Types of Explosions and Explosive Injuries Defined
Richard Linsky
Adam Miller
The worldwide escalation of violence, both civilian and military, demands that medical personnel and first responders be prepared to deal with the consequences of bombings. Explosions serve terrorist goals well. They are dramatic and gain immediate public attention, creating chaos and mass hysteria. Bombings are not only meant to kill and maim, but to strike fear in those removed from the event itself. Despite widespread concerns regarding biological and chemical attacks, conventional and improvised explosive devices are by far the most commonly utilized terrorist weapons. According to FBI statistics, there were 12,216 bombings in the United States between 1980 and 1990, causing 1,782 injuries, 241 deaths, and almost $140 million of property damage. Between 1990 and 1994, there were 8,567 bombings and nearly 2,000 additional bombing attempts, the majority of which involved pipe-bomb-type devices (1). Suicide bombings in Israel are increasing exponentially, with 107 suicide bombings between 2000 and 2003 (2).
In most bombings, many more are injured than killed. For example, the 1993 bombing of the World Trade Center in New York City killed 6 but injured over 1,000. The truck bomb detonated outside the Murrah Federal Building in Oklahoma City in 1995 killed 168 and injured over 750. In 1998, the bombing of the U.S. embassy in Nairobi killed 253 and created a staggering 5,000 casualties. The large numbers of casualties generated can easily overwhelm any local medical system.
Explosions cause a unique form of barotrauma, known as primary blast injury, which affects principally the air-filled organs in the body. The violent forces created also cause all types of penetrating and blunt injuries, termed secondary and tertiary blast injuries, which are more familiar to medical personnel. The number of casualties and the severity of their injuries varies depending on the type of attack, size of the explosive charge, and whether located in a confined or open space (3). Improvements in prehospital trauma care and EMS systems have allowed more bombing victims to arrive at the medical center with signs of life (3). This chapter discusses types of explosive devices, blast physics, and the pathophysiology and management of blast-related injury, focusing on the organ systems most at risk.
TYPES OF EXPLOSIVE DEVICES
Many bombs manufactured by terrorists are relatively unsophisticated “improvised explosive devices,” or IEDs, made
using readily obtainable materials such as agricultural fertilizer or household cleansers. Recipes for such homemade bombs abound on the Internet. The pipe bomb, the most common type of improvised terrorist bomb, consists of low explosives inside a tightly capped piece of metal pipe, often wrapped with nails or scrap metal to cause even more harm. The Molotov cocktail is another easily constructed terrorist weapon that can cause considerable damage. Materials such as gasoline, diesel fuel, kerosene, ethyl or methyl alcohol, lighter fluid, or turpentine are placed in a glass bottle, which is thrown and breaks upon impact. A piece of cotton serves as a fuse, which is ignited before the bottle is thrown at the target (4).
using readily obtainable materials such as agricultural fertilizer or household cleansers. Recipes for such homemade bombs abound on the Internet. The pipe bomb, the most common type of improvised terrorist bomb, consists of low explosives inside a tightly capped piece of metal pipe, often wrapped with nails or scrap metal to cause even more harm. The Molotov cocktail is another easily constructed terrorist weapon that can cause considerable damage. Materials such as gasoline, diesel fuel, kerosene, ethyl or methyl alcohol, lighter fluid, or turpentine are placed in a glass bottle, which is thrown and breaks upon impact. A piece of cotton serves as a fuse, which is ignited before the bottle is thrown at the target (4).
More sophisticated explosive devices generally contain three basic elements: a trigger, a fuse, and the main charge. Various triggering mechanisms may be employed, including motion detectors, photoelectric cells, time switches, radiation triggers, and remotely controlled electronic signals. Once triggered, the fuse is activated and the main charge ignited.
Common high explosives include TNT, C4, PETN, and dynamite. High explosives can be mixed with plasticizers such as oil or wax to create soft, hand-malleable substances called plastic explosives, or plastique. Terrorists frequently employ plastic explosives because of their ease of concealment, and they have been the predominant weapons used in aviation-related terrorism incidents (5). Only 312 grams of the plastic explosive SEMTEX, connected to a battery and a barometric trigger mechanism, and small enough to be hidden inside a portable cassette player, was responsible for the destruction of Pan Am Flight 103 over Lockerbie, Scotland, in 1988.
An explosion is caused by the rapid chemical conversion of the main charge into a gas with resultant violent release of energy. Explosives are classified as “high” or “low,” depending on the speed with which they release that energy. Low explosives, such as flash powder or gunpowder, release energy relatively slowly via a process called deflagration. The low explosives exert more of a pushing or heaving effect and are generally used as propellants.
By contrast, high explosives undergo detonation when initiated, an almost instantaneous transformation of the original explosive material into gases filling the same volume and therefore under extremely high pressure (6). The highly pressurized gases expand rapidly and compress the surrounding medium, generating a pressure pulse that is propagated as a blast wave in all directions. It is this instantaneous rise in pressure that gives high-explosive blast waves the unique characteristic of brisance, or shattering ability. The rapidity with which an explosive reaches its peak pressure is a measure of its brisance. Low explosives do not release energy fast enough to demonstrate brisance.
PATHOPHYSIOLOGY OF BLAST INJURY
Although the physics are complex, a blast wave has three basic components: an extremely short burst of increasing pressure, a longer phase of negative pressure, and a massive movement of air called the blast wind. An idealized blast wave is depicted in Figure 20-1. During the positive-pressure phase, the blast front moves away from its point of origin at supersonic speeds. Under ideal conditions, the blast front is spherical and propagates outward equally in all directions. This positive-pressure phase, much more powerful than the negative phase, is responsible for the majority of the damage. The magnitude of the positive phase impulse, the peak overpressure, is the primary determinant of the severity of blast injury. The overpressure needed to cause blast injury to the lung is approximately 40 pounds per square inch (psi). More than half of all victims will suffer pulmonary damage with pressures of 80 psi or more; overpressures exceeding 200 psi are uniformly fatal (7).
The peak overpressure is governed largely by three factors: (a) the size of the explosive charge, (b) the distance from the detonation, and (c) the surrounding medium (air or water). The larger the charge, the greater the peak overpressure will be and the longer its duration. With the common high explosives used in civilian terrorist bombs, the duration of the positive-pressure impulse is 2 to 10 milliseconds (8). Small bombs that may be carried by hand or in a parcel may contain up to 30 pounds of explosives; car bombs may contain up to 500 pounds or more (9). The size of the truck bomb used in Oklahoma City in 1995 has been estimated to be about 4,000 pounds. The truck bomb that destroyed the Al Khobar Towers in Saudi Arabia in 1996 detonated with an estimated likely yield of more than 20,000 pounds of TNT-equivalent explosives. The 1998 bombing of the U.S. embassy in Nairobi was deadly but fairly small by comparison, equivalent to 3 metric tons of TNT. The 1993 World Trade Center bomb had the explosive power of about 2,000 pounds of TNT.
The peak overpressure at any given point is inversely proportional to the cube of the distance from the blast. As blast waves travel outward, their velocity and pressure fall off rapidly and eventually deteriorate into acoustic waves (10). As a result, casualties must be relatively close to the detonation, or the explosion must be of great magnitude, to make significant primary blast injury a likely finding in survivors (11). As a point of reference, an explosive charge equivalent to 20 kilograms of TNT that detonates more than 6 meters away from the body is unlikely to cause significant blast injury to the lung (7).
Because water is essentially incompressible, a blast wave will be transmitted with greater speed and intensity and over a longer range than the same blast wave in air (9). Underwater blast injuries are more prevalent at any given distance (12), and the lethal range of an underwater explosion is far greater than a similar open-air blast (13). The presence of reflecting or absorbing surfaces, such as walls or other people, will alter the peak overpressure. When reflected by a solid surface, a blast wave can be magnified many times the
original incident pressure. Victims who are near walls, large solid objects, or corners can have injuries several times greater than what would be expected for any given blast pressure (14). A recent Israeli study reported that mortality was 8% in open-air terrorist bombings but 49% in enclosed-space bombings. Similarly, the incidence of primary blast injury in enclosed spaces was twice that of open-air bombings. Body armor may protect from penetrating injury but acts like walls surrounding the thorax and can potentiate the blast effect (15). Animal studies have shown that intrathoracic pressure is generally higher in those wearing ballistic vests (15). The negative-pressure phase of the blast wave follows immediately after the positive phase and lasts about 10 times as long. The mass movement of air away from the blast epicenter creates an area of low pressure, rarely exceeding -1 atmosphere. The suction effect generated reverses the direction of the blast wind and pulls debris into the area, causing additional damage and injury (9).
original incident pressure. Victims who are near walls, large solid objects, or corners can have injuries several times greater than what would be expected for any given blast pressure (14). A recent Israeli study reported that mortality was 8% in open-air terrorist bombings but 49% in enclosed-space bombings. Similarly, the incidence of primary blast injury in enclosed spaces was twice that of open-air bombings. Body armor may protect from penetrating injury but acts like walls surrounding the thorax and can potentiate the blast effect (15). Animal studies have shown that intrathoracic pressure is generally higher in those wearing ballistic vests (15). The negative-pressure phase of the blast wave follows immediately after the positive phase and lasts about 10 times as long. The mass movement of air away from the blast epicenter creates an area of low pressure, rarely exceeding -1 atmosphere. The suction effect generated reverses the direction of the blast wind and pulls debris into the area, causing additional damage and injury (9).
Although the ultimate clinical presentation depends on whether an explosion occurs in open or confined quarters, open air, or water, the pattern of injury inflicted on the body is relatively consistent (3). As a blast wave passes through the body, it causes damage by several different mechanisms: spalling, implosion, inertia, and pressure differentials (9). Spalling occurs at tissue interfaces when particles from a denser medium are thrown into a less dense medium as a blast wave passes through. In the lungs, for example, particles of liquid are spalled into the alveolar space, much as water is thrown into the air in an underwater explosion (9). Blast waves passing through organs containing pockets of gas, such as the middle ear or the sinuses, cause implosion of the air pocket, followed by a rebound expansion once the wave has passed. Because air is easily compressible by a passing blast wave but fluid-containing tissues are not, pressure differentials develop at such air-fluid interfaces, causing shear forces that tear and disrupt tissues. Lastly, the inertia imparted from the force of the blast wind may propel a victim against a stationary object, causing injury on impact (9).
CLASSIFICATION OF BLAST INJURIES
Injuries caused by explosives have traditionally been divided into primary, secondary, and tertiary blast injuries, and miscellaneous or quaternary injuries. Primary blast injury is a form of barotrauma, unique to explosions, which causes damage to air-filled organs: the lungs, the gastrointestinal tract, and the auditory system. Secondary blast injury refers to penetrating trauma caused by the acceleration of shrapnel and other debris by the blast wind. Tertiary blast injury is caused by the displacement of the body by the blast wind, with typical patterns of blunt trauma occurring on forceful impact with a solid object or the ground.
PULMONARY BLAST INJURY
The hallmark of primary blast injury is damage to the respiratory system, or “blast lung,” and is manifested by varying degrees of pulmonary contusion and barotrauma. The extent of pulmonary pathology is directly proportional to the velocity of chest-wall displacement by the incoming blast wave (16), which in turn is dictated by the size of the explosive charge, distance from blast epicenter, orientation of the thorax in relation to the blast wave, and geography of nearby reflecting surfaces. Injury tends to be worse on the side of approach of blast waves in open air but is usually bilateral or diffuse when the victim is located in a confined space (17).
As a blast wave propagates through the chest, enormous pressure differentials are generated at interfaces between media of different densities (8,13) resulting in alveolar tears and vascular disruption. There is also evidence from rat lung models that subtle biochemical changes and free-radical-mediated oxidative stresses contribute to blast-induced injury (18,19). Pleural or subpleural petechiae are the mildest pathological findings (17,20). Higher energy stress waves conducted deeper into the lung can cause subpleural hemorrhage where the blast front first contacted the chest wall, as well as near the diaphragm and mediastinum, where reflections and summations of stress waves occur (7,21). Even more powerful blast impulses cause large parenchymal tears and stripping of small airway epithelium (22). Communication between the airways and the pleural space can lead to simple or tension pneumothorax, hemothorax, traumatic emphysema, and alveolo-venous fistulae, which, in the already compromised lung, will precipitate even more rapid respiratory failure. Unfortunately, the need for early positive-pressure ventilation to treat such patients with rapidly progressing respiratory failure can aggravate the situation, increasing the risk for both tension pneumothorax and air embolism (3).
Clinically, blast lung injury can appear very similar to a pulmonary contusion from blunt chest trauma but without associated rib fractures or chest wall injury (13,23). This is rarely seen in the absence of other secondary or tertiary explosion injuries. Like blunt pulmonary contusion, gas exchange is impaired at the alveolar level, and the degree of respiratory insufficiency depends on the extent of hemorrhage. Signs and symptoms of primary pulmonary blast injury may include dyspnea, difficulty completing sentences in one breath, rapid shallow respirations, poor chest wall expansion, cough, hemoptysis, decreased breath sounds, and wheezes.
SYSTEMIC AIR EMBOLISM
A dreaded complication of primary pulmonary blast injury is the development of arterial air embolism, thought to be responsible for most of the sudden deaths that occur within the first hour after blast exposure (24). The true incidence of systemic air embolism is unknown. Only those with clinical evidence of blast lung injury seem to be at risk, with a greater incidence following explosions in confined spaces (25).
Large blast impulses passing through the lung can cause hemopneumothoraces, traumatic emphysema, and vascular disruption (6). Air emboli result from the direct communication created between the traumatized pulmonary airspace and vasculature. Such communication can develop at any time after a blast injury, either directly from the blast or as a
complication of therapy for respiratory failure (24). Normally, in a patient with spontaneous respirations, the airway pressure remains lower than the pulmonary venous pressure. After blast trauma, states of low pulmonary venous pressure (hypovolemia) or high airway pressure (positive-pressure ventilation, coughing, or tension pneumothorax) may cause the pressure gradient to reverse, allowing air to enter the pulmonary circulation (26,27,28) and to be systemically disseminated. One of the first animal studies linking air embolism with blast lung injury subjected a dog to a near-lethal air blast. Showers of carotid air emboli were detected for 30 minutes after injury (29). Animal experiments have also demonstrated coronary air emboli in rats dying immediately following a blast (9).
complication of therapy for respiratory failure (24). Normally, in a patient with spontaneous respirations, the airway pressure remains lower than the pulmonary venous pressure. After blast trauma, states of low pulmonary venous pressure (hypovolemia) or high airway pressure (positive-pressure ventilation, coughing, or tension pneumothorax) may cause the pressure gradient to reverse, allowing air to enter the pulmonary circulation (26,27,28) and to be systemically disseminated. One of the first animal studies linking air embolism with blast lung injury subjected a dog to a near-lethal air blast. Showers of carotid air emboli were detected for 30 minutes after injury (29). Animal experiments have also demonstrated coronary air emboli in rats dying immediately following a blast (9).
Obstruction from air bubbles may occur in any vascular bed. The passage of air bubbles causes a transient precapillary block and arterial spasm, dilatation, stasis, and ischemia (9). Air emboli to the heart or brain are thought to be the most common cause of rapid death solely caused by blast injury in immediate survivors (30,31,32). The introduction of as little as 2 milliliters of air into the cerebral circulation can be fatal (33,34). Likewise, as little as 0.5 to 1 milliliter of air injected into a pulmonary vein can cause cardiac arrest from coronary air embolism (35).
Clinical manifestations of systemic air embolism depend on the location of embolic occlusion and on the degree of resultant ischemia. Air embolism is often heralded by a rapid decompensation immediately following intubation and positive-pressure ventilation (36); such collapse is usually unresponsive to conventional resuscitation (37). Hemoptysis, suggesting communication between pulmonary blood vessels and the airway, was seen in 22% of cases of systemic air embolism in one large series (38). Other symptoms include blindness due to air in retinal vessels, focal neurological deficit or loss of consciousness following cerebral obstruction, and chest pain from coronary obstruction and myocardial ischemia. Air emboli to the skin may produce a reddish-blue mottling discoloration called livido reticularis. Tongue blanching and pharyngeal petechiae may be seen. An arterial blood gas sample may appear frothy with air bubbles, and EKG may reveal arrhythmias or ischemia (39). In those with lung trauma, the combination of hemoptysis and circulatory or CNS dysfunction immediately after initiation of positive-pressure ventilation is sufficient to make the provisional diagnosis of systemic air embolism (27).
GASTROINTESTINAL (GI) BLAST INJURY
Gas-containing abdominal structures are injured in a similar manner as the lungs (17), but such injuries are frequently occult and overshadowed by the more immediately life-threatening manifestations of pulmonary blast injury (24). Military studies have found that in open-air bombings, GI injury occurs with the same frequency as lung injury (40) and at lower pressures following blasts in enclosed spaces (41). Victims of underwater explosions seem to be at a much greater risk for GI injury, especially if treading water or partially submerged (39).
Blast injury tends to affect the colon more often than the small bowel, owing to the greater amount of air in the former. Damage may range from edema to hemorrhage to frank rupture. Rupture is commonly delayed 24 to 48 hours, after stretching and ischemia have weakened the bowel wall (42). Tangential shear waves affecting the liver, spleen, and kidneys have been described, causing subcapsular petechiae, contusions, lacerations, or rupture (39,43). However, nonbowel or solid organ injuries seen following explosions are far more likely to occur from the effects of secondary or tertiary blast injury (8). Signs and symptoms of GI blast injury are nonspecific and include abdominal pain, nausea, vomiting, diarrhea, tenesmus, absent or decreased bowel sounds, rebound tenderness, guarding, or frank rectal bleeding.
AUDITORY BLAST INJURY
The ear is designed to amplify ambient sound waves, and the thin tympanic membrane is directly exposed to the surrounding air. It is not surprising that blast injury to the auditory system occurs much more commonly and at much lower overpressures than do pulmonary or GI blast injury. As a frame of reference, extremely loud acoustic waves, such as those generated at a concert, are generally less than 0.04 psi. Overpressures of at least 1 to 8 psi are needed to cause tympanic membrane rupture (44,45), whereas the threshold for lung injury is typically upward of 50 to 100 psi.
Blast waves are known to cause conductive, sensorineural, and mixed hearing defects. Conductive loss is usually caused by rupture of the tympanic membrane, although ossicular chain disruption may also be seen (24). Such defects are rarely found in isolation, however; most casualties will invariably suffer some degree of concomitant sensorineural hearing loss (46). Blast-related inner ear damage due to transient cochlear dysfunction can cause acute sensorineural hearing loss, often accompanied by tinnitus, which can be quite incapacitating in the moments following an explosion (24). Vertigo and balance disorders have also been described but are thought to be more likely secondary to blunt head injury than primary auditory damage (47).
Although tympanic membrane rupture is objective evidence of significant exposure to blast overpressure and was once thought to be an effective marker for occult pulmonary or GI blast injury, a recent Israeli study demonstrated no significant correlation. In fact, no patients with isolated tympanic membrane rupture later developed evidence of lung or GI pathology (48).
SECONDARY BLAST INJURY
Secondary blast injury, caused by shrapnel and displaced debris, is responsible for the majority of casualties resulting from an explosive event, partly because victims do not have to be close to the blast epicenter to be injured. In fact, blast winds channeled by an alleyway or corridor can be magnified substantially (49), causing significant secondary blast injury to those distant from the epicenter. Shrapnel injuries have been reported in 20% to 40% of blast victims, with an increased incidence in enclosed spaces (50). Injuries from all types of missiles have been described, although in the urban setting, glass has been the most common
hazard (9). Terrorists often deliberately pack small metal objects such as nails, bolts, and screws around an explosive charge in an attempt to increase shrapnel injuries; military shell casings are specifically designed to fragment to achieve a similar goal. These irregularly shaped missiles are generally of high mass and therefore high kinetic energy. The kinetic energy is dissipated rapidly along wound tracts to produce severe tissue destruction (51). Such wounds are usually heavily contaminated. At missile speeds of 15 meters per second (m/s), skin may be lacerated; at speeds of 120 m/s and above, body penetration and significant tissue damage can occur (9). A detonation causing a peak overpressure of 5 psi, just strong enough to rupture half of exposed tympanic membranes, may generate a blast wind of up to 70 m/s (156 mph) (6), a speed that, although not sustained, can exceed that of most hurricanes (52). A blast wind sufficient to cause primary lung injury in a significant number of casualties may exceed 400 m/s (895 mph) (6). Most conventional military explosives can propel fragments with initial velocities reaching 1,500 m/s (53). Secondary blast injuries were a significant cause of morbidity in the Oklahoma City bombing; the Murrah Building’s glass facade shattered and was propelled over an area of many city blocks. Similarly, in the 1998 U.S. embassy bombing in Nairobi, flying glass wounded people up to 2 km away (52).
hazard (9). Terrorists often deliberately pack small metal objects such as nails, bolts, and screws around an explosive charge in an attempt to increase shrapnel injuries; military shell casings are specifically designed to fragment to achieve a similar goal. These irregularly shaped missiles are generally of high mass and therefore high kinetic energy. The kinetic energy is dissipated rapidly along wound tracts to produce severe tissue destruction (51). Such wounds are usually heavily contaminated. At missile speeds of 15 meters per second (m/s), skin may be lacerated; at speeds of 120 m/s and above, body penetration and significant tissue damage can occur (9). A detonation causing a peak overpressure of 5 psi, just strong enough to rupture half of exposed tympanic membranes, may generate a blast wind of up to 70 m/s (156 mph) (6), a speed that, although not sustained, can exceed that of most hurricanes (52). A blast wind sufficient to cause primary lung injury in a significant number of casualties may exceed 400 m/s (895 mph) (6). Most conventional military explosives can propel fragments with initial velocities reaching 1,500 m/s (53). Secondary blast injuries were a significant cause of morbidity in the Oklahoma City bombing; the Murrah Building’s glass facade shattered and was propelled over an area of many city blocks. Similarly, in the 1998 U.S. embassy bombing in Nairobi, flying glass wounded people up to 2 km away (52).
TERTIARY BLAST INJURY
Tertiary blast injury is a feature of high-energy explosions and occurs when the individual becomes the missile. A high explosive blast wind may propel a 75 kilogram adult with an acceleration of close to 15 G’s. Typical patterns of blunt trauma and deceleration injury are seen when casualties are thrown against a solid object or the ground. Blunt head injury is a leading cause of death in blast victims. Tertiary blast injury accounted for most of the pediatric casualties in the Oklahoma City bombing because children are lighter and more easily displaced by blast wind. There was a high incidence of skull fractures, closed head injuries, and long-bone fractures.
QUATERNARY (MISCELLANEOUS) BLAST INJURY
A variety of other blast-related injuries have been described. Ocular injuries from flying debris are very common after explosions. Injury can be extensive and can involve all tissues of the eye, the ocular adnexa, and the orbit, including simple corneal abrasions, lid lacerations, global rupture, retinal detachment, intraocular foreign bodies, and orbital fractures. Retinal air embolism is also a potential cause of visual disturbance. A review of ocular injuries sustained by survivors of the 1995 Oklahoma City bombing found an 8% overall incidence of ocular injury, with 71% of injuries occurring within 300 feet of the point of detonation. The Murrah Building’s glass facade, shattered by the blast, accounted for two-thirds of all ocular injuries (54).
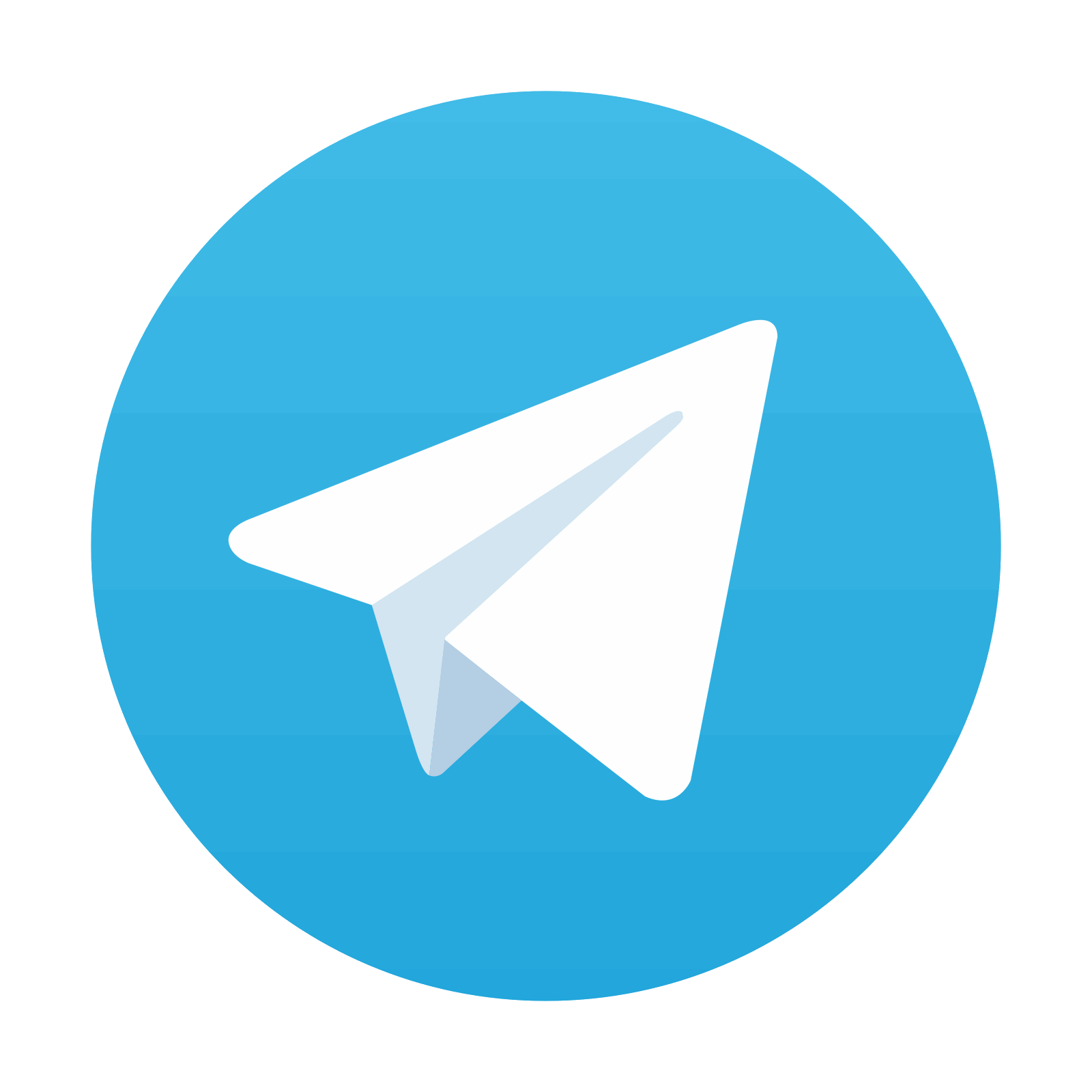
Stay updated, free articles. Join our Telegram channel

Full access? Get Clinical Tree
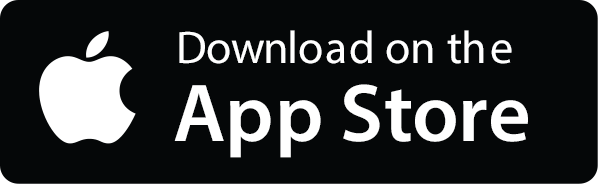
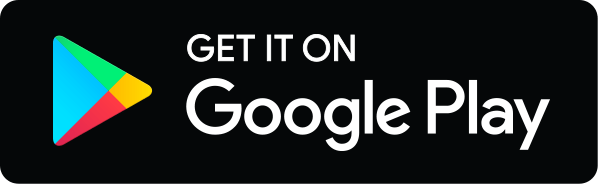