Introduction
Coronaviruses—thus named for their unique crownlike spikes visible under microscopy—are a diverse class of viruses that are known to cause intestinal and respiratory infections within both bird and mammal hosts. On a molecular level, they are enveloped, positive-sense viruses that contain single-stranded RNA as their genetic material. Further, coronaviruses belong to the family Coronaviridae, which are known to cause respiratory disease in humans.
COVID-19, the infectious disease caused by severe acute respiratory syndrome coronavirus 2 (SARS-CoV-2), has been documented to have a wide range of outcomes. Following infection, certain individuals remain asymptomatic or merely develop mild upper respiratory symptoms, while others present with serious conditions such as pneumonia and/or severe acute respiratory distress syndrome, which could possibly require intubation and lead to complications.
In symptomatic patients, COVID-19 can be modeled by breaking symptoms down into four distinct phases, the first of which is characterized by upper respiratory tract infection accompanied by fever, muscle fatigue, and pain. While most patients experience no further symptoms, individuals that progress further develop pneumonia (which can be either symptomatic or asymptomatic) as well as dyspnea. In the third stage, patients worsen as a result of cytokine release syndrome, also known as “cytokine storm,” which floods the bloodstream with inflammatory proteins known as cytokines. This state of hyperinflammation, in turn, can cause renal damage, sepsis, and secondary infection. Finally, in the fourth and final stage, patients either recover or die, the outcome of which is dependent on a myriad of factors including age, race, and past medical history.
Delivering optimal care for SARS-CoV-2 and all future health crises necessitates an understanding of the epidemiological trends of the COVID-19 pandemic; by analyzing and documenting these patterns, we can not only improve our ability to combat future pandemics, but also address current health-related disparities and inform future policy.
Geographic Distribution
Origins of COVID-19 and Global Spread
The origins of SARS-CoV-2 can be traced back to individuals infected within rural locales of the People’s Republic of China as early as October 2019. However, the virus appears to have been incapable of efficient human-to-human transmission during this time. In December 2019, a mutation of the virus occurred in Wuhan, a city located in the Hubei Province of China, resulting in improved transmissibility within human hosts.1,2 Although the Chinese government instituted a quarantine of Wuhan on January 23, 2020, widespread travel among its residents meant the outbreak could no longer be contained, introducing a global pandemic.
The current working theory for the zoonotic basis of SARS-CoV-2 posits that the virus originated in bats before undergoing multiple recombination events as it passed through mammals.3 Evidence supporting this hypothesis can be found in genomic studies. The genome of SARS-CoV-2 shares a 96% similarity to betacoronavirus isolated from a bat in 2013 (RaTG13).4 Further, the SARS-CoV-2 receptor binding motif—a portion of the virus instrumental for host infection—is closely related in sequence to the betacoronavirus isolated from a Malayan pangolin, more so than its bat counterpart (98.7 vs. 77.6% similarity).4
COVID-19 has since spread exponentially, with greater than 150 million cases having been reported globally spanning every continent. It should be noted that this figure is an underestimate of COVID-19’s overall disease burden, as not every case is ultimately reported. This is supported by seroprevalence survey studies conducted in the United States and Europe.5,6
Distribution of COVID-19 in the United States
Spread of COVID-19 proceeded rapidly in the United States: community transmission was first identified in February 2020, and by the middle of March, confirmed cases were reported in all 50 states, the District of Columbia, and four U.S. territories. During spring and early summer of 2020—the initial months of the pandemic—case distribution was heavily skewed in favor of densely populated urban centers such as New York, Boston, and New Orleans relative to less populous rural areas. In July 2020, however, this urban–rural disparity began to close, with less densely populated areas beginning to see a rise in case levels.7
Risk Factors
Ethnic/Racial Disparities in Disease Outcomes
There exist marked racial/ethnic inequities with respect to age-adjusted COVID-19 disease outcomes. In Chicago, Black individuals make up 30% of the population, yet contributed to 50% of COVID-19 cases and 70% of deaths as of July 2020, most of them concentrated within the city’s most vulnerable communities.8 At the national level, Pacific Islanders, Latinos, Blacks, and Indigenous Americans have a COVID-19 death rate of more than double that of White and Asian Americans after controlling for age (Fig. 1.1).9 Ongoing research seeks to examine the extent to which these observed poorer outcomes among people of color can be attributed to direct effects of the SARS-CoV-2 virus versus indirect consequences of the pandemic such as its effects on the job market, as well as its exacerbation of existing disparities in access to resources and health care.10 This clear incongruity in outcome measures has shaped the development of targeted economic interventions and vaccine information campaigns.
Fig. 1.1 Percentage of COVID-19 deaths among racial and ethnic groups (blue) compared to percentage of race and ethnic groups in the United States demonstrating a higher proportion of deaths among minorities compared to non-Hispanic Whites. (Source: https://www.cdc.gov/coronavirus/2019-ncov/community/health-equity/racial-ethnic-disparities/atl-disparities-black-patients.)
Social Determinants of Disease Outcome
Existing structural inequities—exacerbated by the brutal socioeconomic effects of the pandemic—likely have contributed to ethnic/racial disparities in COVID-19 outcomes. COVID-19-related mortality rates are also associated with factors such as education and economic status, with U.S. counties comprised of college-educated individuals with high-earning professions suffering fewer deaths on average than counties with a higher poverty prevalence.11 This observation is linked to risk of exposure, as individuals holding high-earning careers were more likely able to work remotely, allowing for better adherence to social distancing protocols. Persons of color were disproportionately represented among “essential workers” that were exempt from shelter-in-place mandates instituted during heightened waves of COVID-19 spread.10 Additionally, Blacks make up about roughly a quarter of all public transit users in the United States, further contributing to an increased risk of exposure.11 Finally, persons of color are more likely to live in crowded conditions such as multigenerational households that not only elevate exposure, but also limit the ability to quarantine.12
Ethnic/racial disparities in the incidence of chronic conditions have also been implicated in COVID-19 outcomes. Previous diagnoses of conditions such as cardiovascular disease, chronic kidney disease, chronic lung diseases (such as chronic obstructive pulmonary disease), diabetes mellitus, hypertension, and obesity have been demonstrated to predispose patients to more severe disease activity, increasing risk of intubation and death13: data reported by the Centers for Disease Control and Prevention (CDC) illustrate that over 90% of patients who were hospitalized due to COVID-19 had at least one pre-existing condition.14 Notably, the incidence rates of these chronic diseases are disproportionately high among people of color, partially as a consequence of ethnic/racial disparities within health determinants such as socioeconomic status, education, and availability of health care resources.15–17 Thus, vulnerability to COVID-19 based on the presence of these comorbidities in part is driven by the convergence of existing structural inequities along racial lines, further informing the increased mortality rates observed among minorities. Dr. Anthony Fauci, Director of the National Institute of Allergy and Infectious Diseases and Chief Medical Advisor to the President, emphasized these disparities in an April 2020 speech, particularly among Black communities: “Health disparities have always existed for the African American community… [COVID is] shining a bright light on how unacceptable that is because, yet again, when you have a situation like coronavirus, they are suffering disproportionately.”18
Changes in Racial/Ethnic Distribution of COVID-19 Incidence over Time
Analysis of incidence data reported by the CDC from January through December 2020 indicates that COVID-19 ethnic/racial inequities changed over time in adults aged 25 years or older. Specifically, during the early stages of the pandemic (i.e., January–April), this disparity was more pronounced among ethnic minorities relative to White individuals. Over time, this gap began to close, although as a consequence of increased incidence within White individuals rather than decreases within minority populations. The largest disparities observed were among Native Hawaiian/Pacific Islander, Native American, and Hispanic individuals.19
Age-Related Disparities in Disease Outcomes
Among unvaccinated individuals, age is the most significant predictor of poor outcomes and mortality within COVID-19 patients,20 with the distribution of cases heavily skewed toward older age groups.21,22 In fact, the SARS-CoV-2 virus has a remarkably low infection rate among children as well as a decreased propensity toward exhibiting symptoms. For instance, it is estimated that the risk of infection in individuals older than 20 years is roughly double that of those younger than 20 years.23 Further, while individuals between the ages of 18 and 29 years make up the highest percentage of cases in the United States among all age groups, over 94% of COVID-19-related deaths were observed in patients older than 50 years (Fig. 1.2).20
Fig. 1.2 Percentage of deaths from COVID-19 among persons older than 65 years or persons of any age who died in a nursing home. (Reproduced with permission from Gold JAW, Rossen LM, Ahmad FB, et al. Race, ethnicity, and age trends in persons who died from COVID-19—United States, May–August 2020. MMWR Morb Mortal Wkly Rep 2020;69(42):1517–1521.)
Factors Driving Increased COVID-19 Mortality among Older Patients
An explanation for this age distribution disparity lies within the presence of chronic health conditions (CHCs), which have been demonstrated to significantly worsen COVID-19 outcomes. Older individuals have a higher prevalence of such CHCs, including hypertension, diabetes, coronary heart disease, and chronic kidney disease: a study conducted on critically ill older patients with SARS-CoV-2 found that 86% had at least one CHC diagnosis.24 Furthermore, having multiple chronic diseases—the likelihood of which increases with age—has been shown to place patients at even greater risk of mortality from COVID-19.25
The use of angiotensin-converting enzyme (ACE) inhibitors—a frequently prescribed type of medication among older patients—has also been thought to worsen outcomes, providing further explanation for the observed distribution of age-related COVID-19 mortality.25 The foundation of this theory lies in the SARS-CoV-2 viral mechanism of action: external spike proteins anchor the virus to cellular ACE2 receptors, allowing for viral entry. Experimental studies in animal models have found that inhibition of the renin–angiotensin–aldosterone system (RAAS) via ACE inhibitors results in a compensatory increase in tissue expression of these ACE2 receptors, generating speculation as to whether this could adversely affect COVID-19-related outcomes through enhancement of viral binding and cell entry.26,27 Conversely, other researchers have postulated that RAAS inhibitors could be advantageous in COVID-19 patients by increasing expression of angiotensin 1–7 and 1–9, both of which have vasodilatory and anti-inflammatory effects that could potentially attenuate lung damage. Ultimately, COVID-19 clinical guidelines have cleared ACE inhibitors, stating that there is no evidence of adverse effects as a consequence of continuing these medications.28–31 To this point, there were no significant differences in the average number of days alive and out of hospital—a composite measure that integrates clinically important outcomes such as death, hospital length of stay, and readmission to hospital—between patients who continued and discontinued ACE inhibitor therapy, lending support to its acceptability within COVID-19 patients.31
COVID-19 Infection Rates and Outcomes in Children and Adolescents
By observation, children appear to be less vulnerable to COVID-19 infection than adults: per 2020 data from China, children aged 0 to 15 years make up 17.8% of the population, yet only represent 2.1% of reported cases.32 However, this does not necessarily indicate that children have a lower rate of infection than adults: a study found that children younger than 10 years who were exposed to COVID-19 were at similar risk of infection compared to all other age groups.33 Thus, while risk of infection remains the same across age groups, younger patients are less likely to suffer from severe disease activity. Moreover, the number of laboratory-confirmed cases of SARS-CoV-2 infection in children is an underestimate, a consequence of the high proportion of mild and asymptomatic cases within this age group. This assertion is validated by a seroprevalence study that found the reported number of cases within children to be significantly lower than the actual number of infections.34
Understanding the factors that drive these clear incongruities in outcomes between children and adults remains an ongoing area of research. One proposed explanation lies in the fact that children have less mature ACE2, potentially disrupting the SARS-CoV-2 virus’ ability to anchor onto cells via their spike proteins. Additionally, children—those who are younger, in particular—tend to have frequent viral infections; thus, there is a possibility that this repeated exposure could support the immune system in its response to SARS-CoV-2.35
Transmission
Person-to-Person Transmission
Direct Respiratory Transmission: The Dominant Route of Transmission
Direct respiratory transmission is dominant, with proximity and ventilation being key determinants of infection risk. Transmission is thought to occur when two individuals—at least one of whom is infected—come in close contact with each other, specifically within 6 feet distance. When in close proximity, virions suspended on either large droplets or fine aerosol particles are expelled from the respiratory tract of the infected individual via mechanisms such as coughing, sneezing, and/or speaking. When these droplets/aerosols are inhaled or come into contact with a mucous membrane of an uninfected person, SARS-CoV-2 is able to effectively spread from person to person.36 Therefore, proximity plays a crucial role in SARS-CoV-2 transmission, informing the social distancing and quarantine protocols that have been instituted by public health experts.
Environmental Transmission
Airborne Transmission
In addition to direct person-to-person respiratory transmission, SARS-CoV-2 also has the ability to spread over longer distances via airborne transmission, occurring when individuals inhale viral particles that remain in the air. Recent work has demonstrated that coughs, sneezes, and exhalations produce ejecta that take the form of turbulent clouds composed of hot, humid air and suspended mucosalivary droplets, making them multiphase in nature. This gas-phase component has critical implications toward extending the longevity of these viral droplets: the warm, moist environment within these turbulent clouds allows droplets to evade evaporation for a much greater period of time than if they were isolated. This, in turn, has the ability to increase the lifespan of a droplet from seconds to minutes, allowing for the possibility of infections over time and distance.37,38 This is validated by studies that analyzed transmission patterns within certain outbreaks (namely, an indoor restaurant, choir practice, and public bus), all of which conclude that airborne spread is plausible, particularly within indoor, poorly ventilated environments.39–41 Further, a 2020 report from China illustrated that lingering SARS-CoV-2 particles can be found in the hospital rooms of infected patients, underscoring the significance of ventilation in managing transmission.
Overall, however, observational studies utilizing secondary attack rate (SAR) as a means of quantifying spread suggest that this mechanism is not a primary route of transmission. In epidemiology, SAR is defined as the number of cases occurring within the incubation period upon exposure to a primary case divided by the number of susceptible individuals.42 Overall, only about 5% of close contacts to patients with confirmed COVID-19 become infected, although this rate is dependent upon the duration and intensity of contact.43 For instance, sharing a meal is associated with a 7% SAR, while passing interactions during shopping has a 0.6% rate. Further, in health care workers unknowingly caring for infected patients, the SAR is roughly 3%.44 Notably, among these few cases, the providers were found to have performed aerosol-generating procedures and/or underwent prolonged exposure with inconsistent usage of face masks.45,46
Fomite Transmission
SARS-CoV-2 also has the capacity to spread indirectly through contaminated intermediates known as fomites, which are defined as objects or materials that are likely to carry infection. These fomites become infected either through direct contact with another fomite or by settling airborne viral particles. Fomite transmission can occur when an individual makes physical contact with a fomite and then touches a facial membrane, although its overall contribution is currently thought to be insignificant.47 While there are little available data on the environmental stability of SARS-CoV-2, there is evidence to suggest that the virus is able to persist on surfaces such as metal, glass, ceramics, plastic, and rubber to varying degrees of longevity, lasting anywhere from hours to greater than a week.48
In general, while this type of transmission necessitates that the virus is able to persist in an area outside of its given host, certain environmental characteristics can influence infectivity retention and speed of spread. Specifically, SARS-CoV-2 transmission is optimized at low temperatures and low humidity, which partially explains the surge of COVID-19 cases observed during colder seasons.49 Simulated sunlight has also been experimentally determined to inactivate SARS-CoV-2 over the course of 15 to 20 minutes, with higher levels of ultraviolet-B (UVB) linked to more rapid inactivation. These results suggest that persistence and exposure risk, by extension, vary significantly between indoor and outdoor environments, and have contributed to the development of past economic shutdown policies.50 Taken together, an environment that poses the greatest risk for SARS-CoV-2 fomite transmission are indoor settings with heavy viral contaminations (i.e., an infected patient’s hospital room), and it highlights the continued importance of hygiene vigilance and cleaning protocols in public settings.
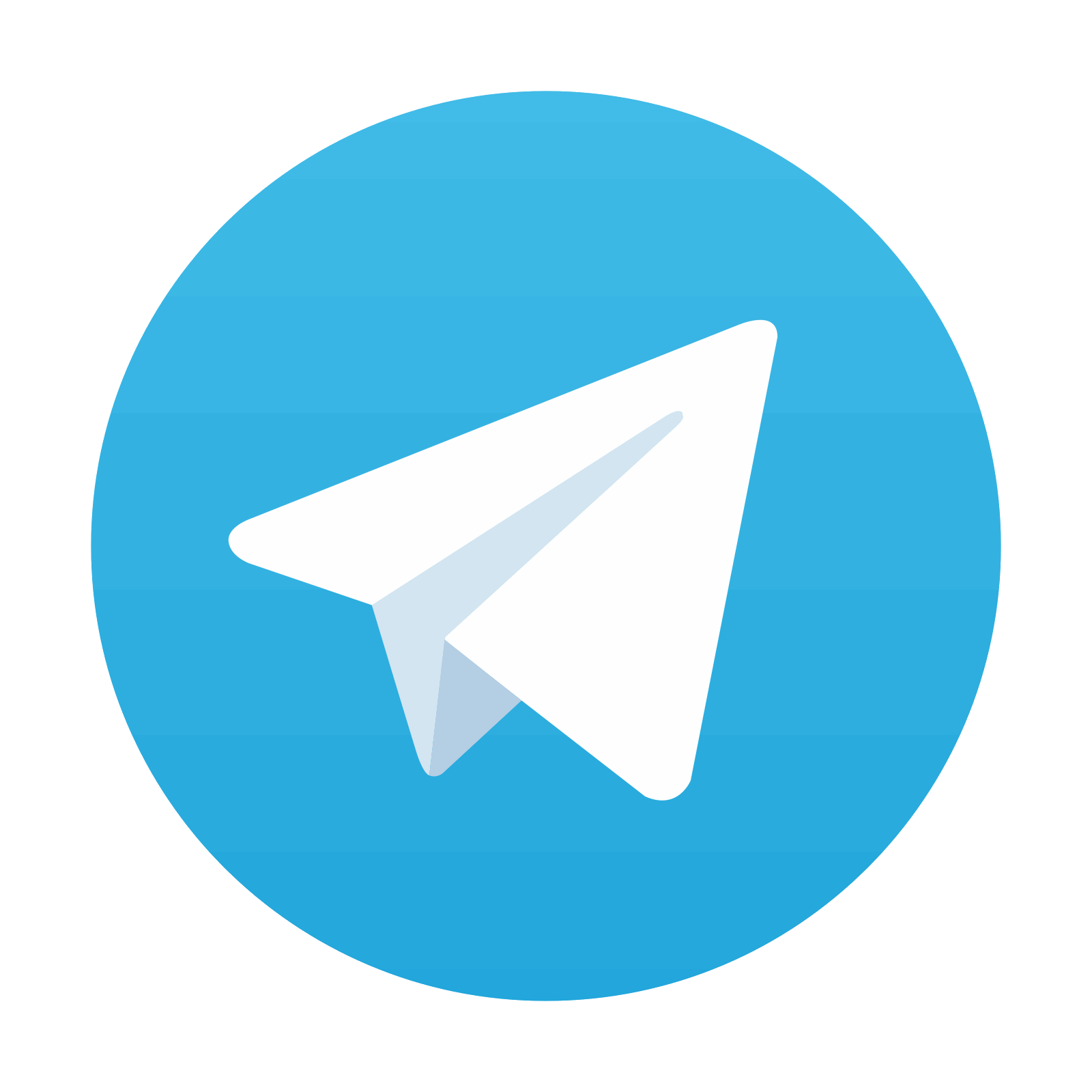
Stay updated, free articles. Join our Telegram channel

Full access? Get Clinical Tree
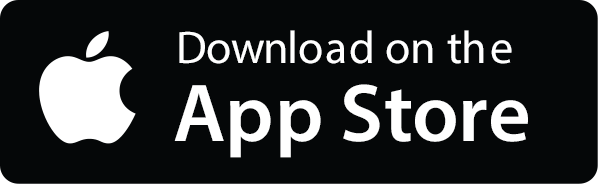
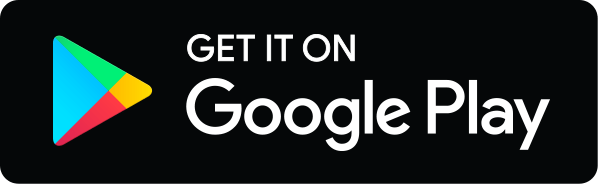
