Introduction
The COVID-19 pandemic, which originated in Wuhan, China, likely in December 2019, has caused devastation to global human health as well as incalculable socioeconomic harms and burdens. The world faced multiple waves of infection as well as several predominant variants with seemingly increasing contagiousness. While the majority of infected individuals either are asymptomatic or experience a self-limiting viral illness, many will progress to a severity of disease requiring visits to urgent care centers and emergency departments; although the proportion of these patients who progress further and require hospitalization has been relatively low with respect to overall infections, it was enough to overwhelm entire hospital systems during waves and local surges.
While the case fatality rate of COVID-19 is low, this number rises significantly among those who are hospitalized.1 The quality of hospital care has proven to be extremely impactful on survival—the original “supportive care only” and “early intubation” strategies recommended by public health agencies and professional medical societies resulted in unprecedentedly high inpatient mortality rates as well as ventilator dependence rates and lengths of stay. Conversely, when improved treatment protocols emerged, particularly those centered around corticosteroids, mortality rates improved markedly.
During peak community infection rates, hospitals experienced severe staffing shortages and personal protective equipment (PPE) shortages, as well as bed and equipment shortages, requiring everything from interstate staffing, to recycling N95 masks, to splitting ventilators, and other extreme measures. Hospital staff members suffered extreme physical, psychological, emotional, and spiritual hardship, as mass amounts of rapid clinical deterioration and death surrounded them, and they simultaneously feared for their own well-being and the safety of their household members. Fortunately, as the medical and scientific community accumulated increasing knowledge and understanding about the transmission dynamics of severe acute respiratory syndrome coronavirus 2 (SARS-COV-2) and pathophysiology of COVID-19, this disease became quite manageable. Sound, evidence-based hospital practice is therefore critical in reducing morbidity and mortality of patients and preserving the safety and well-being of hospital staff. Here, we will review an evidence-based approach to the hospitalized patient with COVID-19.
Evaluation and Diagnosis
Thorough history taking is the cornerstone of diagnosing COVID-19 infection and primarily categorizing the patients upon initial presentation according to the severity of their illness. Clinicians should balance the need for a thorough history with the importance of avoiding unnecessarily prolonged exposure. The patient should be wearing a surgical or well-fitted mask during the interview and exam. Proper hand sanitizing, donning and doffing of protective equipment, and proper N95 respirator fit test and use are also critical in order to protect the clinician, colleagues, patients, and the community. A clinician may consider obtaining a portion of the history by calling into the room and discussing by telephone or video telemedicine, and limiting the in-person encounter to the physical examination and possibly key portions of the history, if the necessary setup is in place and the patient is capable of participating.
A focused history needs to be meticulously obtained with attention to recent travel, contact with suspicious or confirmed cases of COVID-19, and the duration of symptoms, if any. Wide ranges of symptoms have been identified with COVID-19 infection, ranging from asymptomatic to severe multiorgan failure. Common generalized symptoms include fatigue, muscle and body aches, fever and/or chills, and malaise. Upper respiratory symptoms vary between dry and/or productive cough, shortness of breath, rhinorrhea, and sore throat with or without respiratory distress. Approximately 15% of patients will present with purely gastrointestinal (GI) symptoms including anorexia, nausea, vomiting, diarrhea, and abdominal pain. Neurological symptoms include headaches, anosmia/dysgeusia, and confusion. Less frequently, acute lower motor neuropathies such as facial palsy and Guillain–Barré syndrome have been causally linked to COVID-19 infection. Onset of symptoms has ranged between 2 days and 2 weeks after confirmed exposure to SARS-CoV-2. A study in Wuhan, China, reported that the mean incubation period was 5.1 days and that 97.5% of individuals who developed symptoms did so within 11.5 days of being infected.2
Detailed past medical history must be obtained; underlying lung diseases, diabetes, hypertension, chronic corticosteroids, or immunosuppressant usage may predispose patients to severe COVID-19 pneumonia and can worsen the outcome. A meta-analysis of eight studies including 46,248 infected patients found that the most prevalent comorbidities were hypertension and diabetes, followed by cardiovascular diseases and respiratory system disease.3 Aggressive treatment from the beginning of the onset should be considered for these patients. Relevant social history needs to be addressed; patients of low socioeconomic classes living in overcrowded households must be isolated and other members of their families tested for possible infection.
It is of utmost importance that patients with suspected and/or confirmed cases of COVID-19 be reported to the local or state health department. Caution should be exercised while caring for suspected/confirmed cases; Centers for Disease Control and Prevention (CDC) guidelines call for practicing airborne and contact precautions with such cases. Airborne and contact precautions must be practiced with all patients with suspected/confirmed diagnoses as per the CDC to protect health care providers and limit the spread of the virus. Physical examination of COVID-19 patients should include proper general physical, cardiopulmonary, neurological, and other systems examination as relevant and should ideally be done in isolated designated areas within the health care facilities. Similar to history taking, a clinician must find a proper balance between a sufficiently thorough examination and avoidance of unnecessarily prolonged exposure.
Clinicians should carefully evaluate vital signs and any deviations from normal range or concerning trends. Fever, tachycardia, tachypnea, and reduction in oxygen saturation (SpO2) are common.
In performing a general examination, the clinician should assess whether the patient appears distressed or toxic. Other findings may transiently appear normal—the clinician must be able to recognize impending deterioration, as it occurs rapidly and is difficult to mitigate once it becomes severe, whereas patients will respond much more favorably to prompt treatment.
Examination of the head, ears, eyes, nose, and throat should assess for signs of sinusitis as well as pharyngitis. Extraocular muscle examination is important as patients are at risk of stroke. Examination of mucosal membranes may reveal dehydration and need for resuscitative fluids. Examination of the neck should assess for tracheal tugging as well as lymphadenopathy. A careful cardiac examination may detect tachycardia, arrhythmia, or signs of heart failure. Patients are also at risk for myocardial infarction and myocarditis.
Pulmonary examination poses a challenge, as lung auscultation has limited concordance with severity of lung injury in COVID-19. Course crackles and dullness to percussion could indicate a secondary bacterial lobar pneumonia. Clinicians must recognize signs of hypoxia, such as increased work of breathing, accessory muscle use, and cyanosis. In some cases, the phenomenon of “happy hypoxia” has been described, in which patients, despite low oxygen saturation and/or low PaO2, do not experience respiratory distress and do not seem to experience sequelae such as end-organ ischemic injury.
Examination of the extremities and skin may reveal rashes or decreased skin turgor in the case of dehydration. Edema can be a manifestation of edema. The extremities should be evaluated for edema as patients are at risk for pathologies that can cause right heart failure (left heart failure, pulmonary embolism, and pulmonary vasoconstriction). The clinician should also assess for signs of vascular occlusive disease, such as decreased pulse, decreased skin temperature, and decreased sensation, as well as small vessel vaso-occlusive distal skin lesions (“COVID toes”).
Neurological examination is highly important, as a patient in respiratory failure who may otherwise respond well to noninvasive respiratory support may become confused or encephalopathic and require airway protection. A full neurological examination is important as patients are at risk for cerebral ischemia as well as inflammatory neuropathies.
Laboratory tests to detect the viral nucleic acids or antigens are obtained on initial presentation to confirm the viral infection using nucleic acid amplification tests (NAATs) such as real-time reverse transcription–polymerase chain reaction test of a nasopharyngeal specimen obtained with a swab. Immunoassay tests (antigen tests) may also be used, yet with less sensitivity than most NAATs.4
Arterial blood gases (ABG) test should be obtained to characterize the patients’ oxygen partial pressure in the blood and the degree of hypoxia that may necessitate different types of assisted ventilation. In addition, ABG test will enable physicians to calculate the A-a gradient, which provides an insight into the extent of alveolar damage. Inflammatory markers including C-reactive protein (CRP), D-dimer, lactate dehydrogenase, and ferritin levels can play a role in both diagnostic and prognostic workup. Observing the trend of these inflammatory markers is helpful in either reinforcing or modifying the treatment plan. Rising acute-phase reactants may indicate the need for increased corticosteroid dosing. Markedly rising fibrin split product may indicate the need to transition from prophylactic to intermediate or full anticoagulation, although the literature has been mixed.
Complete blood count can reveal leucopenia, leukocytosis, and lymphopenia. According to a meta-analysis of 3,062 COVID-19 patients, normal leucocyte count, lymphopenia, and elevated levels of CRP and erythrocyte sedimentation rate were found to be the most prevalent laboratory findings in these patients.5
Hepatic injury is common, and liver transaminases along with bilirubin should be monitored. Marked elevations in transaminases, hypertriglyceridemia, and hyperferritinemia suggest the development of hemophagocytic lymphohistiocytic syndrome/macrophage activation syndrome. Of 417 hospitalized patients, over 75% were found to have abnormal liver tests and 21.5% had hepatic injury, according to a study by Bai et al.6 Bai et al concluded that patients with abnormal liver tests were at a significantly increased odds of developing severe pneumonia.
Acute kidney injury in COVID-19 patients may be causally related to the direct damage of the renal tubules by the virus or as a result of the heightened inflammatory response by the immune response. Monitoring renal function and applying renal supportive measures can prevent lifelong renal damage to these patients.7 If the patient presents with abdominal pain, nausea, vomiting, or diarrhea, obtaining a lipase level should be considered, as the patient could be experiencing acute pancreatitis.
Due to the nonspecificity of findings on chest imaging and to minimize the risk of airborne transmission of the virus in fixed radiography or computed tomography (CT) scanner rooms, the CDC does not recommend chest X-ray or CT to diagnose COVID-19 infection. In addition, the current American College of Radiology (ACR) Appropriateness Criteria statement on Acute Respiratory Illness states that chest CT is “usually not appropriate.”8 The ACR recommends frugal use of CT chest, only for hospitalized, symptomatic patients with specific clinical indications for CT and stresses on the importance of practicing proper infection control in order to limit any potential transmission of infection.9
COVID-19 pneumonia presents with several possible changes in the CT chest: ground-glass opacities with consolidation and infiltrates affecting (commonly) both lungs, which may have a peripheral distribution. Compared with non-COVID-19 pneumonia, COVID-19 pneumonia was less likely to have a central and peripheral distribution, pleural effusion, or lymphadenopathy.6
A 12-lead electrocardiogram should be performed to assess for any arrhythmia, ischemia, conduction disturbance, or sign of right ventricular strain, which, in turn, could raise suspicion of pulmonary embolism.
Once admitted to the hospital, complete blood counts, metabolic profile, hepatic profile, and acute-phase reactants should be monitored closely. Electrolytes must be repleted appropriately, anemia must be evaluated, and the etiology identified. Patients may be at risk for GI bleeding as well as microangiopathy. Hemodilution from aggressive hydration must also be considered. Platelet count must also be evaluated and monitored. It has been proposed that the viral spike protein may bind to the CD147 receptor expressed on the surface of leukocytes and erythrocytes, which in turn behaves as a platelet activator, and may result in a “catch-and-clump” mechanism of intravascular thrombosis.10 Also, the use of heparin products, along with other pharmacological agents, may be associated with thrombocytopenia. Offending agents should be discontinued or changed when possible.
Management
A number of nonpharmacologic (high-flow oxygen support, noninvasive ventilatory support, permissive hypoxia, and awake prone positioning) and pharmacologic interventions have been utilized with mixed results in terms of outcomes data. Pharmacologic modalities have included antiviral therapy (remdesivir), targeted immunomodulatory therapy (tocilizumab), passive immunization therapy (convalescent plasma, monoclonal antibodies), antithrombotic therapy (heparin, direct anticoagulants, aspirin [ASA]), and micronutrient supplementation (ascorbic acid, zinc, cholecalciferol, thiamine) as well as repurposed use of various medications with putative angioprotective, immunomodulatory, and/or antiviral activity (statins, hydroxychloroquine [HCQ], ivermectin, colchicine, famotidine, melatonin). However, the pharmacologic modality universally recognized to have resulted in a marked reduction in hospital mortality, and therefore emerged as global standard of care for hospitalized patients with COVID-19 pneumonia, is corticosteroid therapy.
Patients requiring hospital admission are likely experiencing the pulmonary–inflammatory phases of COVID-19. At this stage, little to no viral replication persists, and the patient is experiencing a maladaptive host response to the presence of RNA viral material, which is highly reactogenic. The most common form of lung injury appears to be organizing pneumonia, whereas chest radiography consistent with viral pneumonia is less frequent.11 Additionally, lung biopsy findings have not been consistent with an invasive viral disease, with few viral cytopathic changes noted.
In addition to parenchymal and interstitial lung involvement, patients experience a multifaceted vascular disease as well. This includes a vasculitic component, a microangiopathic thrombotic component, and a pulmonary vasoconstrictive component, likely driven by excessive serotonergic activity driven by activated platelets.
In order to counteract these mechanisms of disease, various pharmacological interventions have been included in hospital protocols.
As with any patient presenting with bacterial or viral sepsis, airway, breathing and circulation (ABC) must be assessed and patients must be triaged, stabilized, and appropriately dispositioned. If the patient is suitable for the medical wards and does not require step-down or intensive care, he or she should be placed on telemetry and admitted to an appropriate COVID-19 treatment unit. Large-bore intravenous (IV) access should be obtained in the emergency department, appropriate IV crystalloid should be administered, and oxygen therapy should be titrated ideally to SpO2 92% or higher; however, if a patient with lower oxygen saturation is comfortable and tolerating high-flow oxygen, increasing to more advanced modalities, especially endotracheal intubation and invasive ventilation, should be avoided, as these modalities may increase transpulmonary pressures and pulmonary inflammation, in addition to increasing intrathoracic pressure and impairing venous return, whereas end-organ injury has not been observed among patients with “happy hypoxia.”
Nonpharmacological
When patients progress to more severe states of illness despite basic supportive measures, a number of nonpharmacological techniques can be applied to mitigate clinical deterioration. In patients with severe acute respiratory distress syndrome (ARDS) supported by invasive mechanical ventilation (MV), prone positioning is considered standard management. However, prior to the COVID-19 pandemic, proning was not considered usual practice for nonintubated awake individuals in respiratory failure. The applicability of proning to both MV and nonventilated patients has yielded positive results with improved oxygenation. In addition, prone positioning has been observed in nonintubated patients.
Prone positioning results in physiological and anatomic modifications that decrease ventilation and perfusion mismatch, thus improving oxygenation. In addition, pronation of patients facilitates improved expansion of posterior lung segments, resulting in improvement of airway secretions, decreases in alveolar collapse, and improvement of atelectasis. The prone positioning may potentially decrease lung injury. Typically, a six-member team is required for proning. Typical proning teams consists of critical care nurses, respiratory therapists, physicians, and physical therapists. Most patients during the COVID-19 pandemic spend 16 to 18 hours prone and then 6 to 8 hours supine. In one small study, involving 25 patients undergoing awake proning, over 75% patients (19 patients) demonstrated an improvement in oxygenation, with oxygen saturation levels above 95%; thus, they did not need to be intubated, at least immediately. Of those 19 patients, only 7 subsequently required intubation.12 There was a decrease in the immediate need to proceed to MV in many patients. In those patients who did not respond to proning, 83% required MV.12 During proning, expansion of the anterior chest wall is limited. This results in increased uniformity of chest wall compliance. The gravitational changes induced by proning improve alveolar recruitment in the posterior lung zones, allowing for an increased number of alveoli to engage in gas exchange. In both awake nonintubated patients and those on MV, changes in diaphragmatic excursion result in equalization of stress dynamics, thereby attenuating lung injury and improving recruitability in posterior lung zones and thus relieving atelectatic changes.13 Furthermore, the posterior lung zone is highly perfused and thus recruitment of alveoli in this zone will result in improved V/Q matching.13
The presence of the SARS-CoV-2 in patients who develop severe COVID-19 disease generates a dysregulated immune response, resulting in hypercytokinemia, capillary leak migration of inflammatory cells, monocytes, and polymorphonuclear leukocytes. This occurs both in the lung and systemically. The pulmonary manifestations are alveolar injury, organizing pneumonia, and ARDS response. As the inflammations escalate, disruption of the alveolar capillary units occurs and progressive severe hypoxemic respiratory failure ensues. The maximization of oxygen delivery via by ancillary therapies such as high-flow nasal cannula (HFNC) and noninvasive ventilation has proven to be beneficial in many patients who have not undergone invasive MV. The overall goal is to safely delay or abate MV as long as possible.
During the pandemic, HFNC, which is able to provide warm humidified concentrations of 21 to 100% oxygen, was used with increasing frequency. HFNC is able to deliver flow rates of up to 60 L/min and provide some degree of PEEP. The benefits of this mode of oxygenation are potential for improvement in mucous clearance and prevention of atelectasis. In the management of hypoxemic respiratory failure, HFNC can be employed as a bridge to MV or in the prevention of early intubation. Although the use of this ancillary method of oxygenation did not decrease mortality, it was effective in decreasing intensive care unit (ICU) length of stay and increased ventilator-free days.14,15
There has been a concern for dispersion of SARS-CoV-2 particles or airborne transmission from patients with noninvasive positive-pressure ventilation (NIPPV) devices. The risk of viral transmission with NIPPV can be significantly reduced with the use of a filter on the expiratory circuit and the automatic measurement and quantification of a leak at the interface (which allows prompt leak correction and reduction of virus dispersion).
NIPPV includes continuous positive airway pressure and bilevel positive airway pressure. In the initial management of severe hypoxemic respiratory failure not immediately requiring endotracheal intubation and MV, several representative bodies such as the Society of Critical Care Medicine, the National Institutes of Health (NIH), and the European Society of Intensive Care Medicine as well as the World Health Organization (WHO) recommend the use of HFNC over NIPPV. In settings where HFNC is unavailable, NIPPV should be employed with close monitoring of respiratory and oxygenation status.16–18
A recent analysis of the HOPE COVID-19 registry demonstrated that in patients with respiratory failure 20% were managed with NIPPV, with 50% of these patients not ever requiring MV.19 Failure of NIPPV in preventing MV was associated with a poor prognosis and increased mortality. Thus, NIPPV with close monitoring of patient is a viable strategy in preserving resource utilization and reducing the need for MV.19 Noninvasive ventilation may represent a viable strategy particularly in cases of overcrowded and limited intensive care resources in this setting, but prompt identification of those failing is mandatory to avoid harm.19
Recommendations and guidelines vary on the use of NIPPV, as currently there is insufficient evidence regarding true effectiveness of NIPPV in acute viral pneumonia with hypoxia. However, we have enough data to support clinically guided use of NIPPV in some patients in order to abate and thus reduce the need for MV.
It is pertinent to note that patients on HFNC and NIPPV due to possible encumbrance of using the oropharyngeal route of nutrition can go days without oral intake and are at risk for the development of malnutrition. The increased catabolic state due to infection easily throws off the patients’ metabolism. Oral diet or enteral nutrition (EN) in patients with pre-existing GI conditions (e.g., inflammatory bowel disease) has been the preferred route for nutrition to allow patients to benefit from gut nutrition. Nasogastric tube insertion and any other nutrition access procedures should be done as soon as possible in eligible patients to avoid further malnutrition. Switching to parenteral nutrition can be considered in patients intolerant to EN and in more critical cases.20
The psychological stress on both hospitalized critical and noncritical COVID-19 patients and their families adds further hindrance to the successful treatment of patients. The social distancing, isolation, and sense of impending doom are all contributing factors to patients’ anxiety and worsening psychological stress. Hence, implementing psychological support in the patients’ treatment regimen is essential. Continued social support from relatives and friends can be achieved through texts, phone, and video calls and should be encouraged.21 When it comes to end-of-life care and more severe states of illness, policies should be flexible and allow families the proper autonomy to make informed risk-based decisions, as opposed to potentially depriving them from seeing their loved ones again. Proper protective measures should be maintained, and family members should be encouraged to isolate, if feasible, and to follow all state and local public health guidelines to avoid promoting community spread after a hospital visit. If there is very high local community transmission, a more conservative and restrictive approach may be warranted. These matters should be the subject of debate and discussion involving patient advocacy service, ethics committee members, infection control officers, nursing management, and hospital upper management.
Pharmacologic Therapy
Corticosteroid Therapy
The pathologic hallmarks of COVID-19 are a dysregulated hyperinflammatory state, endothelial activation, and injury with ensuing coagulopathy.22,23 The pathogenesis of COVID-19 illness appears to be induced by dysregulated systemic and pulmonary inflammation, along with endothelial injury, hypercoagulability, and thrombosis.22,23 Clinical evidence and autopsy studies evaluating the pulmonary parenchyma and vasculature demonstrated the presence of microvascular and macrovascular thrombosis.22–24 In part, recent evidence supports that the coagulopathy occurring in these patients is mediated by uncontrolled release of neutrophil extracellular traps (NETs). Emerging data indicate that hypercoagulability in COVID-19 is induced by dysregulated release of NETs.25 Glucocorticoid therapy may decrease NET formation and may in part explain the therapeutic benefit observed by implementing corticoid therapy in severe COVID-19.25
It is worth noting that early guidelines cautioned against using systemic glucocorticoids because of concerns about deteriorating clinical state, delayed virus clearance, and adverse effects.26–28 Currently, the data do not support the use of systemic glucocorticoids in individuals who do not require supplementary oxygen.28,29 In the RECOVERY trial, a subgroup analysis demonstrated no improvement in outcome and a trend toward harm in individuals not requiring oxygen therapy.28
A cohort study conducted at Montefiore Medical Center in New York demonstrated that patients with CRP of 20 or greater benefitted from steroids, while patients with CRP below 10 showed nonstatistically significant trends toward harm.29
The choice, dose, and duration of corticosteroid also remain a matter of controversy. While a fixed dose of 6-mg dexamethasone emerged from the RECOVERY trial, multiple trials have compared this regimen to higher doses of methylprednisolone, a steroid with a much longer track record of effectiveness in lung disease. A dose of 1 to 2 mg/kg/day has been proposed, with several head-to-head studies demonstrating substantively beneficial effect sizes over dexamethasone 6 mg, based on important outcomes (mortality, duration of ICU stay), and in more severe refractory cases, pulse dose methylprednisolone has been demonstrated to be more effective.28,30,31
Methylprednisolone achieves higher lung tissue concentrations than dexamethasone, which raises questions about whether it would be more effective.
In a recent prospective triple-blinded randomized controlled trial, 2 mg/kg of methylprednisolone was found to be superior to fixed-dose dexamethasone employed in the RECOVERY trial (6 mg/day) in terms of hospital length of stay, need for MV, and improved clinical status.31
Remdesivir
Remdesivir is an inhibitor of the SARS-CoV-2 RNA-dependent RNA polymerase (RdRp), which is essential for viral replication. At present, it is the position of the WHO that there are insufficient data to recommend for or against the use of remdesivir.18 We suggest that clinicians make the judgment about which patients may be appropriate recipients of remdesivir based on their risk of clinical deterioration.
The Adaptive COVID-19 Treatment Trial (ACTT-1), a randomized controlled trial that compared remdesivir to placebo in hospitalized individuals, demonstrated no significant benefit in patients with mild-to-moderate disease.32 In an open-label randomized trial involving individuals with moderate disease, individuals treated with remdesivir for 5 days demonstrated improvement in clinical symptoms by day 11. The results of this trial are of questionable benefit.33
The Solidarity trial, a large, multinational, open-label randomized controlled trial in which a 10-day course of remdesivir was compared to standard of care, demonstrated no difference between groups in the primary end point of in-hospital mortality.34 These trials have conflicting results and as such there is a paucity of data to advise for or against establishing remdesivir as routine therapy for hospitalized patients with moderate COVID-19. In light of this, the NIH panel recognizes that there may be situations in which a clinician judges that remdesivir is an appropriate treatment for a hospitalized patient with moderate disease (e.g., a person who is at a particularly high risk for clinical deterioration).
Ascorbic Acid
The coronavirus elicits a cytokine storm with subsequent lung damage including oxidative damage from reactive oxygen and nitrogen species. There is ongoing inflammation and pulmonary injury in that setting, which may lead to subsequent respiratory failure. It has been theorized that large dosages of an antioxidant such as ascorbic acid may blunt the oxidative stress induced by the coronavirus. Additionally, during states of increased oxidative stress, ascorbic acid may be rapidly depleted and as such mega dosages may be required.
A proof-of-concept trial l randomized adults in the ICU to receive parenteral vitamin C 24 g per day or placebo for 7 days. Unfortunately, due to the case of COVID-19 dropping in China, the study was terminated early due to lack of enrollment. Overall, the study found no differences between the arms in mortality, the duration of MV, or the change in median sequential organ failure assessment (SOFA) scores. The investigators did observe a significantly improved PaO2/FIO2 ratio from baseline to day 7 in the treatment arm when compared to placebo.35
The COVID A to Z study investigated whether high-dose zinc and/or high-dose ascorbic acid decreased the severity or duration of symptoms compared with usual care among ambulatory patients with COVID-19. The investigators observed no statistically significant difference in duration or severity of symptoms between the treatment and the control groups.36
The NIH COVID-19 Treatment Guidelines Panel states that there are insufficient data to recommend for or against the use of ascorbic acid for COVID-19.
We recommend that ascorbic acid be given in the following doses: for non-ICU patients with O2 level less than 4 L on hospital ward, 2,000 to 4,000 mg oral in divided doses daily as tolerated until discharge; for those in ICU or with O2 level more than 4 L, 50 mg/kg IV every 6 hours up to 7 days or until discharge from ICU and then transitioning to oral dose as mentioned previously. If in ICU and not improving, consider mega-doses: 25 g IV twice daily for 3 days.37
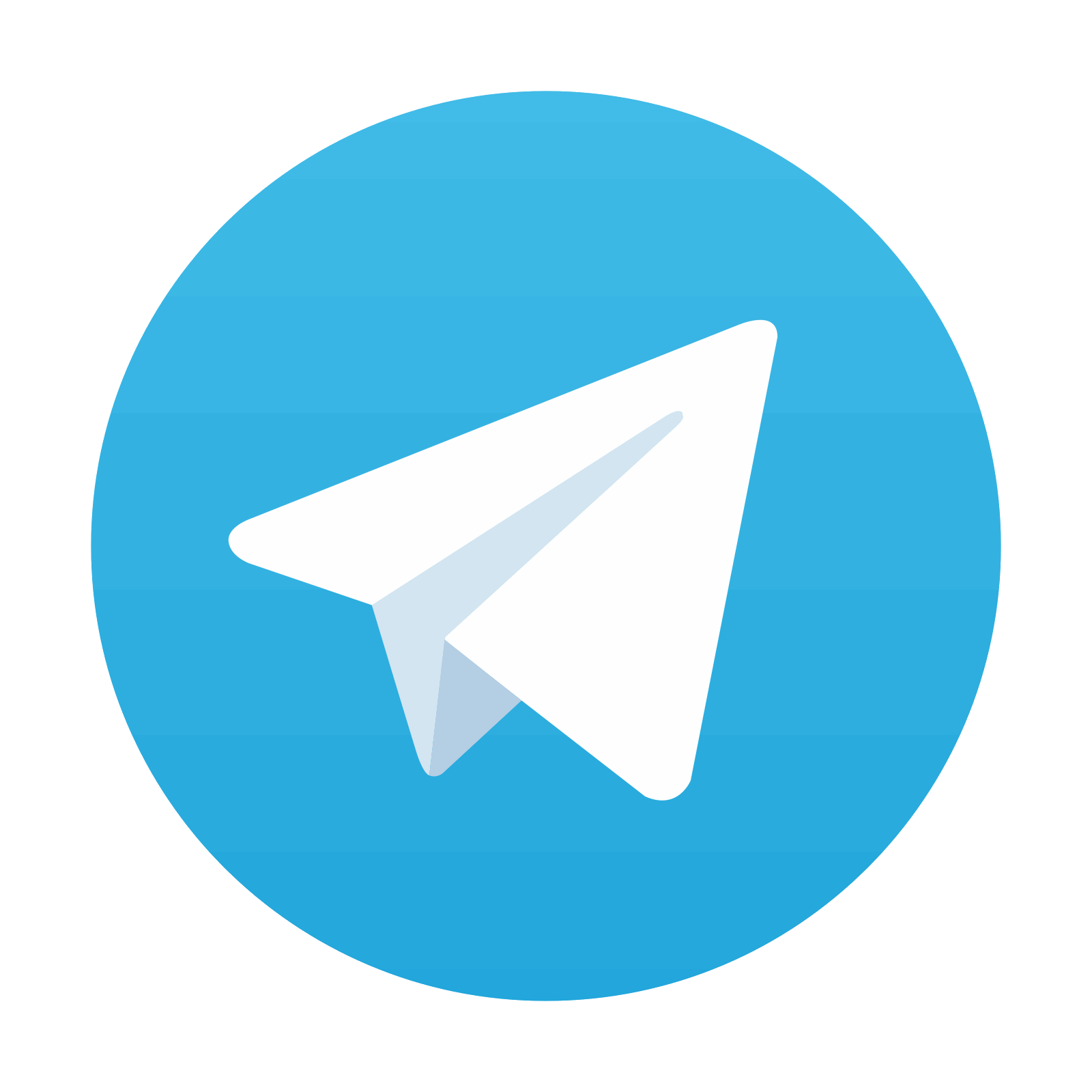
Stay updated, free articles. Join our Telegram channel

Full access? Get Clinical Tree
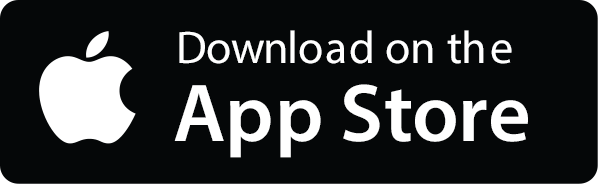
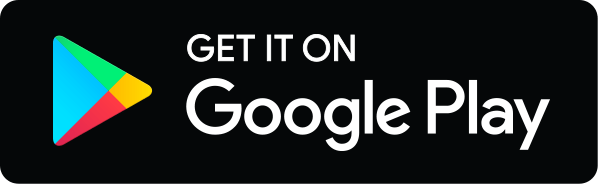
