Kinetics Following an Extravascular Dose
OBJECTIVES
The reader will be able to:
- Define the following terms: absorption rate constant, absorption half-life, absorption phase, bioavailability, bioequivalence, biopharmaceutics, delayed-release dosage forms, elimination phase, first-order, flip-flop, generic products, extended-release dosage forms, immediate-release dosage forms, lag time, method of residuals, modified-release dosage forms, peak concentration, peak time, relative bioavailability, zero-order absorption.
- Describe the characteristics of, and the differences between, first-order and zero-order absorption processes.
- Determine whether absorption or disposition rate limits drug elimination, given plasma concentration–time data following different dosage forms or routes of administration.
- Anticipate the effect of altering absorption kinetics, extent of absorption, clearance, or volume of distribution on the plasma concentration and amount of drug in the body following extravascular administration.
- Estimate the bioavailability of a drug, given either plasma concentration or urinary excretion data, following both extravascular and intravascular administration.
- Estimate the relative bioavailability of a drug, given either plasma concentration or urinary excretion data following different dosage forms or routes of administration.
- Estimate the renal clearance of a drug from plasma concentration and urinary excretion data following extravascular administration.
ROUTES OF EXTRAVASCULAR ADMINISTRATION
Drugs are more frequently administered extravascularly (common routes listed in Table 2-3 of Chapter 2, Fundamental Concepts and Terminology) than intravascularly, and most are intended to act systemically rather than locally. For most drugs then, systemic absorption, the focus of this chapter, is a prerequisite for activity. Delays or losses of drug during systemic input may contribute to variability in drug response and, occasionally, may result in failure of drug therapy. It is primarily in this context, as a source of variability in systemic response and as a means of controlling the plasma concentration–time profile, that systemic absorption is considered here and throughout the remainder of the book. It should be kept in mind, however, that even for those drugs that are used locally (e.g., mydriatics, local anesthetics, nasal decongestants, topical agents, and aerosol bronchodilators), systemic absorption may influence time of onset, intensity, and duration of both local and systemic adverse effects.
This chapter primarily deals with the general principles governing rate and extent of systemic drug absorption, particularly after oral administration. Several oral dosage forms are used. Some are liquids (syrups, elixirs, suspensions, and emulsions), whereas the more common ones are solids (tablets and capsules). Tablets and capsules are generally formulated to release drug immediately after their administration to hasten systemic absorption. These are called immediate-release products. Other products are called modified-release dosage forms.
Modified-release products fall into two categories. One is extended-release, a dosage form that allows a reduction in dosing frequency or diminishes the fluctuation of drug levels, or both, on repeated administration compared with that observed with an immediate-release dosage form. Controlled-release and sustained-release products fall into this category. The second category is that of delayed-release. This kind of dosage form releases drug, in part or in total, at a time other than promptly after administration. Enteric-coated dosage forms are the most common delayed-release products; they are designed to prevent drug release in the stomach, where it may decompose in the acidic environment or cause gastric irritation, and then to release the drug for immediate absorption once in the intestine. Modified-release products are also administered by nonoral extravascular routes. For example, repository (depot) dosage forms are given intramuscularly and subcutaneously in the form of emulsions, solutions in oil, suspensions, and tablet implants. While the duration of drug release is limited for orally administered products by the gastrointestinal transit time (typically 12–36 hr), no such limit applies to nonoral routes of administration. Products may be designed to deliver drug over weeks (octreotide acetate for injectable suspension [Sandostatin LAR Depot], used in treating acromegaly, 4 weeks); months (e.g., goserelin acetate implant [Zolodex, 10.8 mg, used in prostate cancer], 3 months); or years (levonorgesterol-releasing intrauterine system [Mirena, contraceptive system], 5 years).
The physiologic and physicochemical factors that influence drug absorption are considered in Chapter 7, Absorption. In this chapter, the following aspects are examined: the impact of rate and extent of absorption on the time course of both plasma concentration and amount of drug in the body; the effect of alterations in absorption and disposition on body level-time relationships; and the methods used to assess pharmacokinetic parameters from plasma and urinary data following extravascular administration.
KINETICS OF ABSORPTION
The oral absorption of drugs often approximates first-order kinetics, especially when given in solution. The same holds true for the absorption of drugs from many other extravascular sites, including subcutaneous tissue and muscle. Under these circumstances, absorption is characterized by an absorption rate constant, ka, and the corresponding absorption half-life, t1/2,a. The two are related to each other in the same way that elimination half-life is related to elimination rate constant, that is,
The half-lives for the absorption of drugs taken orally in solution or in a rapidly disinte-grating dosage form usually range from 15 min to 1 hr. Occasionally, they are longer.
When absorption occurs by a first-order process,
Restated, the rate is proportional to the amount remaining to be absorbed, Aa. First-order absorption is schematically depicted in Fig. 6-1 by the emptying of water from a cylindrical bucket. The rate of emptying depends on the amount of water in the bucket and the size of the hole at the bottom. With time, the level, and hence the head of pressure of water decreases, reducing the rate at which it leaves the bucket. The rate of emptying is directly proportional (ka) to the level or amount of water in the bucket.
FIGURE 6-1. First-order systemic absorption is analogous to the emptying of water from a hole in the bottom of a cylindrical bucket. The level of water in the bucket decreases with time, as does the rate of emptying. The slowing of the decline of the water level and the rate of emptying are a result of the decrease in water pressure, which depends on the water level (or amount of water) in the bucket. The rate of emptying (g/min), which declines exponentially with time, is proportional to the amount (g) of water in the bucket and the size of the hole. The rate of emptying relative to the amount in the bucket is the fractional rate of emptying, which does not vary with time. In absorption terms, this constant is called the absorption rate constant, ka.
Sometimes, a drug is absorbed at essentially a constant rate. The absorption kinetics is then said to be zero order in nature. Differences between zero-order and first-order kinetics are illustrated in Fig. 6-2. For zero-order absorption, a plot of amount remaining to be absorbed against time yields a straight line, the slope of which is the rate of absorption (Fig. 6-2A). Recall from Chapter 3, Kinetics Following an Intravenous Bolus Dose, that the fractional rate of decline is constant for a first-order process; the amount declines linearly with time when plotted semilogarithmically. In contrast, for a zero-order absorption process, the fractional rate increases with time, because the rate is constant but the amount remaining decreases. This is reflected in an ever-increasing gradient with time in a semilogarithmic plot of the amount remaining to be absorbed (Fig. 6-2B).
FIGURE 6-2. A comparison of zero-order (colored lines) and first-order (black lines) absorption processes. Depicted are regular (A) and semilogarithmic (B) plots of the percent remaining to be absorbed against time. Note the curvatures of the two processes on the two plots.
For the remainder of this chapter, and for much of the book, absorption is assumed to follow first-order kinetics. When absorption is zero order in nature, then the concepts and equations developed in Chapter 10, Constant-Rate Input, apply.
EXPOSURE–TIME AND EXPOSURE–DOSE RELATIONSHIPS
The systemic exposure to a drug after a single extravascular dose depends on both absorption and disposition. Consider first how exposure with time after an extravascular dose compares with that seen after an intravenous (i.v.) dose.
COMPARISON WITH INTRAVENOUS ADMINISTRATION
Absorption delays and reduces the magnitude of the peak compared with that seen following an equal i.v. bolus dose. These effects are portrayed for aspirin in Fig. 6-3. The rise and fall of the drug concentration in plasma are best understood by remembering (see Eq. 2-2) that at any time,
FIGURE 6-3. Aspirin (650 mg) was administered as an i.v. bolus (black) and as an oral solution (colored) on separate occasions to the same individual. Absorption causes a delay and a lowering of the peak concentration. (From: Rowland M, Riegelman S, Harris PA, Sholkoff SD. Absorption kinetics of aspirin in man following oral administration of an aqueous solution. J Pharm Sci 1972;67:379–385. Adapted with permission of the copyright owner.)
When absorption occurs by a first-order process, the rate of absorption at any time is given by ka · Aa.
The scheme in Fig. 6-4 illustrates the expectation. Drug is input into the reservoir by a first-order process and is eliminated in the same manner as that following an i.v. dose (see Fig. 3-3).
Initially, with the entire dose at the absorption site (bucket, see Fig. 6-1) and none in the body (reservoir), rate of absorption is maximal and rate of elimination is zero. Thereafter, as drug is absorbed, its rate of absorption decreases, whereas its rate of elimination, reflecting the amount in the body, increases. Consequently, the difference between the two rates diminishes. As long as the rate of absorption exceeds that of elimination, the plasma concentration continues to rise. Eventually, a time, tmax, is reached when the rate of elimination matches the rate of absorption; the concentration (water level) is then at a maximum, Cmax. Subsequently, the rate of elimination exceeds the rate of absorption and the plasma concentration declines, as shown for aspirin in Fig. 6-3.
The peak plasma concentration is always lower following extravascular administration than the initial value following an equal i.v. bolus dose. In the former case, at the peak time some drug remains at the absorption site and some has been eliminated, whereas the entire dose is in the body immediately following the i.v. dose. Beyond the peak time, the plasma concentration is expected to exceed that following i.v. administration of the same dose, if the drug is fully bioavailable. This occurs because of continual entry of drug into the body. If bioavailability is low, the plasma concentration may remain lower than that observed after i.v. administration at all times. Frequently, the rising portion of the plasma concentration–time curve is called the absorption phase and, the declining portion, the elimination phase. As will be seen later, this description may be misleading.
FIGURE 6-4. Scheme for the first-order systemic absorption and elimination of a drug after a single extravascular dose. The systemic absorption is simulated by the emptying of a water bucket (see Fig. 6-1). The rate constant for absorption ka is the fractional rate of absorption (i.e., the rate of absorption relative to the amount in the bucket). The elimination of the drug from the body (see Fig. 5-3) depends on the extent of its tissue distribution (volume of reservoir, V), and how well the drug is extracted from the fluid going to the eliminating organ (s) (as measured by CL). In this integrated model, the amount of water added to the reservoir is negligible as is the amount of drug in the extractor and in the fluid going to the extractor, relative to the amount in the reservoir.
One may observe a delay between drug administration and the beginning of absorption. This is called a lag time; it may be particularly important when a rapid onset of effect is desired. The lag time can be anywhere from a few minutes to many hours. Long lag times are frequently observed following ingestion of enteric-coated tablets. Factors contributing to the lag time are the delay in emptying the product from the stomach and the time taken for the protective coating to dissolve or to swell and release the inner contents into the intestinal fluids. However, once absorption begins, it may be as rapid as with uncoated tablets. Because of variability in gastric emptying, enteric-coated products should not be used when a prompt and predictable response is desired.
Absorption influences the time course of drug in the body; but what of the total area under the exposure–time profile, area under the curve (AUC)? Recall from Chapter 3, Kinetics Following an Intravenous Bolus Dose, that the rate of elimination is:
Rate of elimination = CL · C 6-4
Integrating over all time,
Total amount eliminated = CL · AUC 6-5
The total amount eliminated after an oral dose equals the total amount absorbed, F · Dose, where the parameter F, bioavailability, takes into account that only this fraction of the oral dose reaches the systemic circulation. That is,
Absorption kinetics from plasma concentration–time data following an extravascular dose can be calculated when a one-compartment model for drug disposition and first-order kinetics apply (Appendix F, Absorption Kinetics). Other less restrictive but generally more complex numeric methods exist to deal with more complicated situations. One of these, the Wagner-Nelson method, is given in Appendix G, Wagner-Nelson Method.
CHANGES IN DOSE OR ABSORPTION KINETICS
The concentration–time profile following a change in dose or in the absorption characteristics of a dosage form can be anticipated.
CHANGING DOSE
If all other factors remain constant, as anticipated intuitively, increasing the dose or the fraction of a dose absorbed produces a proportional increase in plasma concentration at all times (Fig. 6-5). The value of tmax remains unchanged, but Cmax increases proportionally with dose.
FIGURE 6-5. Effect of change in dose on concentration–time curve. With a decrease in dose (10, 7.5, 5.0, 2.5 mg), note that the peak concentration (Cmax) and AUC decrease in proportion to the dose, but that the peak time (tmax) remains the same.
CHANGING ABSORPTION KINETICS
Alterations in absorption kinetics (e.g., by changing dosage form or sometimes when giving the product with food) produce changes in the time profiles of the plasma concentration. This point is illustrated by the three situations depicted in the semilogarithmic plots of Fig. 6-6, involving only a change in the absorption half-life. All other factors (bioavailability, clearance, volume of distribution, and hence, elimination half-life) remain unchanged.
Disposition is Rate Limiting. In Case A, the most common situation, absorption half-life is much shorter than elimination half-life. In this case, by the time the peak is reached, most of the drug has been absorbed and little has been eliminated. Thereafter, decline of drug in the body is determined primarily by the disposition of the drug, that is, disposition is the rate-limiting step. The half-life estimated from the decline phase is, therefore, the elimination half-life.
FIGURE 6-6. Rates of absorption (colored line) and elimination (black line) with time (graphs on left) and corresponding plasma concentration–time profiles (graphs on right) following a single oral dose of drug under different input conditions. A slowing (from top to bottom) of drug absorption delays the attainment (tmax) and decreases the magnitude (Cmax) of the peak plasma drug concentration. In Cases A and B (top two sets of graphs), the absorption process is faster than that of elimination. In Case C (bottom set of graphs), absorption rate limits elimination, so that the decline of drug in plasma reflects absorption rather than elimination; because there is a net elimination of drug during the decline phase, the rate of elimination is slightly greater than the rate of absorption. In all three cases, bioavailability is 1.0 and clearance is unchanged. Consequently, the areas under the plasma concentration–time curves (corresponding linear plots of the top three graphs) are identical. The AUCs of the linear plots of the rate data are also equal because the integral of the rate of absorption, amount absorbed, equals the integral of the rate of elimination, amount eliminated.
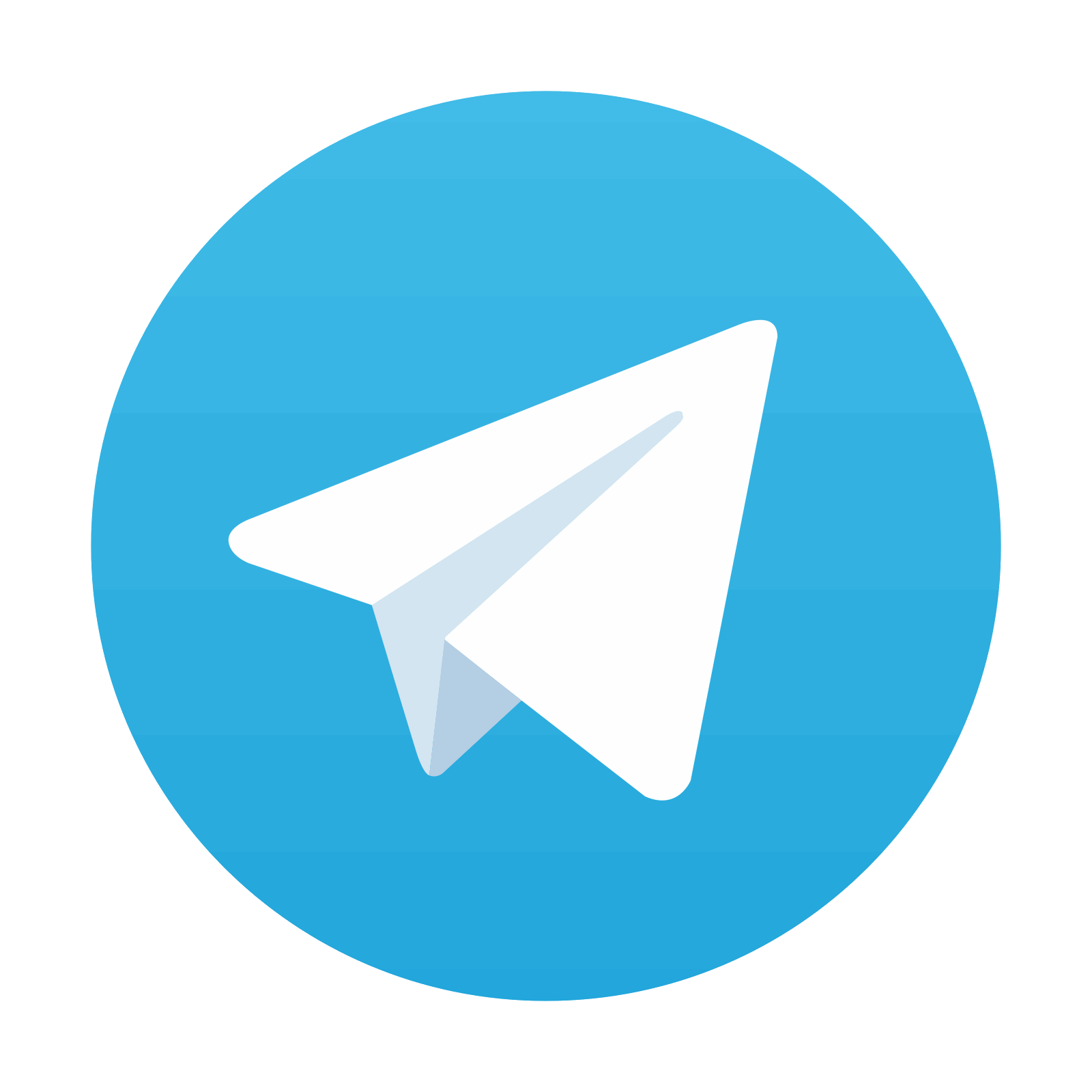
Stay updated, free articles. Join our Telegram channel

Full access? Get Clinical Tree
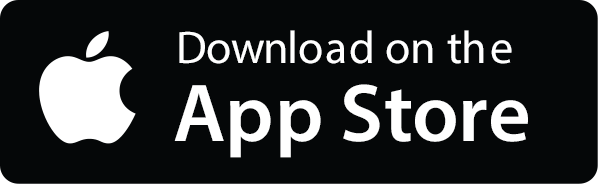
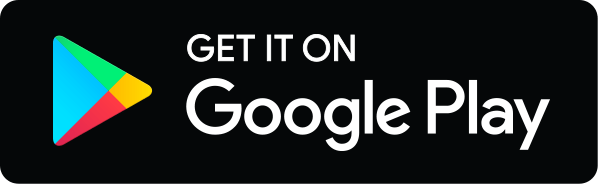