those found in the United States occur in the western mountains and high deserts and plains of the southwest, with fewer than 30 cases diagnosed annually [22]. In the United States there are two forms of relapsing fever: epidemic louse-borne disease caused by B. recurrentis and the endemic tick-borne form caused by various organisms including B. hermsii, B. turicatae, and B. parkeri. Louse-borne relapsing fever occurs under conditions of poor hygiene, crowding and poverty, where organisms are maintained by passage between lice and humans. TBRF is transmitted via the saliva during the attachment of ticks. Clinically, in both types of relapsing fever, after an incubation period of 2–15 days, there is a massive spirochetemia accompanied by high fever (usually above 39°C), headaches, myalgias, arthralgias, nausea, chills, and weakness. Jaundice occurs in 7% of patients, and the case fatality rate is 2–5% [7]. The initial febrile episode usually ends suddenly and patients defervesce after 3 to 7 days, when an immune response is mounted and the spirochetemia disappears. Several days to weeks later, the disease can recur with a less severe course. The relapses are due to variation of surface antigens of the organism, resulting in temporary evasion of the immune system [7, 22, 27]. A definitive diagnosis is made by blood smear examination during a febrile period. Direct examination by dark-field microscopy or staining of blood specimens with Giemsa or Wright’s stain may be performed to detect Borrelia spp. Either method provides a sensitivity of 70%. The organisms can usually be seen in the blood from patients with relapsing fever, but not from those with Lyme disease. The Warthin–Starry silver stain or monoclonal antibodies can be used to stain tissues. Nucleic acid amplification techniques have high sensitivity when used with tissue specimens. Therefore, skin biopsy specimens, from the periphery of the erythema are recommended. Cultures of joint fluid are recommended for patients with arthritic symptoms. The medium of choice is Barbour–Stoenner–Kelly broth (Sigma, St. Louis, MO) and the culture should be maintained in a microaerophilic environment at 30–33°C for 4–6 weeks. While useful in detection of TBRF causing borreliae, culture has low sensitivity for diagnosis of B. burgdorferi associated with Lyme disease. Serologic assays for TBRF are not readily available, and diagnostic value of these assays is limited due to antigenic variation shown by etiologic borreliae agents [1, 7, 22, 27, 75]. Tularemia is caused by Francisella tularensis, a small aerobic and pleomorphic Gram-negative coccobacillus that has worldwide distribution, and can be transmitted by organism inhalation, direct inoculation, or by tick vectors. In the United States, cases of tularemia have been reported in all states, except Hawaii, with most occurring during the summer months [25, 36, 66]. Tick-borne cases of tularemia are known to be spread by three tick species: A. americanum, Dermacentor andersoni, and Dermacentor variabilis. Francisella tularensis is a highly potent human pathogen. Irrespective of acquisition by inhalation or inoculation of skin or mucous membranes, the infective dose may be as low as 10 organisms. Tularemia is an acute febrile invasive disease characterized pathogenically by rapid intracellular replication into high bacterial densities, accumulation of phagocytes, and extensive tissue necrosis. The clinical presentation of this infection is abrupt, with fever, chills, headache, and generalized pain. There are several distinct clinical forms including ulceroglandular, glandular, oculoglandular, oropharyngeal, pneumonic, and typhoidal. The most common form of presentation in the United States is the ulceroglandular form, resulting from tick bites and contact with infected animals (e.g., squirrels, rabbits, rats, and other rodents) [25]. Following an incubation period of 3–10 days, a papule forms at the site of infection, eventually ulcerates, and is accompanied by regional lymphadenopathy [15]. Due to the rapid development of the infection, successful treatment of disease is dependent on early clinical suspicion and initiation of appropriate antibiotics. In general, mortality may be associated with 2% of cases, even with antibiotic treatment [36]. In routine clinical work, culture of specimens in tularemia-suspected cases is infrequently undertaken due to F. tularensis being fastidious and relatively slow growing. However, F. tularensis grows favorably on cysteine supplemented agar media such as enriched chocolate agar, cysteine heart agar with 9% heated sheep red blood cells (CHAB) agar, or GC Agar II with 1% hemoglobin and 1% isovitalex, with cultures incubated in a 5% CO2
Rickettsiae and Tick-borne Diseases
11.1 Introduction
11.2 Overview of tick-borne diseases
11.2.1 Ticks as vectors for infectious disease transmission
11.2.2 Rickettsial infections
11.2.2.1 Epidemiology and clinical manifestations
11.2.2.2 Laboratory diagnosis and diagnostic challenges
11.2.3 Other tick-borne diseases
11.2.3.1 Bacterial agents
11.2.3.1.1 Lyme disease
Epidemiology and clinical manifestations
Diagnosis and diagnostic challenges
11.2.3.1.2 Tick-borne relapsing fever
Epidemiology and clinical manifestations
Diagnosis and diagnostic challenges
11.2.3.1.3 Tularemia
Epidemiology and clinical manifestations
Diagnosis and diagnostic challenges
Stay updated, free articles. Join our Telegram channel

Full access? Get Clinical Tree
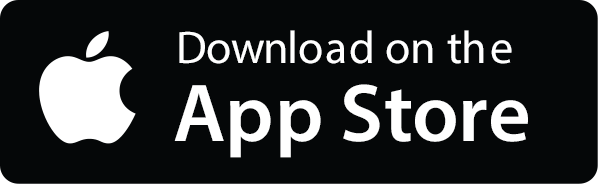
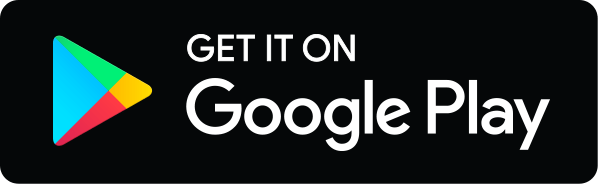