SARS-CoV-2 Transmission
From the beginning, the major role of airborne transmission was highlighted for severe acute respiratory syndrome coronavirus 2 (SARS-CoV-2) infection.1 In addition, contact with contaminated surfaces was considered a potential source of contagion. Indeed, studies reporting the different times of viral particle survival as infectious on distinct materials were published.2 However, over time, it became clear that inhalation of infectious particles through aerosols is by far the major route of infection.
Resembling what is well established for other respiratory viral infections, such as influenza, the size of the inoculum determines in SARS-CoV-2 infection both the risk of contagion and the chances of developing severe disease.3 This observation was made during the first wave of COVID-19 comparing outbreaks in different settings (e.g., chorus singers in close spaces). In Madrid, Spain, we reported several clusters of infected people, some of them developing severe pneumonia and others mostly remaining asymptomatic or developing mild disease.3 The estimated airborne viral inoculum was the major determinant of this discordant outcome, with an indoor meeting associated with severe disease and living in a large home with a garden linked to mild infection, as shown in Fig. 4.1.3
A unique feature of SARS-CoV-2 compared to other life-threatening coronaviruses such as severe acute respiratory syndrome (SARS) or Middle East respiratory syndrome (MERS) is that infected persons may transmit the virus before they begin symptoms, during the incubation period. In this way, preventive strategies such as isolation are often implemented too late, when contacts already have been exposed unnoticed.4
Vaccines
Vaccines are very effective biologic agents that prevent infections, save lives, and reduce global mortality. The incidence of vaccine-preventable diseases among children has decreased enormously during the last five decades in developed countries. Four vaccine-preventable diseases were eradicated by 2015: smallpox, poliomyelitis, rubella, and congenital rubella syndrome.5 Other important achievements regarding vaccination during this period involved at least three major advances. First, the administration of the first recombinant vaccine, the hepatitis B vaccine, was found to reduce the infection rates and the liver cancer risk.5 Second, the license of the first polysaccharide-protein conjugate vaccine decreased the incidence of invasive Haemophilus influenzae type B in children.5 Third, the first vaccine was designed for people in low-income countries as the vaccine for Neisseria meningitides group A.6
Effective vaccines should stimulate the immune system and elicit adaptive and long-lasting immunity to a particular infectious disease. Adaptive immunity is exerted by T- and B-lymphocytes that induce memory cells and amplify the response to a later infection with the microorganism. B-lymphocytes will produce antibodies that might neutralize the pathogen, while T-lymphocytes are effector cells that will destroy those cells infected with the infectious agent. However, vaccines should induce efficiently not only adaptive but also innate immune responses. In the past, innate immunity was considered to induce nonspecific immune responses throughout phagocytic cells such as monocytes, macrophages, or dendritic cells, which recognize pathogens throughout pattern recognition receptors and lack immunologic memory. However, more recently, it was discovered that innate immune cells presented a heterologous memory of past infections, a process known as trained immunity that involves specificity.7
There are two main requirements to design prophylactic vaccines. First, a target antigen that elicits a strong immune response and correlates with protection is needed. Classical antigens used in vaccine designs are either main virulence factors or relevant factors involved in the entry of a pathogen into cells. The second requirement implies a vaccine platform that allows the antigen expression and allows a safe and convenient administration while generating an adequate prophylactic immune response. While the target antigen differs in each pathogen, vaccine platforms might be common to a great variety of pathogens. In this regard, vaccine platforms prepared with small molecules such as DNA, mRNA, recombinant proteins, peptides, polysaccharides, nanoparticles, or cellular vaccines as dendritic cells loaded with small molecules, filled into the category of flexible vaccine platforms. In this regard, we would like to stress the pioneering work of Robert Malone in the late 1980s.8,9
Vaccine formulations based on small molecules, except cellular dendritic-based vaccines, require adjuvants to help to amplify the immune response, improve the immunogenicity of the antigens or elicit a preferred immune response. For instance, adjuvants can attract the antigen-presenting cells to the site of vaccination, increase the generation of memory immune cells or improve the generation of neutralizing antibodies or effector T cells. Very few adjuvants are licensed for human use since the approval of aluminum salts in 1920 that induce Th2-immune responses.10,11 Live attenuated or inactivated microorganisms are other types of vaccines composed of whole pathogens and do not require adjuvants. Vaccine formulations that do not include whole microorganisms in their design should also include adjuvants.12 Finally, vaccine formulations also include preservatives in their compositions such as formaldehyde, thimerosal, phenol, polyethilenglycol, and stabilizers such as sugars, gelatin, proteins as human albumin, and hydrolyzed collagen.10,11
Once the vaccine formulation is ready, several parameters are explored before the approval of a vaccine for commercialization, such as toxicity, immunogenicity, and efficacy. These parameters are examined first in preclinical trials using experimental animals and in vitro human testing and second in human trials after authorization by the Food and Drug regulatory agencies. Human trials for vaccine approval involve three phases.13
Phase 1
It examines the induction of fatal toxicities or severe pathologies. It is performed in 20 to 100 recruited healthy volunteers. This phase usually takes around three months.
Phase 2
It explores the immune response against the infectious agent, the vaccine doses, and the vaccine protocol. For this phase, randomized 200 to 500 volunteers are recruited. Volunteers should be adults, between 18 and 55 years, healthy, that they have not had the infection. The election of the groups depends on the risk groups for each infectious disease. Half of the volunteers are inoculated with the complete vaccine and half of them with placebo, a vaccine formulation without the target antigen of the pathogen. Volunteers should live together with the pathogen to evaluate if the vaccine can protect against the infection. Phase 2 can last at least 6 months.
Phase 3
It has the purpose of evaluating long-lasting immune responses. In this stage, thousands of volunteers are recruited, usually between 30,000 and 50,000 persons, with the same features and groups as the previous phase. That is healthy individuals that did not have the infection. Since this phase includes a high number of volunteers, the results of this phase have a great statistical value to detect secondary adverse effects that were not detected in previous phases. These toxicities are always mild and not severe. This phase also measures vaccine efficacy. A normal vaccine efficiency is at least 60%. This phase usually lasts between 9 and 12 months.
Which Clinical Effects are Explored with the Analysis of Toxicity, Immunogenicity, and Efficacy?
The toxicity explores any severe or fatal adverse effects of the vaccine design. The most common severe adverse effects associated with vaccines are caused by an inflammation elicited that is accompanied by the release of proinflammatory cytokines that evokes an acute-phase response involving the production of IL-6, C-reactive protein (CRP), fibrinogen, or TNF.14 The entry of these acute-phase cytokines into the bloodstream might trigger fever or malaise. Since vaccines are administrated by intramuscular injection, they might also cause some local trauma and small pain that does not last longer than a week but, in some cases, might be longer. Another type of severe adverse effects is the induction of autoimmune diseases in rare cases as the cases of systemic lupus erythematosus detected in some adolescent girls after HPV vaccination or the Guillain-Barré syndrome, a neurological complication immune-mediated, a rare event observed six weeks after a dose of tetanus, oral polio, rabies or influenza containing vaccines.15,16 In fact, autoimmune diseases can be explained by the molecular mimicry of the antigen and the production of autoantibodies. Some viruses such as Epstein-Barr (EBV), cytomegalovirus (CMV), human immunodeficiency virus (HIV), influenza (HIN1) or human T lymphotropic virus (HTLV-1) are examples that might induce autoimmune diseases, and SARS-CoV-2 infection can also trigger autoantibodies.17 Also, some components of the vaccine formulation such as adjuvants that induce innate immunity and are required to augment the immune response or other ingredients such as preservatives or stabilizers might cause some toxicities or some autoimmune associated reactions.18
The immunogenicity is based on the ability of an antigen or antigens to induce the adaptive immune system, formed by T-lymphocytes, both CD4+ and CD8+ T cells, as well as B-lymphocytes.19 To measure the vaccine immunogenicity specific antibodies in the blood are measured. If vaccines induced a good immune memory, a large increase in the antibody levels occurs after the application of a booster dose of the vaccine. But not only do antibodies measure the immunogenicity of a vaccine, but vaccines also induce the expansion of CD4+ and CD8+ T cells specific for the vaccine antigen, and therefore, antigen-specific T cells are examined to determine if a vaccine induces a strong cellular immune response.
The efficacy is measured as a percentage of the reduction in disease between individuals vaccinated and control participants, known as the placebo group, and performed in optimal conditions. Therefore, it is a measurement of the protection attributable to a vaccine. However, vaccine efficacy does not always predict their effectiveness, known as the protection attributable to a vaccine administered under field conditions. For instance, vaccines for older age groups may implement methods to improve their immunogenicity, including the use of adjuvants. Therefore, prospective studies of vaccine effectiveness in real scenarios are needed for adjustments.20
Vaccines for Coronaviruses
Coronaviruses are a group of viruses of the Coronaviridae family that demonstrate spike glycoproteins on the nucleocapsid envelope, which are necessary to attach to host cells.21 Coronavirus has a positive-sense single-stranded RNA genome and a nucleocapsid with helicoid symmetry. They infect mammals and birds and caused respiratory tract and diarrhea symptoms. Coronavirus infections in humans can be lethal as the cases of SARS or MERS.
After the SARS epidemic in 2002–2003, several laboratories performed vaccine developments to prevent this lethal disease whose symptoms are high fever, shortness of breath, and pneumonia. The origin was southern China, with more than 8096 cases and 774 deaths that involved 26 countries. No vaccines were commercially available, and SARS cases ceased from 2004. Most subunit vaccines targeted the spike (S) glycoprotein as the main virulence factor used by the virus to bind and enter host cells. Vaccines inducing strong immune responses against S protein would affect the entry of the virus to host cells. Vaccines prepared for SARS used different technologies, mainly live-attenuated or inactivated virus, recombinant viral vectors, DNA, virus-like particles (VLPs), and soluble proteins, but all of them were studied in preclinical studies, none of these vaccines passed to clinical trials.22
Live attenuated or inactivated viral vaccines imply first to transform the virus into a non-replicating virus, and therefore, the virus infectivity is reduced either deleting components of the virus genome or using physical-chemical methods such as heat or formaldehyde. Recombinant viral vectors prepared as SARS vaccines used a different virus able of host cell infection that has been genetically engineered to express components of SARS virus. VLPs are non-infectious multiprotein structures from other virus proteins that self-assemble into virus-like particles (Table 4.1).22 Human clinical trials of phase I were only performed with vaccines that used inactivated SARS, DNA, or soluble proteins based on S glycoprotein.22 To be able to examine protection with these vaccines from virus infection and clinical signs, vaccinated individuals should be exposed to the virus. However, it should be noted that SARS has high virulence, and challenge studies were not performed in humans, and consequently, protection efficacy was not assessed.
Adapted from Padron-Regalado (2020).
MERS coronavirus emerged in 2012. Similar to SARS vaccines, most of the subunit vaccines were targeted to S glycoprotein. Vaccines based on live attenuated viruses, recombinant viral vectors, nanoparticles, DNA, and soluble proteins were tested in experimental animal models (Table 4.2).22 Only a DNA-based vaccine went into a clinical trial at phase I and viral replication vaccines using modified Ankara virus MVA.23
Current COVID-19 Vaccine Overview
Since the formal declaration by WHO of a global pandemic on 11 March 2020 due to a SARS-CoV-2 that caused the coronavirus disease (COVID-19), a year later, over 3 million deaths had been reported in the world. There has been a race to develop safe and effective vaccines in a scale and manner never reported before. More than 200 potential vaccines are using different approaches and platforms. Genome sequencing and structural analyses revealed that this positive-sense single-stranded RNA encodes for structural proteins spike (S), envelope (E), membrane (M), and nucleocapsid (N), as well as several accessory and non-structural proteins. The virion shape is giving by M and N proteins, while the spikes on the viral surface that confers a solar corona appearance are provided by the S protein.24 S protein forms a homotrimeric glycoprotein that binds to the functional receptor human for angiotensin-converting enzyme 2 (ACE2). Spike protein binding to ACE2 is responsible for the virus entry into target cells via the receptor binding domain (RBD).25 The S protein exists in a prefusion conformation that undergoes major structural rearrangements to fuse the viral membrane with the host cell membrane. The RBD performs conformational movements that hide or expose receptor binding determinants, implying two states, the down and inaccessible state and the up and receptor-accessible state. These studies also revealed key epitopes, amino acids A475 and F486, as the binding sites for neutralizing antibodies.26,27 Therefore, S protein and the RBD appeared as the primary targets to block viral entry and the focus of most vaccine developments, therapeutic antibodies, and diagnostics.
Immune responses to SARS-CoV-2 is characterized by the following issues that condition the designs of vaccines:
Induction of Innate Immunity
Innate immunity is triggered via pattern associated molecular patterns (PAMPS) expressed by the virus, such as RNA that stimulates multiple immune pathways such as Toll-like receptors (TLR) 3 and 7, cytosolic retinoic acid-inducible gene 1 (RIG-1), and melanoma differentiation associated protein 5 (MDA5).28 Local tissue damage in the lungs released damage molecular patterns (DAMPS) that induce local inflammation. These inflammatory responses provide anti-viral immunity after activating type I and III IFN-1 pathways that upregulate certain acute phase cytokines such as IL-6 or IL-1βa nd recruit neutrophils and other innate immune cells that elicit anti-SARS-CoV-2 adaptive memory T and B cells.29 Uncontrolled inflammation in COVID-19 patients described as the “cytokine storm” causes tissue damage with inflammatory cell infiltration. Acute respiratory distress syndrome is characteristic of the severe manifestations of COVID-19.30 Patients with severe disease demonstrate high levels of IL-6, IL-2R, IL-10, and TNF-α while having low levels of IFN.29 Patients requiring ICU admission show high levels of IL-2, IL-7, IL-10, G-CSF, MCP-1, TNF-α.30,31 Vaccine design should avoid the production of this cytokine storm while inducing IFN regulatory cytokines.
Production of SARS-CoV-2 Specific Neutralizing Antibodies
Activated B cells produce antibodies that play several roles as anti-viral immunity, viral neutralization, antibody-dependent cellular cytotoxicity (ADCC), and antibody-dependent cellular phagocytosis, and antibody-dependent complement activation.32 While the generation of high levels of neutralizing antibodies would be important for a successful human vaccine; COVID-19 patients can recover without producing high levels of neutralizing antibodies.33 In this regard, the IgG memory B cells to S protein and RBD observed in COVID-19 patients are essential for a rapid recall response to further contact with the virus. Some COVID-19 patients lose neutralizing antibodies rapidly; however, other patients maintained high levels even 3–4 months post-infection.32
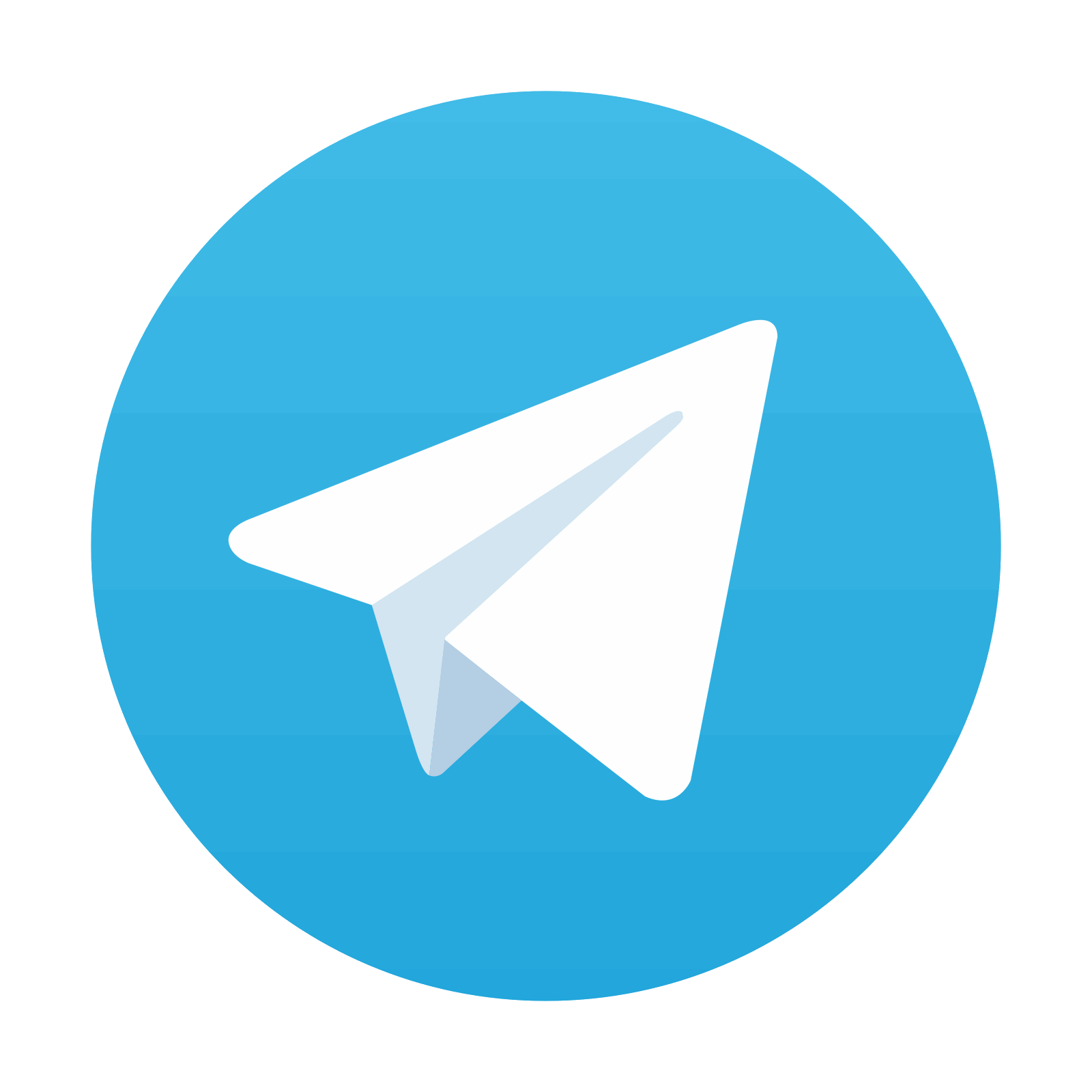
Stay updated, free articles. Join our Telegram channel

Full access? Get Clinical Tree
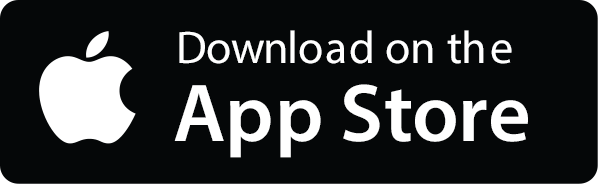
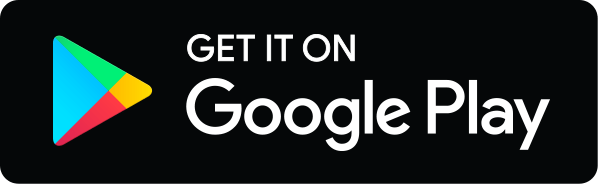