Introduction
Severe acute respiratory syndrome coronavirus 2 (SARS-CoV-2) is the species of coronavirus responsible for the ongoing outbreak. This virus was named by a consensus scientific committee assembled by the World Health Organization, with classification based on genetic and structural analysis similarities relating to the general family of coronaviruses, genus Betacoronavirus and subgenus Sarbecovirus, and specifically relating to another Sarbecovirus responsible for a prior outbreak.1,2 It has been designated SARS because it is genetically related to the SARS-CoV Sarbecovirus and causes a similar clinical disease. In contrast, the Sarbecovirus named Middle East respiratory syndrome coronavirus (MERS-CoV) is less closely related based on genetic analyses.2
Pretty much everyone reading this has been infected by milder forms of coronaviruses without knowing it. Four different strains of human coronaviruses cause about one-fifth of all common colds. Until 2003, coronaviruses were generally thought to only cause mild disease in humans. Then SARS came along and changed that assumption forever. SARS-CoV-2 is the seventh recognized coronavirus with human hosts.1–3 The four human adapted “common cold” coronaviruses are 229E, NL63, OC43, and HKU1. The more recently discovered sarbe-coronavirus, known as SARS-CoV, MERS-CoV, and SARS-CoV-2, are able to cause severe morbidity and mortality, with mortality rates of 10, 37, and 1.04 to 3.09% worldwide (0.7–2.3% in the United States), respectively.1,2,4 Other feline enteric coronavirus (FECV) types of coronaviruses are pathogenic and endemic in certain animal populations, and some readers may have heard of coronaviruses infecting cats, dogs, or cattle (FECV, canine respiratory coronavirus [CRCoV], and bovine coronavirus [BCV], which are different from SARS-CoV-2 virus and COVID-19 disease).5
Coronaviruses: The Transition from Zoonotic Nuisance to Human Pandemic
The SARS-CoV-2 epidemic, which started in China during 2003, abruptly shifted the paradigm that human coronaviruses only cause mild disease. Susan Weiss of the University of Pennsylvania has stated that “Everybody in the field was shocked,” and then added: “People started really caring about this group of viruses.”6 It is thought that the epidemic began when a coronavirus spread from other mammals to humans. Infectious diseases that pass from animals to humans are called zoonotic diseases or zoonosis. SARS, MERS, and SARS-CoV-2 are all zoonotic diseases. To illustrate the importance of SARS, the government of the People’s Republic of China is estimated to spend well over the equivalent of over a billion U.S. dollars per year every year since the initial SARS outbreak to monitor and protect its citizens from SARS and to eliminate the disease.3 There is no vaccine for SARS; numerous, well-funded vaccine development efforts have all failed. The propensity of this family of coronaviruses to demonstrate the ability to transition from animal species to humans was again highlighted in 2012, when the MERS-CoV adapted from camels to humans.7 As of the end of December 2020, there have been a total of 2,566 laboratory-confirmed cases of MERS, including 882 associated deaths.8 As with SARS, all efforts to date to develop a MERS vaccine have failed. MERS is a good reason to not quit your day job to join the circus and become a camel trainer or handler.
SARS and SARS-CoV-2 most likely originated in bats (SARS-CoV-2 may have been a product of gain-of-function research) and probably had an intermediate animal host.3,4 The sequence of SARS-CoV-2 as isolated from humans is genetically closely related to coronaviruses isolated from bats of the genus Rhinolophus (“horseshoe bats”), and SARS is also related to coronaviruses found in bats.2,9 Similar viruses also exist in pangolins and civet cats.10–12 These close genetic relations suggest that all of these sarbecoviruses have their ecological origin in bat populations. Bats of the genus Rhinolophus are found across Asia, Africa, the Middle East, and Europe. Analysis of genomic sequences of SARS-CoV-2 also shows that it is very adapted to human cell receptors.13 This enables it to invade human cells and easily infect people. Bats have similar immune systems to humans, but it is sort of like the volume is turned down on how bats respond to viral infections. One interesting thing about bats is that their body temperature spikes as they fly, which may inhibit virus replication. This and other factors appear to make it easier for bats to live with certain types of viral infections, including some coronaviruses. However, when a bat coronavirus evolves to be able to infect a human, everything changes. The immune system of humans is generally turned up toward stronger immediate responses (“inflammation”) relative to bats. SARS-CoV-2 in a bat is not very pathogenic to the bat, due to the bat’s higher immunologic level of tolerance of viruses compared to humans. However, when this virus infects a human, it can result in the human immune system reacting in new, different, and unusual ways. SARS-CoV-2 in humans causes immune upregulation of mast cells, which control the release of histamine. The very high histamine release then causes damage to the human body. This is one new important aspect of the severe COVID-19 disease threat that we are now facing.14 How the SARS-CoV-2 first came to productively infect humans (apparently during October–November 2019) remains a mystery, but there are many theories ranging from natural zoonotic transmission to escape from a research laboratory.
Initial reports from Chinese scientists theorized that the virus originated from snakes because of similarities in fragments of both genomes, but that theory was quickly debunked. Then, based on bat biology and an analysis of sequence data from various strains of coronavirus, the primary animal host species was determined most likely to be the horseshoe bat. According to local officials in Wuhan, China, where the outbreak is thought to have started, there were no bats being sold at the live animal market in Wuhan city. However, 66% of the original cases had contact with the animal market. However, after tracing down the earliest cases of disease caused by this virus, epidemiologists have not been able to establish a clear link between early human cases of disease and any exposure to the live animal food market in Wuhan, China. However, the World Health Organization believes that this is the probable cause.15 This report suggests that an intermediate host species may have been involved but then goes on to disclose that although 188 animals spanning 18 species were tested, none were positive for COVID-19.15 The Wuhan Institute of Virology and the live animal market are in close proximity, so another theory is that the virus is an escaped mutation from the institute. This theory is supported by many in the U.S. government, although the World Health Organization investigated this thoroughly and no data have ever been found by western investigators linking the virus to the institute.
Having an intermediate animal host other than bat seems to be a common feature of zoonotic coronaviruses.1,2,9,11 This is what happened with MERS and its intermediary host, the camel. These intermediate animal hosts may increase the viruses’ genetic diversity and mammalian host range by facilitating development of more or different mutations via cross-species virus transmission. Viruses infrequently develop the potential to spread efficiently within a new host that has never been exposed to or sensitive to them. Devastating epidemics can occur when there is increasing exposure or viruses acquire mutations that permit them to overcome limits to infect new hosts.16
In the case of SARS-CoV-2, the intermediary host probably served as a sort of bridge between bat and human. The pangolin, an endangered animal that some Chinese use for traditional Chinese medicine (sold for as much as $350 per kilo), supports replication of a coronavirus that shares about 90% homology with SARS-CoV-2.9,16–18 Early on in the outbreak, it was hypothesized to be the animal intermediate. As the horseshoe bat range does not overlap with Wuhan, the most likely mammalian host of SARS-CoV-2 may reside far outside of the Wuhan province. Based on this logic, the original crossover from bat to human may have happened elsewhere in China and then transported to Wuhan by either an intermediary animal or human host. Members of the cat family (felids) as well as civets (there are a dozen members of the “civet cat” family) share an angiotensin-converting enzyme 2 (ACE2) receptor similar to the human version.5,9,11 These and other species that can be infected by this virus have also been considered as an intermediate host. In the end, the origins of how this virus cross into humans remain shrouded in mystery and clouded by conspiracy theories. As the science on the evolution of the virus progresses into how it made the leap from an animal host to human, that data will influence how humans interact with and treat other species. This, in of itself, could influence culture beyond the current crisis and could change how humans view and interact with the natural world. For example, the high transmissibility rate of this virus has permanently impacted global tourism, travel, and commerce.
There are many vulnerable species, such as those in the great ape family, that can be infected by this virus. The transmissibility and disease severity in most of these is not known. How this may affect other species found in domestic or wild populations including those found in urban zoos is not yet determined. For example, during January 2021, two gorillas at the San Diego Zoo tested positive for SARS-CoV-2 and developed COVID-19 disease, exhibiting mild respiratory symptoms. Since then, zoos have begun to vaccinate animal species that may be in danger of contracting this virus.19–21 But how this virus may impact other mammalian and even avian species is still unknown. Many animals do have cell surface receptors (such as ACE2) that will support SARS-CoV-2 infection. Studies have shown that critically endangered nonhuman primate species are predicted to be at very high risk of infection by SARS-CoV-2 via their ACE2 receptors. Other animals that are at high risk include other nonhuman primates and marine mammals. Domestic animals demonstrate a medium risk for getting infected with this virus. However, dogs, horses, and pigs were found to have low risk for ACE2 binding. Documented SARS-COV-2 infection of mink, cats, dogs, hamsters, lions, and tigers may rely on ACE2 receptors or may employ alternate pathways to acquire entry to host cells.22 Furthermore, as the virus continues to mutate and become more transmissible, there is the potential that future virus mutations may support infection in additional animal populations.
About the Virus
Coronaviruses are about 120 nm in diameter, which is fairly large for a virus. Just for comparison, the thickness of a single human hair is more than 1,000 times wider than a single coronavirus. Coronaviruses are spherical in shape, and under high-powered microscopes they look like they have a ring or halo around a central sphere, like the corona around the sun. And no, coronaviruses have nothing to do with the famous Mexican Corona brand beer! These viruses use a single strand of RNA, rather than a double strand of DNA, to carry their genetic code. RNA is not as stable as DNA, and so even a simple dish soap can do a lot of damage to a coronavirus.23
Coronaviruses are known to cause pulmonary and gastrointestinal diseases in various animals with coughing, pneumonia, and diarrhea all being typic symptoms.24 Examples of animals that get infected by different coronaviruses include camels, cattle, chickens, bats, mice, alpacas, swine, dogs, horses, and cats as well as other mammals and (of course) human and nonhuman primates.24 There are many different types of coronaviruses, and different coronaviruses tend to specialize in infecting different animals. For example (as discussed previously), SARS-CoV-2 is in the same coronavirus family (group 3) as MERS and SARS, but is different from cat (feline) coronavirus and also different from the human coronaviruses that cause the common cold. MERS, SARS, and the novel coronavirus demonstrate genetic homology and each has its genesis in bats, although the MERS virus also infects camels and is primarily transmitted to humans from camels. Genetic analysis demonstrate that SARS-CoV-2 is more closely related to SARS than to MERS, but is even most closely related to a subset of group 3 coronaviruses that virologists refer to as the bat SARS-like coronaviruses.2,5,9,11SARS, SARS-CoV-2, and closely related pangolin, civet cat, and horseshoe bat coronaviruses all show significant levels of conserved nucleocapsid 2 protein COX-2 promoter transactivation domains and nuclear localization regions.25
Coronaviruses and other single-stranded RNA viruses are classified as positive- or negative-stranded depending on the polarity of the RNA.26,27 One can think of RNA polarity as sort of like reading a sentence from left to right (positive polarity) or right to left (negative polarity). The machinery in cells that makes proteins from RNA reads the RNA (message) from left to right. Therefore, getting really technical, coronaviruses are a type of positive-polarity single-stranded enveloped RNA virus, which is to say that virus proteins can be produced directly from the RNA genome by reading it from left to right.26,27 The RNA does not have to go through another round of replication (as is required for negative-polarity RNA viral genomes) to get back to a form that can be read from left to right to produce proteins after infecting a cell. At a practical level, this also means that the RNA genome of a coronavirus can be infectious; the RNA alone, if transferred into a cell, can cause that cell to produce complete and infectious new coronaviruses. This is why mRNA vaccines only use a fragment of the mRNA genome, so that the mRNA cannot reproduce virus. The life cycle of SARS coronavirus and cellular disruption induced by viral invasion are demonstrated in Fig. 2.1.
Fig. 2.1 Life cycle of SARS-CoV-2. (a) Attachment of virus occurs via viral interaction via its S protein and ACE2 and TMPRSS2, resulting in endocytosis of virion. (b) S2-mediated fusion of virus membrane with endosomal membrane with resultant release of genome +ssRNA into cell cytoplasm. (c) Synthesis of replicase polyprotein products of subgenomic mRNA, inducing disruption of cellular processes. (d) Transcription of new viral genomes. (e) Structural proteins encoded by subgenomic mRNA. (f) Assembly and budding at membranes of the endoplasmic reticulum Golgi body complex. (g) Exocytotic release. (Source: ViralZone, SIB Swiss Institute of Bioinformatics; http://creativecommons.org/licenses/by/4.0/.)
Using RNA as the genetic material is very efficient (a single strand is easier and cheaper to make than two!), but it is also very likely to develop errors during replication relative to using double-stranded DNA (like human beings use). Among other problems with this viral strategy is that this means that viruses that use RNA often mutate very fast. Good thing that human beings use DNA to store their genetic information! For humans, lots of mutations would generally be very bad. However, RNA viruses make this high mutation rate work for them. The high mutation rate of RNA viruses is one reason why it is difficult to make effective vaccines against many of these types of viruses. Influenza virus is an RNA virus, and often mutates from one year to the next, requiring new and slightly different influenza vaccines to be produced every year. Positive-sense RNA viruses account for a significant proportion of all known human viruses, including many pathogenic viruses such as HIV (the AIDS virus), hepatitis C virus (liver cancer), rhinoviruses (common cold), West Nile virus, dengue virus, Zika virus, SARS and MERS coronaviruses, and COVID-19. Even though the single-stranded RNA strategy comes with the problem of high mutation rate, these viruses replicate so efficiently and produce so many viruses so fast that it does not slow them down. In fact, the high mutation rate is sort of an advantage for viruses—it makes it easy for them to evolve and adapt to a new host (you and me) very rapidly, and to adapt to escape immunity in the animals that they infect (including us). The SARS-CoV-2 virus that is currently circulating in humans is a group of closely related quasi-species; in other words, there is still significant diversity in the genetic sequences of all SARS-CoV-2 viral genomes when different samples of the novel coronavirus are sequenced.28
Variants
There are now many strains of SARS-CoV-2 circulating, with new strains being discovered on a regular basis. The occurrence of new variants affects viral transmissibility (R0), pathogenicity, reinfection rates, and SARS-CoV-2 vaccine effectiveness. These factors will influence how the world responds to the virus. Travel, commerce, government policy, health care systems, and other social infrastructure will all be influenced by how these new variants impact on the human body differently than the current strain(s) now circulating.
Mutations within the virus genome are clustered, and it appears that the most important of these genetic variation clusters may be in a region defined as ORF8 (open reading frame 8). Mutations in ORF8 were also associated with adaptation of the SARS virus to human infection. ORF8 (or the alternative form ORF8ab) appears to be involved in viral replication and/or disease, but the way that it works has not been completely defined at this point. What is known is that ORF8 disrupts IFN-I signaling when exogenously overexpressed in cells.29 Also known is that ORF8 of SARS-CoV-2 downregulates MHC-I in cells, but that the ORF8 in SARS does not.30 These data imply that the SARS-CoV-2 is still evolving to adapt to a new host (humans), and has not settled in on a predominant final strain. Therefore, any current assessments or predictions of future behavior (infectivity, incidence of disease, and death) may change as the virus settles down into a dominant viral strain and sequence, if it does settle. It is also important to know that this is a single-stranded RNA virus and these viruses mutate very fast. There have been reports of the virus’ genome being different at various time points within an individual. Another RNA virus with this capability that we are all familiar with is HIV.
Mode of Action
Viral infections can cause multiple different pathophysiologic patterns. One example of this is damage caused by direct virus injury and another example involves reactive host immune-mediated pathology in response to the pathogen. These could also be described as a hyperinflammatory immune response, which can cause significant clinical injury or even death. In the case of COVID-19, mast cell activation releases histamine and is a significant cause of organ damage.14 Although there are reports that a significant proportion of SARS-CoV-2-positive individuals demonstrate little or no symptoms, a large systematic review of literature documented that between 20 and 31% of SARS-CoV-2-positive patients are truly asymptomatic throughout the entirety of their infection.31 This means that viral infection is required but not sufficient for development of clinical disease.
The predominant pathophysiology in COVID-19 disease involves hyperimmune-mediated responses that are most frequently observed in high-risk patients, such as those with advanced age and those with comorbid medical conditions such as high blood pressure, obesity, diabetes, and cardiac, vascular, neurologic, renal, chronic lung, and asthmatic diseases. In particular, metabolic syndrome, caused by insulin resistance, hyperglycemia, high blood pressure, and subclinical inflammation are linked with a high risk of severe disease.32 All of these risk factors are associated with chronic proinflammatory states, which may predispose the patient to the hyperinflammatory responses typical of COVID-19 disease.
Viral spike protein and nucleocapsid N protein induce overexpression of COX-2. COX-2 is an enzyme that is the inducible form of cyclo-oxygenase.14,25 This enzyme catalyzes the conversion of arachidonic acid to prostaglandins. Prostaglandins are a class of lipids that are produced at locations of tissue damage or infection and modulate the disease process.
They control processes such as inflammation, thrombosis, and circulation at an injury site. COX-2 is expressed by inflammatory cells, such as macrophages, and can be induced by tumor necrosis factor (TNF) and epidermal growth factor.
COX-2 is most likely the dominant source of prostaglandin formation in inflammation. Patients with COVID-19 disease frequently have abnormally high amounts of prostaglandin E2 (PGE2). PGE2 and histamine are two of the inflammatory signaling molecules likely involved in mediating COVID-19 hyperinflammatory responses to viral infection as well as the superimposed coagulopathy.14,25 Coagulopathy results in thromboembolic events, leading to significant morbidity and mortality. The increased hyperinflammatory responses and coagulopathy correlate with a parallel rise in markers of inflammation. Many of these inflammatory processes are mediated by the COX-2 isoenzyme system. Pharmaceutical inhibition of COX-2 can provide reduce inflammation and hence modulate the hyperimmune-mediated responses associated with severe COVID-19.14,25
COX-2 is expressed in many different types of cells, including lung fibroblasts, vascular endothelial cells, type II alveolar pneumocytes, and alveolar macrophages. These cell types are all found in compromised pulmonary gas exchange tissue during the early stages of COVID-19 disease.33 Vascular endothelial cells and type II alveolar pneumocytes are primary targets for viral infection and replication, and alveolar macrophages are permissive to infection.34
SARS-CoV can transactivate the inducible COX-2 gene promoter in infected cells. SARS transcriptional upregulation of COX- 2 is mediated by the nucleocapsid protein (N, directly via specific N2 protein sequences) and spike glycoprotein (S, via indirect transactivation cascade), promoting upregulation of COX-2 in a dose-dependent manner in infected cells.35,36 The ability of SARS-CoV-2 to similarly transactivate COX-2 expression is untested, but nonclinical studies reveal viral infection does upregulate COX-2 expression.37 Homology analysis of the analogous N2 sequences of SARS-CoV-2 and other betacoronaviruses relative to SARS has not been reported. Based on urinary levels of PGE2, viral infection is associated with high levels of PGE2 production, which were several fold higher than levels observed in normal uninfected individuals (17,040 vs. 18,138 ng/mL, p = 0.01), and in some cases increased to 150 times the normal level in one study of hospitalized patients.38 These investigators postulated that increases in PGE2 potentially play a pivotal role in the pathophysiology of COVID-19 by binding to EP2, EP3, and EP4 receptors.38 EP2 receptor binding is associated with pyrexia, pain, acute inflammation, and enhanced capillary leak. EP3 receptor binding via a mast cell activation–dependent pathway leads to edema, inflammatory mucus secretion, and increased viscosity of alveolar exudate, resulting in mucous plugging of alveolar and respiratory bronchioles.39 EP4 receptor binding causes bronchial contractions and spasms, resulting in increased airway resistance, and is associated with pulmonary and circulatory disorders, acute respiratory distress syndrome (ARDS), and multiorgan failure.40 Excessive PGE2 binding to EP4 is also associated with inhibition of T lymphocyte functionality by promoting amplification, differentiation, and proliferation of Th1 and Th17 subtypes.41 In addition, PGE2 and thromboxane A2 activation can cause platelet aggregation and thrombosis.42 All of these signs and symptoms of excessive signaling by the products of arachidonic acid metabolism resulting from COX-2 activation are features of COVID-19 disease.
Researchers have attempted to elucidate COVID-19 clinical and pathologic data using just traditional models of antiviral innate and adaptive immune responses, which overlook the contribution of mast cell activation and degranulation. Other investigators highlight the inflammatory cell response cascade related with monocytes, macrophages, and adaptive T and B lymphocyte helper and effector responses.43,44 These immune responses have also been suggested as a possible explanation for the unique microvascular and macrovascular pulmonary and systemic coagulopathy linked to COVID-19.45 All of these reviews are flawed because they do not show the entire landscape of the virus–host immunologic interactions and cascading immunologic events.
Clinically, the most serious effects of COVID-19 disease are the pathologic hyperinflammatory host responses described in the subset of critically ill symptomatic SARS-CoV-2-infected patients. These responses manifest as exuberant immunological and paracrine pathway alterations. While COVID-19 symptoms are systemic in nature, ARDS is the most common cause of morbidity and mortality. Evaluation of RNA transcriptomic profiles of the cells that contribute to pulmonary anatomy and function reveals the existence of multiple ACE2/transmembrane protease serine 2 (TMPRSS2)-positive cell types susceptible to viral infection in the lung. Furthermore, these and other associated lung cells that express histamine receptors H1 and H2 may react to local histamine release following mast cell degranulation.46
Mast cell activation caused by SARS-CoV-2 infection is responsible for a portion of the central pathophysiological cascade and much of the unique manifestations linked with COVID-19.14,25,47 A fair number of the unique symptoms observed in the early stages of COVID-19 are compatible with established histamine-related consequences.48 Histamine may function in an autocrine fashion regulating mast cell cytokine and TNF-α release mediated by a PGE2-dependent mechanism. In vitro studies demonstrate the autocrine feedback appears to be mediated by H2 and H3.14,49 This model is consistent with the histopathologic findings observed during surgery and postmortem studies, and is substantiated by clinical pharmacologic findings suggesting potential benefits of histamine H2 receptor blockade, such as famotidine for treatment of COVID-19 disease. Substantial overlap exists between the clinical manifestations of the early phase of COVID-19 and those of mast cell activation syndrome14,25,50,51
There are also many resemblances to dengue hemorrhagic fever and shock syndrome (including T cell depletion) during the later stages of COVID-19.50–52 The cardiovascular complications, cerebrovascular accidents, and related complications linked with COVID-19 are similar to the Kounis syndrome.53–55 This theory is substantiated by the discovery of increased amount of mast cells in the alveolar septal walls and lung tissues of SARS-CoV-2-infected African green monkeys and in the alveolar septa of COVID-19 patients.56
If COVID-19 is partially driven by dysfunctional mast cells, then a variety of treatments employing FDA-approved therapeutic agents useful in the management of mast cell–associated diseases may lead to a reduction in morbidity and mortality in patients suffering from COVID-19.14 These include mast cell stabilizers such as beta-2 adrenoceptor antagonists or cromolyn sodium, other histamine antagonists (for example H1 and H4 types), leukotriene antagonists and leukotriene receptor antagonists, anti-inflammatory agents such as those developed for inflammatory bowel diseases, and mast cell activation inhibitors.57–65 If such repurposed drugs are used in combination with pharmaceuticals that directly inhibit SARS-CoV-2 infection or replication, it may be possible to rapidly develop potent, safe, and effective outpatient treatments for preventing or treating COVID-19. The emergence of new variants can affect viral transmissibility, disease severity, reinfection rates, and SARS-CoV-2 vaccine effectiveness. The need for antiviral and therapeutic drugs to treat COVID-19 disease and long hauler syndrome has not diminished with the advent of emergency-use authorized vaccines. Breakthrough disease, undervaccinated populations, and new variants that continue to evolved mean that this disease needs more effective treatments. By understanding the link between COVID-19, mast cells, and histamine release, combined with the structural elements of this virus, new therapeutics can be developed.
ACE2 is a transmembrane protein that was first discovered in 2000.66,67 This protein is found on epithelial and endothelial cells of the upper and lower respiratory tract, heart and vasculature, kidneys, and portions of the gastrointestinal tract.68 ACE2 is an ectoenzyme. An ectoenzyme means that its actions occur outside of the cell. ACE2 is found in many different organs that can be affected by SARS-CoV-2.68 The primary role of the ACE2 protein is to counterbalance the effects of the first angiotensin-converting enzyme, commonly referred to as ACE.68 The primary role of ACE2 relates to the renin–angiotensin system (RAS)—the hormone system that regulates blood pressure: volume and tone, electrolyte balance, and systemic vascular resistance. RAS is regulated by the action of angiotensin II, which is a peptide hormone.68
In SARS-CoV-2 infection, the viral S (spike) protein receptor-binding domain binds to ACE2. This allows the virus to enter the host cell. ACE2 expression in the lungs is relatively low, but it is present in type II pneumocytes, which are the surface epithelial cells of the alveoli that are adapted to carry out gas exchange. This cell type is also endowed with TMPRSS2.68 This protease binds the SARS-CoV-2 S protein to ACE2. That is the key that allows entry into the cells. Blocking TMPRSS2 could potentially be an effective clinical therapy for COVID-19, as it would effectively not allow the viruses spike protein to bind and enter the cell.69
SARS-CoV-2 include four major structural proteins. These proteins are the spike (S), nucleocapsid (N), membrane (M), and envelope (E) proteins. The S, M, and E proteins are all embedded in the viral surface envelope. The other structural protein (N) forms the viral ribonucleoprotein core.68–70 S proteins are involved in recognition of the host cellular receptor to initiate virus entry. The M proteins are in and shape the virion envelope.70 E proteins are small polypeptides that are responsible for coronavirus infectivity. N proteins are the building blocks of the helical nucleocapsid and they bind along the viral RNA genome. As well as these four structural proteins, SARS-CoV-2 encodes at least 16 nonstructural proteins and nine accessory proteins.70 Several of these viral proteins could potentially serve as targets of vaccine-induced immune responses.70
For the development of vaccine against SARS-CoV-2, the spike (S) protein is most often chosen.70 The spike (S) protein of SARS-CoV-2 is an important element in the receptor recognition and cell membrane fusion process. The spike protein is composed of two subunits, named S1 and S2. The S1 subunit contains a receptor-binding domain that recognizes and binds to the host receptor ACE2.70 The S2 subunit is responsible for viral cell membrane fusion. It does this by forming a six-helical bundle via the two-heptad repeat domain.71 The spike protein is essential for viral entry into cells, and it is present on the outer layer of the virus. This makes it an ideal target for vaccination strategies against SARS-CoV-2, as antibodies and cytotoxic T lymphocyte (in the case of mRNA vaccines) targeting the S protein prevent the virus from entering the host cell. If the virus does not enter the cell, it cannot replicate inside the host cell.72 The Moderna, Pfizer, and AstraZeneca vaccines all target the spike (S) protein. The fundamental role of the S protein in viral infection indicates that it is a potential target not just for vaccines, but also for antibody-blocking therapies and small molecule inhibitors (antiviral drugs).
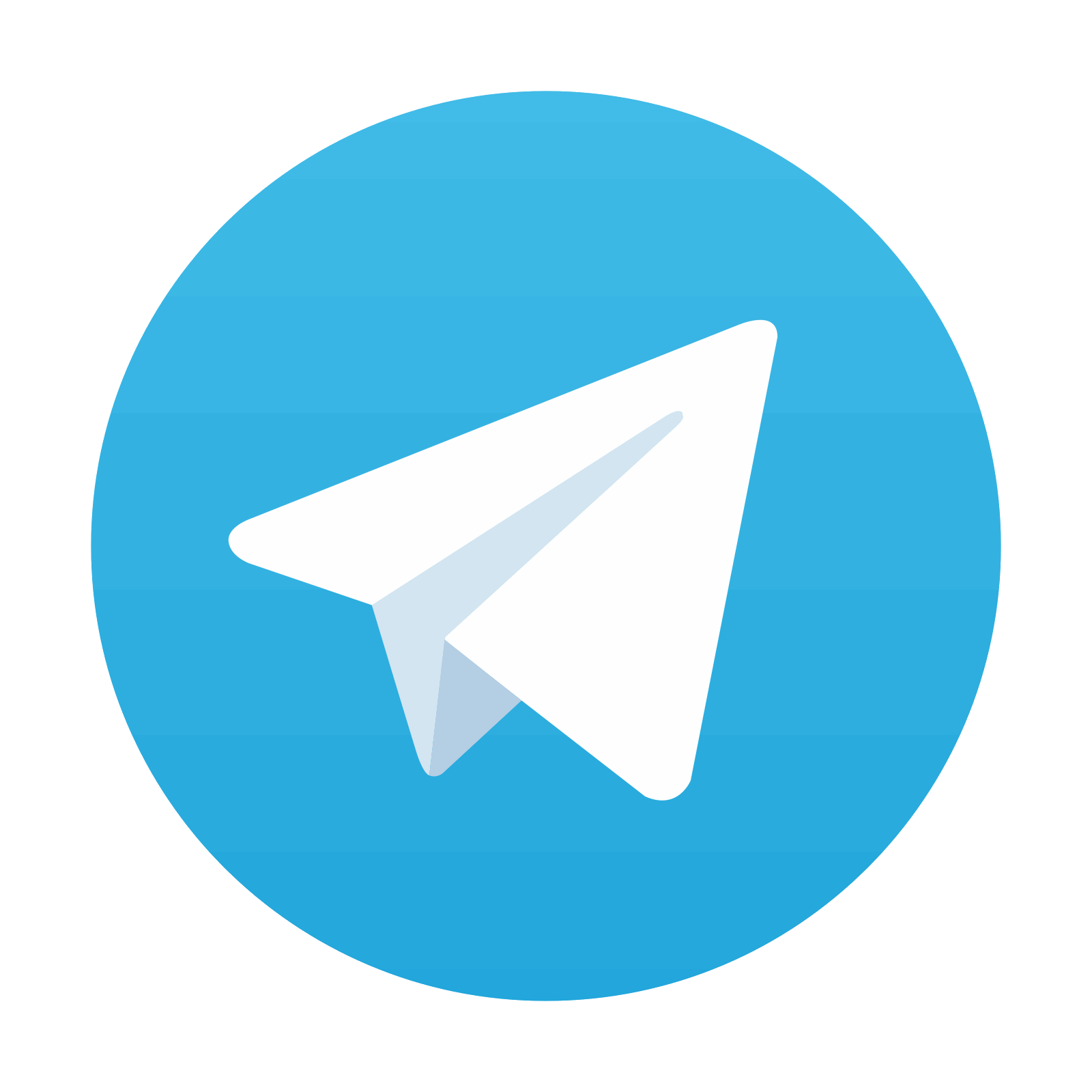
Stay updated, free articles. Join our Telegram channel

Full access? Get Clinical Tree
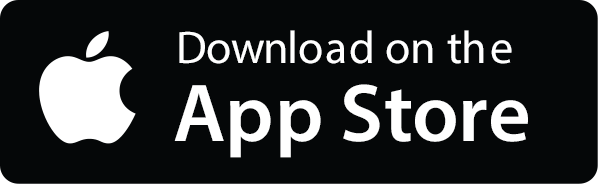
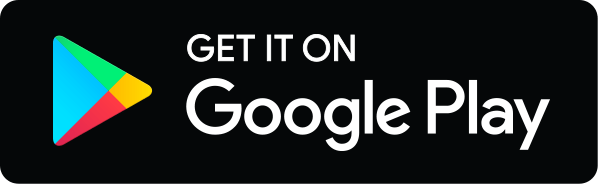