KEY POINTS
There has been a paradigm shift in the surgical management of Graves’ disease with increased use of total or near-total thyroidectomy, rather than subtotal thyroidectomy.
Familial nonmedullary thyroid cancer is increasingly being recognized as a separate entity. Surgeons must be aware of the potential for false-negative fine-needle aspiration biopsy in this setting.
Fine-needle aspiration biopsies are now classified into six groups based on the risk of malignancy associated with each group (Bethesda criteria).
Total thyroidectomy is the surgical treatment of choice for most thyroid cancers, provided complication rates are low.
Due to the limitations of fine-needle aspiration biopsy in the setting of indeterminate thyroid nodules, a number of molecular markers are being evaluated as adjuncts to refine the diagnosis and management of these patients.
Focused mini-incision parathyroidectomy, after appropriate localization, has become the procedure of choice for the treatment of sporadic primary hyperparathyroidism.
Parathyroidectomy has been shown to improve the classic and the so-called nonspecific symptoms and metabolic complications of primary hyperparathyroidism.
Normocalcemic hyperparathyroidism is being increasingly recognized; however, there are no definitive guidelines for management.
Very high calcium and parathyroid hormone levels in a patient with primary hyperparathyroidism should alert the surgeon to the presence of a possible parathyroid carcinoma.
Subclinical Cushing’s syndrome is characterized by subtle abnormalities in corticosteroid synthesis, and many of its manifestations appear to be treated by adrenalectomy.
Fine-needle aspiration biopsy has a very limited role in the evaluation of adrenal incidentalomas unless the patient has previously had a cancer and should only be performed after appropriate biochemical studies have been performed to rule out pheochromocytoma.
Laparoscopic adrenalectomy has become the procedure of choice for excision of most adrenal lesions, except known or suspected cancers.
THYROID
Goiters (from the Latin guttur, throat), defined as an enlargement of the thyroid, have been recognized since 2700 b.c. even though the thyroid gland was not documented as such until the Renaissance period. In 1619, Hieronymus Fabricius ab Aquapendente recognized that goiters arose from the thyroid gland. The term thyroid gland (Greek thyreoeides, shield-shaped) is, however, attributed to Thomas Wharton in his Adenographia (1656). In 1776, the thyroid was classified as a ductless gland by Albrecht von Haller and was thought to have numerous functions ranging from lubrication of the larynx to acting as a reservoir for blood to provide continuous flow to the brain, and to beautifying women’s necks. Burnt seaweed was considered to be the most effective treatment for goiters.
The first accounts of thyroid surgery for the treatment of goiters were given by Roger Frugardi in 1170. In response to failure of medical treatment, two setons were inserted at right angles into the goiter and tightened twice daily until the goiter separated. The open wound was treated with caustic powder and left to heal. However, thyroid surgery continued to be hazardous with prohibitive mortality rates (>40%) until the latter half of the nineteenth century, when advances in general anesthesia, antisepsis, and hemostasis enabled surgeons to perform thyroid surgery with significantly reduced mortality and morbidity rates. The most notable thyroid surgeons were Emil Theodor Kocher (1841–1917) and C.A. Theodor Billroth (1829–1894), who performed thousands of operations with increasingly successful results. However, as more patients survived thyroid operations, new problems and issues became apparent. After total thyroidectomy, patients (particularly children) became myxedematous with cretinous features. Myxedema was first effectively treated in 1891 by George Murray using a subcutaneous injection of an extract of sheep’s thyroid, and later, Edward Fox demonstrated that oral therapy was equally effective. In 1909, Kocher was awarded the Nobel Prize for medicine in recognition “for his works on the physiology, pathology, and surgery of the thyroid gland.”
The thyroid gland arises as an outpouching of the primitive foregut around the third week of gestation. It originates at the base of the tongue at the foramen cecum. Endoderm cells in the floor of the pharyngeal anlage thicken to form the medial thyroid anlage (Fig. 38-1) that descends in the neck anterior to structures that form the hyoid bone and larynx. During its descent, the anlage remains connected to the foramen cecum via an epithelial-lined tube known as the thyroglossal duct. The epithelial cells making up the anlage give rise to the thyroid follicular cells. The paired lateral anlages originate from the fourth branchial pouch and fuse with the median anlage at approximately the fifth week of gestation. The lateral anlages are neuroectodermal in origin (ultimobranchial bodies) and provide the calcitonin producing parafollicular or C cells, which thus come to lie in the superoposterior region of the gland. Thyroid follicles are initially apparent by 8 weeks, and colloid formation begins by the eleventh week of gestation.
Thyroglossal duct cysts are the most commonly encountered congenital cervical anomalies. During the fifth week of gestation, the thyroglossal duct lumen starts to obliterate, and the duct disappears by the eighth week of gestation. Rarely, the thyroglossal duct may persist in whole or in part. Thyroglossal duct cysts may occur anywhere along the migratory path of the thyroid, although 80% are found in juxtaposition to the hyoid bone. They are usually asymptomatic but occasionally become infected by oral bacteria, prompting the patient to seek medical advice. Thyroglossal duct sinuses result from infection of the cyst secondary to spontaneous or surgical drainage of the cyst and are accompanied by minor inflammation of the surrounding skin. Histologically, thyroglossal duct cysts are lined by pseudostratified ciliated columnar epithelium and squamous epithelium, with heterotopic thyroid tissue present in 20% of cases.
The diagnosis usually is established by observing a 1- to 2-cm, smooth, well-defined midline neck mass that moves upward with protrusion of the tongue. Routine thyroid imaging is not necessary, although thyroid scintigraphy and ultrasound have been performed to document the presence of normal thyroid tissue in the neck. Treatment involves the “Sistrunk operation,” which consists of en bloc cystectomy and excision of the central hyoid bone to minimize recurrence. Approximately 1% of thyroglossal duct cysts are found to contain cancer, which is usually papillary (85%). The role of total thyroidectomy in this setting is debated, but is advised in patients with large tumors, particularly if there are additional thyroid nodules and evidence of cyst wall invasion or lymph node metastases.1 Squamous, Hürthle cell, and anaplastic cancers also have been reported but are rare. Medullary thyroid cancers (MTCs) are, however, not found in thyroglossal duct cysts.
A lingual thyroid represents a failure of the median thyroid anlage to descend normally and may be the only thyroid tissue present. Intervention becomes necessary for obstructive symptoms such as choking, dysphagia, airway obstruction, or hemorrhage. Many of these patients develop hypothyroidism. Medical treatment options include administration of exogenous thyroid hormone to suppress thyroid-stimulating hormone (TSH) and radioactive iodine (RAI) ablation followed by hormone replacement. Surgical excision is rarely needed but, if required, should be preceded by an evaluation of normal thyroid tissue in the neck to avoid inadvertently rendering the patient hypothyroid.
Normal thyroid tissue may be found anywhere in the central neck compartment, including the esophagus, trachea, and anterior mediastinum. Thyroid tissue has been observed adjacent to the aortic arch, in the aortopulmonary window, within the upper pericardium, or in the interventricular septum. Often, “tongues” of thyroid tissue are seen to extend off the inferior poles of the gland and are particularly apparent in large goiters. Thyroid tissue situated lateral to the carotid sheath and jugular vein, previously termed lateral aberrant thyroid, almost always represents metastatic thyroid cancer in lymph nodes, and not remnants of the lateral anlage that had failed to fuse with the main thyroid, as previously suggested by Crile. Even if not readily apparent on physical examination or ultrasound imaging, the ipsilateral thyroid lobe contains a focus of papillary thyroid cancer (PTC), which may be microscopic.
Normally the thyroglossal duct atrophies, although it may remain as a fibrous band. In about 50% of individuals, the distal end that connects to the thyroid persists as a pyramidal lobe projecting up from the isthmus, lying just to the left or right of the midline. In the normal individual, the pyramidal lobe is not palpable, but in disorders resulting in thyroid hypertrophy (e.g., Graves’ disease, diffuse nodular goiter, or lymphocytic thyroiditis), the pyramidal lobe usually is enlarged and palpable.
The anatomic relations of the thyroid gland and surrounding structures are depicted in Fig. 38-2. The adult thyroid gland is brown in color and firm in consistency and is located posterior to the strap muscles. The normal thyroid gland weighs approximately 20 g, but gland weight varies with body weight and iodine intake. The thyroid lobes are located adjacent to the thyroid cartilage and connected in the midline by an isthmus that is located just inferior to the cricoid cartilage. A pyramidal lobe is present in about 50% of patients. The thyroid lobes extend to the midthyroid cartilage superiorly and lie adjacent to the carotid sheaths and sternocleidomastoid muscles laterally. The strap muscles (sternohyoid, sternothyroid, and superior belly of the omohyoid) are located anteriorly and are innervated by the ansa cervicalis (ansa hypoglossi). The thyroid gland is enveloped by a loosely connecting fascia that is formed from the partition of the deep cervical fascia into anterior and posterior divisions. The true capsule of the thyroid is a thin, densely adherent fibrous layer that sends out septa that invaginate into the gland, forming pseudolobules. The thyroid capsule is condensed into the posterior suspensory or Berry’s ligament near the cricoid cartilage and upper tracheal rings.
The superior thyroid arteries arise from the ipsilateral external carotid arteries and divide into anterior and posterior branches at the apices of the thyroid lobes. The inferior thyroid arteries arise from the thyrocervical trunk shortly after their origin from the subclavian arteries. The inferior thyroid arteries travel upward in the neck posterior to the carotid sheath to enter the thyroid lobes at their midpoint. A thyroidea ima artery arises directly from the aorta or innominate in 1% to 4% of individuals to enter the isthmus or replace a missing inferior thyroid artery. The inferior thyroid artery crosses the recurrent laryngeal nerve (RLN), necessitating identification of the RLN before the arterial branches can be ligated. The venous drainage of the thyroid gland occurs via multiple small surface veins, which coalesce to form three sets of veins—the superior, middle, and inferior thyroid veins. The superior thyroid veins run with the superior thyroid arteries bilaterally. The middle vein or veins are the least consistent. The superior and middle veins drain directly into the internal jugular veins. The inferior veins often form a plexus, which drains into the brachiocephalic veins.
The left RLN arises from the vagus nerve where it crosses the aortic arch, loops around the ligamentum arteriosum, and ascends medially in the neck within the tracheoesophageal groove. The right RLN arises from the vagus at its crossing with the right subclavian artery. The nerve usually passes posterior to the artery before ascending in the neck, its course being more oblique than the left RLN. Along their course in the neck, the RLNs may branch, and pass anterior, posterior, or interdigitate with branches of the inferior thyroid artery (Fig. 38-3). The right RLN may be nonrecurrent in 0.5% to 1% of individuals and often is associated with a vascular anomaly. Nonrecurrent left RLNs are rare but have been reported in patients with situs inversus and a right-sided aortic arch. The RLN may branch in its course in the neck, and identification of a small nerve should alert the surgeon to this possibility. Identification of the nerves or their branches often necessitates mobilization of the most lateral and posterior extent of the thyroid gland, the tubercle of Zuckerkandl, at the level of the cricoid cartilage. The last segments of the nerves often course below the tubercle and are closely approximated to the ligament of Berry. Branches of the nerve may traverse the ligament in 25% of individuals and are particularly vulnerable to injury at this junction. The RLNs terminate by entering the larynx posterior to the cricothyroid muscle.
The RLNs innervate all the intrinsic muscles of the larynx, except the cricothyroid muscles, which are innervated by the external laryngeal nerves. Injury to one RLN leads to paralysis of the ipsilateral vocal cord, which comes to lie in the paramedian or the abducted position. The paramedian position results in a normal but weak voice, whereas the abducted position leads to a hoarse voice and an ineffective cough. Bilateral RLN injury may lead to airway obstruction, necessitating emergency tracheostomy, or loss of voice. If both cords come to lie in an abducted position, air movement can occur, but the patient has an ineffective cough and is at increased risk of repeated respiratory tract infections from aspiration.
The superior laryngeal nerves also arise from the vagus nerves. After their origin at the base of the skull, these nerves travel along the internal carotid artery and divide into two branches at the level of the hyoid bone. The internal branch of the superior laryngeal nerve is sensory to the supraglottic larynx. Injury to this nerve is rare in thyroid surgery, but its occurrence may result in aspiration. The external branch of the superior laryngeal nerve lies on the inferior pharyngeal constrictor muscle and descends alongside the superior thyroid vessels before innervating the cricothyroid muscle. Cernea and colleagues2 proposed a classification system to describe the relationship of this nerve to the superior thyroid vessels (Fig. 38-4). The type 2a variant, in which the nerve crosses below the tip of the thyroid superior pole, occurs in up to 20% of individuals and places the nerve at a greater risk of injury. Therefore, the superior pole vessels should not be ligated en masse, but should be individually divided, low on the thyroid gland and dissected lateral to the cricothyroid muscle. Injury to this nerve leads to inability to tense the ipsilateral vocal cord and hence difficulty “hitting high notes,” difficulty projecting the voice, and voice fatigue during prolonged speech.
Figure 38-4.
Relationship of the external branch of the superior laryngeal nerve and superior thyroid artery originally described by Cernea and colleagues.2 In type 1 anatomy, the nerve crosses the artery ≥1 cm above the superior aspect of the thyroid lobe. In type 2 anatomy, the nerve crosses the artery <1 cm above the thyroid pole (2a) or below (2b) it. (Reproduced with permission from Bliss RD et al. Surgeon’s approach to the thyroid gland: Surgical anatomy and the importance of technique. World J Surg. 2000;24:893. With kind permission of Springer Science + Business Media.)
Sympathetic innervation of the thyroid gland is provided by fibers from the superior and middle cervical sympathetic ganglia. The fibers enter the gland with the blood vessels and are vasomotor in action. Parasympathetic fibers are derived from the vagus nerve and reach the gland via branches of the laryngeal nerves.
The embryology and anatomy of the parathyroid glands are discussed in detail in the Parathyroid Gland section of this chapter. About 85% of individuals have four parathyroid glands that can be found within 1 cm of the junction of the inferior thyroid artery and the RLN. The superior glands are usually located dorsal to the RLN, whereas the inferior glands are usually found ventral to the RLN (Fig. 38-5).
The thyroid gland is endowed with an extensive network of lymphatics. Intraglandular lymphatic vessels connect both thyroid lobes through the isthmus and also drain to perithyroidal structures and lymph nodes. Regional lymph nodes include pretracheal, paratracheal, perithyroidal, RLN, superior mediastinal, retropharyngeal, esophageal, and upper, middle, and lower jugular chain nodes. These lymph nodes can be classified into seven levels as depicted in Fig. 38-6. The central compartment includes nodes located in the area between the two carotid sheaths, whereas nodes lateral to the vessels are present in the lateral compartment. Thyroid cancers may metastasize to any of these regions, although metastases to submaxillary nodes (level I) are rare (<1%). There also can be “skip” metastases to nodes in the lateral ipsilateral neck without central neck nodes.
Microscopically, the thyroid is divided into lobules that contain 20 to 40 follicles (Fig. 38-7). There are about 3 × 106 follicles in the adult male thyroid gland. The follicles are spherical and average 30 μm in diameter. Each follicle is lined by cuboidal epithelial cells and contains a central store of colloid secreted from the epithelial cells under the influence of the pituitary hormone TSH. The second group of thyroid secretory cells is the C cells or parafollicular cells, which contain and secrete the hormone calcitonin. They are found as individual cells or clumped in small groups in the interfollicular stroma and located in the upper poles of the thyroid lobes.
The average daily iodine requirement is 0.1 mg, which can be derived from foods such as fish, milk, and eggs or as additives in bread or salt. In the stomach and jejunum, iodine is rapidly converted to iodide and absorbed into the bloodstream, and from there it is distributed uniformly throughout the extracellular space. Iodide is actively transported into the thyroid follicular cells by an adenosine triphosphate (ATP)–dependent process. The thyroid is the storage site of >90% of the body’s iodine content and accounts for one third of the plasma iodine loss. The remaining plasma iodine is cleared via renal excretion.
The synthesis of thyroid hormone consists of several steps (Fig. 38-8). The first, iodide trapping, involves active (ATP-dependent) transport of iodide across the basement membrane of the thyrocyte via an intrinsic membrane protein, the sodium/iodine (Na+/I–) symporter. Thyroglobulin (Tg) is a large (660 kDa) glycoprotein, which is present in thyroid follicles and has four tyrosyl residues. The second step in thyroid hormone synthesis involves oxidation of iodide to iodine and iodination of tyrosine residues on Tg, to form monoiodotyrosines (MIT) and diiodotyrosines (DIT). Both processes are catalyzed by thyroid peroxidase (TPO). A recently identified protein, pendrin, is thought to mediate iodine efflux at the apical membrane. The third step leads to coupling of two DIT molecules to form tetra-iodothyronine or thyroxine (T4), and one DIT molecule with one MIT molecule to form 3,5,3′-triiodothyronine (T3) or 3,3′,5′-triiodothyronine reverse (rT3). When stimulated by TSH, thyrocytes form pseudopodia, which encircle portions of cell membrane containing Tg, which in turn, fuse with enzyme-containing lysosomes. In the fourth step, Tg is hydrolyzed to release free iodothyronines (T3 and T4) and mono- and diiodotyrosines. The latter are deiodinated in the fifth step to yield iodide, which is reused in the thyrocyte. In the euthyroid state, T4 is produced and released entirely by the thyroid gland, whereas only 20% of the total T3 is produced by the thyroid. Most of the T3 is produced by peripheral deiodination (removal of 5′-iodine from the outer ring) of T4 in the liver, muscles, kidney, and anterior pituitary, a reaction that is catalyzed by 5′-mono-deiodinase. Some T4 is converted to rT3, the metabolically inactive compound, by deiodination of the inner ring of T4. In conditions such as Graves’ disease, toxic multinodular goiter, or a stimulated thyroid gland, the proportion of T3 released from the thyroid may be dramatically elevated. Thyroid hormones are transported in serum bound to carrier proteins such as T4-binding globulin, T4-binding prealbumin, and albumin. Only a small fraction (0.02%) of thyroid hormone (T3 and T4) is free (unbound) and is the physiologically active component. T3 is the more potent of the two thyroid hormones, although its circulating plasma level is much lower than that of T4. T3 is less tightly bound to protein in the plasma than T4, and so it enters tissues more readily. T3 is three to four times more active than T4 per unit weight, with a half-life of about 1 day, compared to approximately 7 days for T4.
Figure 38-8.
Thyroid follicular cell showing the major signaling pathways involved in thyroid cell growth and function and key steps in thyroid hormone synthesis. The basal membrane of the cell in contact with the circulation and its apical surface contact the thyroid follicle. Thyroid hormone synthesis is initiated by the binding of thyroid-stimulating hormone (TSH) to the TSH receptor (TSHR), a G-protein–coupled transmembrane receptor, on the basal membrane. Activation leads to an increase in cyclic adenosine monophosphate (cAMP), phosphorylation of protein kinase A (PKA), and activation of target cytosolic and nuclear proteins. The protein kinase C (PKC) pathway is stimulated at higher doses of TSH. Iodide is actively transported into the cell via the Na/I symporter (NIS) and flows down an electrical gradient to the apical membrane. There, thyroid peroxidase (TPO) oxidizes iodide and iodinated tyrosyl residues on thyroglobulin (Tg) in the presence of peroxide (H2O2). Mono- and diiodotyrosyl (MIT, DIT) residues are also coupled to form T4 and T3 by TPO. Thyroglobulin carrying T4 and T3 is then internalized by pinocytosis and digested in lysosomes. Thyroid hormone is released into the circulation, while MIT and DIT are deiodinated and recycled. ATP = adenosine triphosphate; CREB = cAMP response element binding protein; CREM = cAMP response element modulator; DAG = diacylglycerol; IGF-1 = insulin-like growth factor 1; IP3 = inositol-3-phosphate; NADP+ = nicotinamide adenine dinucleotide phosphate, oxidized form; NADPH = nicotinamide adenine dinucleotide phosphate; PIP2 = phosphatidylinositol; PLC = phospholipase C; T3 = 3,5′,3-triiodothyronine; T4 = thyroxine. (Reproduced with permission from Kopp P. Pendred’s syndrome and genetic defects in thyroid hormone synthesis. Rev Endocr Metab Disord. 2000;1:114. Kluwer Academic Publishers.)
The secretion of thyroid hormone is controlled by the hypothalamic-pituitary-thyroid axis (Fig. 38-9). The hypothalamus produces a peptide, the thyrotropin-releasing hormone (TRH), which stimulates the pituitary to release TSH or thyrotropin. TRH reaches the pituitary via the portovenous circulation. TSH, a 28-kDa glycopeptide, mediates iodide trapping, secretion, and release of thyroid hormones, in addition to increasing the cellularity and vascularity of the thyroid gland. The TSH receptor (TSH-R) belongs to a family of G-protein–coupled receptors that have seven transmembrane-spanning domains and use cyclic adenosine monophosphate in the signal-transduction pathway. TSH secretion by the anterior pituitary is also regulated via a negative feedback loop by T4 and T3. Because the pituitary has the ability to convert T4 to T3, the latter is thought to be more important in this feedback control. T3 also inhibits the release of TRH.
Figure 38-9.
Hypothalamic-pituitary-thyroid hormone axis. In both the hypothalamus and pituitary, 3,5′,3-triiodothyronine (T3) is primarily responsible for inhibition of thyrotropin-releasing hormone (TRH) and thyroid-stimulating hormone (TSH) secretion. T4 = thyroxine. (Reproduced with permission from Greenspan FS. The thyroid gland. In: Greenspan FS, Gardner D, eds. Basic and Clinical Endocrinology. 6th ed. New York: McGraw-Hill; 2001:217. Copyright © The McGraw-Hill Companies, Inc.)
The thyroid gland also is capable of autoregulation, which allows it to modify its function independent of TSH. As an adaptation to low iodide intake, the gland preferentially synthesizes T3 rather than T4, thereby increasing the efficiency of secreted hormone. In situations of iodine excess, iodide transport, peroxide generation, and synthesis and secretion of thyroid hormones are inhibited. Excessively large doses of iodide may lead to initial increased organification, followed by suppression, a phenomenon called the Wolff-Chaikoff effect. Epinephrine and human chorionic gonadotropin hormones stimulate thyroid hormone production. Thus, elevated thyroid hormone levels are found in pregnancy and gynecologic malignancies such as hydatidiform mole. In contrast, glucocorticoids inhibit thyroid hormone production. In severely ill patients, peripheral thyroid hormones may be reduced, without a compensatory increase in TSH levels, giving rise to the euthyroid sick syndrome.
Free thyroid hormone enters the cell membrane by diffusion or by specific carriers and is carried to the nuclear membrane by binding to specific proteins. T4 is deiodinated to T3 and enters the nucleus via active transport, where it binds to the thyroid hormone receptor. The T3 receptor is similar to the nuclear receptors for glucocorticoids, mineralocorticoids, estrogens, vitamin D, and retinoic acid. In humans, two types of T3 receptor genes (α and β) are located on chromosomes 3 and 17. Thyroid receptor expression depends on peripheral concentrations of thyroid hormones and is tissue specific—the α form is abundant in the central nervous system, whereas the β form predominates in the liver. Each gene product has a ligand-independent, amino-terminal domain; a ligand-binding, carboxy-terminal domain; and centrally located DNA-binding regions. Binding of thyroid hormone leads to the transcription and translation of specific hormone-responsive genes.
Thyroid hormones affect almost every system in the body. They are important for fetal brain development and skeletal maturation. T3 increases oxygen consumption, basal metabolic rate, and heat production by stimulation of Na+/K+ ATPase in various tissues. It also has positive inotropic and chronotropic effects on the heart by increasing transcription of the Ca2+ ATPase in the sarcoplasmic reticulum and increasing levels of β-adrenergic receptors and concentration of G proteins. Myocardial α receptors are decreased, and actions of catecholamines are amplified. Thyroid hormones are responsible for maintaining the normal hypoxic and hypercapnic drive in the respiratory center of the brain. They also increase gastrointestinal (GI) motility, leading to diarrhea in hyperthyroidism and constipation in hypothyroidism. Thyroid hormones also increase bone and protein turnover and the speed of muscle contraction and relaxation. They also increase glycogenolysis, hepatic gluconeogenesis, intestinal glucose absorption, and cholesterol synthesis and degradation.
A multitude of different tests are available to evaluate thyroid function. No single test is sufficient to assess thyroid function in all situations, and the results must be interpreted in the context of the patient’s clinical condition. TSH is the only test necessary in most patients with thyroid nodules that clinically appear to be euthyroid.
The tests for serum TSH are based on the following principle: monoclonal TSH antibodies are bound to a solid matrix and bind serum TSH. A second monoclonal antibody binds to a separate epitope on TSH and is labeled with radioisotope, enzyme, or fluorescent tag. Therefore, the amount of serum TSH is proportional to the amount of bound secondary antibody (immunometric assay). Serum TSH levels reflect the ability of the anterior pituitary to detect free T4 levels. There is an inverse relationship between the free T4 level and the logarithm of the TSH concentration—small changes in free T4 lead to a large shift in TSH levels. The ultrasensitive TSH assay has become the most sensitive and specific test for the diagnosis of hyper- and hypothyroidism and for optimizing T4 therapy.
Total T4 and T3 levels are measured by radioimmunoassay and measure both the free and bound components of the hormones. Total T4 levels reflect the output from the thyroid gland, whereas T3 levels in the nonstimulated thyroid gland are more indicative of peripheral thyroid hormone metabolism, and are, therefore, not generally suitable as a general screening test. Total T4 levels are increased not only in hyperthyroid patients, but also in those with elevated Tg levels secondary to pregnancy, estrogen/progesterone use, or congenital diseases. Similarly, total T4 levels decrease in hypothyroidism and in patients with decreased Tg levels due to anabolic steroid use and protein-losing disorders like nephrotic syndrome. Individuals with these latter disorders may be euthyroid if their free T4 levels are normal. Measurement of total T3 levels is important in clinically hyperthyroid patients with normal T4 levels, who may have T3 thyrotoxicosis. As discussed previously in Thyroid Hormone Synthesis, Secretion, and Transport, total T3 levels often are increased in early hypothyroidism.
These radioimmunoassay-based tests are a sensitive and accurate measurement of biologically active thyroid hormone. Free T4 estimates are not performed as a routine screening tool in thyroid disease. Use of this test is confined to cases of early hyperthyroidism in which total T4 levels may be normal but free T4 levels are raised. In patients with end-organ resistance to T4 (Refetoff’s syndrome), T4 levels are increased, but TSH levels usually are normal. Free T3 is most useful in confirming the diagnosis of early hyperthyroidism, in which levels of free T4 and free T3 rise before total T4 and T3. Free T4 levels may also be measured indirectly using the T3-resin uptake test. If free T4 levels are increased, fewer hormone binding sites are available for binding radiolabeled T3 that has been added to the patient’s serum. Therefore, more T3 binds with an ion-exchange resin, and the T3-resin uptake is increased.
This test is useful to evaluate pituitary TSH secretory function and is performed by administering 500 μg of TRH intravenously and measuring TSH levels after 30 and 60 minutes. In a normal individual, TSH levels should increase at least 6 μIU/mL from the baseline. This test also was previously used to assess patients with borderline hyperthyroidism but has largely been replaced by sensitive TSH assays for this purpose.
Thyroid antibodies include anti-Tg, antimicrosomal, or anti-TPO and thyroid-stimulating immunoglobulin (TSI). Anti-Tg and anti-TPO antibody levels do not determine thyroid function, but rather indicate the underlying disorder, usually an autoimmune thyroiditis. About 80% of patients with Hashimoto’s thyroiditis have elevated thyroid antibody levels; however, levels may also be increased in patients with Graves’ disease, multinodular goiter, and occasionally, thyroid neoplasms.
Tg is only made by normal or abnormal thyroid tissue. It normally is not released into the circulation in large amounts but increases dramatically in destructive processes of the thyroid gland, such as thyroiditis, or overactive states such as Graves’ disease and toxic multinodular goiter. The most important use for serum Tg levels is in monitoring patients with differentiated thyroid cancer for recurrence, particularly after total thyroidectomy and RAI ablation. Elevated anti-Tg antibodies can interfere with the accuracy of serum Tg levels and should always be measured when interpreting Tg levels.
This 32-amino-acid polypeptide is secreted by the C cells and functions to lower serum calcium levels, although in humans, it has only minimal physiologic effects. It is also a sensitive marker of MTC.
Both iodine-123 (123I) and iodine-131 (131I) are used to image the thyroid gland. The former emits low-dose radiation, has a half-life of 12 to 14 hours, and is used to image lingual thyroids or goiters. In contrast, 131I has a half-life of 8 to 10 days and leads to higher-dose radiation exposure. Therefore, this isotope is used to screen and treat patients with differentiated thyroid cancers for metastatic disease. The images obtained by these studies provide information not only about the size and shape of the gland, but also the distribution of functional activity. Areas that trap less radioactivity than the surrounding gland are termed cold (Fig. 38-10), whereas areas that demonstrate increased activity are termed hot. The risk of malignancy is higher in “cold” lesions (20%) compared to “hot” or “warm” lesions (<5%). Technetium Tc 99m pertechnetate (99mTc) is taken up by the thyroid gland and is increasingly being used for thyroid evaluation. This isotope is taken up by the mitochondria, but is not organified. It also has the advantage of having a shorter half-life and minimizes radiation exposure. It is particularly sensitive for nodal metastases. More recently, 18F-fluorodeoxyglucose (FDG) positron emission tomography (PET) combined with computed tomography (CT) is being increasingly used to screen for metastases in patients with thyroid cancer in whom other imaging studies are negative. PET scans are not routinely used in the evaluation of thyroid nodules; however, they may show clinically occult thyroid lesions. There are several recent reports of rates of malignancy in these lesions ranging from 14% to 63%. These incidentally discovered nodules should be worked up by ultrasound and fine-needle aspiration biopsy (FNAB).
Ultrasound is an excellent noninvasive and portable imaging study of the thyroid gland with the added advantage of no radiation exposure. It is helpful in the evaluation of thyroid nodules, distinguishing solid from cystic ones, and providing information about size and multicentricity. In addition, characteristics such as echotexture, shape, borders and presence of calcifications, and vascularity can provide useful information regarding risk of malignancy. Ultrasound is also especially helpful for assessing cervical lymphadenopathy (Fig. 38-11) and to guide FNAB. An experienced ultrasonographer is necessary for the best results.
CT and magnetic resonance imaging (MRI) studies provide excellent imaging of the thyroid gland and adjacent nodes and are particularly useful in evaluating the extent of large, fixed, or substernal goiters (which cannot be evaluated by ultrasound) and their relationship to the airway and vascular structures. Noncontrast CT scans should be obtained for patients who are likely to require subsequent RAI therapy. If contrast is necessary, therapy needs to be delayed by several months. Combined PET-CT scans are increasingly being used for Tg-positive, RAI-negative tumors.
The clinical manifestations of hyperthyroidism result from an excess of circulating thyroid hormone. Hyperthyroidism may arise from a number of conditions that are listed in Table 38-1. It is important to distinguish disorders such as Graves’ disease and toxic nodular goiters that result from increased production of thyroid hormone from those disorders that lead to a release of stored hormone from injury to the thyroid gland (thyroiditis) or from other nonthyroid gland–related conditions. The former disorders lead to an increase in RAI uptake (RAIU), whereas the latter group is characterized by low RAIU. Of these disorders, Graves’ disease, toxic multinodular goiter, and solitary toxic nodule are most relevant to the surgeon.
INCREASED HORMONE SYNTHESIS (INCREASED RAIU) | RELEASE OF PREFORMED HORMONE (DECREASED RAIU) |
---|---|
Graves’ disease (diffuse toxic goiter) Toxic multinodular goiter Toxic adenoma Drug induced—amiodarone, iodine Thyroid cancer Struma ovarii Hydatidiform mole TSH-secreting pituitary adenoma | Thyroiditis—acute phase of Hashimoto’s thyroiditis, subacute thyroiditis Factitious (iatrogenic) thyrotoxicosis “Hamburger thyrotoxicosis” |
Although originally described by the Welsh physician Caleb Parry in a posthumous article in 1825, this disorder is known as Graves’ disease after Robert Graves, an Irish physician who described three patients in 1835. Graves’ disease is by far the most common cause of hyperthyroidism in North America, accounting for 60% to 80% of cases. It is an autoimmune disease with a strong familial predisposition, female preponderance (5:1), and peak incidence between the ages of 40 and 60 years. Graves’ disease is characterized by thyrotoxicosis, diffuse goiter, and extrathyroidal conditions including ophthalmopathy, dermopathy (pretibial myxedema), thyroid acropachy, gynecomastia, and other manifestations.
The exact etiology of the initiation of the autoimmune process in Graves’ disease is not known. However, conditions such as the postpartum state, iodine excess, lithium therapy, and bacterial and viral infections have been suggested as possible triggers. Genetic factors also play a role, as haplotyping studies indicate that Graves’ disease is associated with certain human leukocyte antigen (HLA) haplotypes, including HLA-B8, HLA-DR3, and HLADQA1*0501 in Caucasian patients, whereas HLA-DRB1*0701 is protective against it. Polymorphisms of the cytotoxic T-lymphocyte antigen 4 (CTLA-4) gene also have been associated with Graves’ disease development.3 Once initiated, the process causes sensitized T-helper lymphocytes to stimulate B lymphocytes, which produce antibodies directed against the thyroid hormone receptor. TSIs or antibodies that stimulate the TSH-R, as well as TSH-binding inhibiting immunoglobulins or antibodies, have been described. The thyroid-stimulating antibodies stimulate the thyrocytes to grow and synthesize excess thyroid hormone, which is a hallmark of Graves’ disease. Graves’ disease also is associated with other autoimmune conditions such as type 1 diabetes mellitus, Addison’s disease, pernicious anemia, and myasthenia gravis.
Macroscopically, the thyroid gland in patients with Graves’ disease is diffusely and smoothly enlarged, with a concomitant increase in vascularity. Microscopically, the gland is hyperplastic, and the epithelium is columnar with minimal colloid present. The nuclei exhibit mitosis, and papillary projections of hyperplastic epithelium are common. There may be aggregates of lymphoid tissue, and vascularity is markedly increased.
The clinical manifestations of Graves’ disease can be divided into those related to hyperthyroidism and those specific to Graves’ disease. Hyperthyroid symptoms include heat intolerance, increased sweating and thirst, and weight loss despite adequate caloric intake. Symptoms of increased adrenergic stimulation include palpitations, nervousness, fatigue, emotional lability, hyperkinesis, and tremors. The most common GI symptoms include increased frequency of bowel movements and diarrhea. Female patients often develop amenorrhea, decreased fertility, and an increased incidence of miscarriages. Children experience rapid growth with early bone maturation, whereas older patients may present with cardiovascular complications such as atrial fibrillation and congestive heart failure.
On physical examination, weight loss and facial flushing may be evident. The skin is warm and moist, and African American patients often note darkening of their skin. Tachycardia or atrial fibrillation is present, with cutaneous vasodilation leading to a widening of the pulse pressure and a rapid falloff in the transmitted pulse wave (collapsing pulse). A fine tremor, muscle wasting, and proximal muscle group weakness with hyperactive tendon reflexes often are present.
Approximately 50% of patients with Graves’ disease also develop clinically evident ophthalmopathy, and dermopathy occurs in 1% to 2% of patients. It is characterized by deposition of glycosaminoglycans, leading to thickened skin in the pretibial region and dorsum of the foot (Fig. 38-12). Eye symptoms include lid lag (von Graefe’s sign), spasm of the upper eyelid revealing the sclera above the corneoscleral limbus (Dalrymple’s sign), and a prominent stare, due to catecholamine excess. True infiltrative eye disease results in periorbital edema, conjunctival swelling and congestion (chemosis), proptosis, limitation of upward and lateral gaze (from involvement of the inferior and medial rectus muscles, respectively), keratitis, and even blindness due to optic nerve involvement. The etiology of Graves’ ophthalmopathy is not completely known; however, orbital fibroblasts and muscles are thought to share a common antigen, the TSH-R. Ophthalmopathy is thought to result from inflammation caused by cytokines released from sensitized killer T lymphocytes and cytotoxic antibodies. Gynecomastia is common in young men. Rare bony involvement leads to subperiosteal bone formation and swelling in the metacarpals (thyroid acropachy). Onycholysis, or separation of fingernails from their beds, is a commonly observed finding. On physical examination, the thyroid usually is diffusely and symmetrically enlarged, as evidenced by an enlarged pyramidal lobe. There may be an overlying bruit or thrill over the thyroid gland and a loud venous hum in the supraclavicular space.
The diagnosis of hyperthyroidism is made by a suppressed TSH with or without an elevated free T4 or T3 level. If eye signs are present, other tests are generally not needed. However, in the absence of eye findings, an 123I uptake and scan should be performed. An elevated uptake, with a diffusely enlarged gland, confirms the diagnosis of Graves’ disease and helps to differentiate it from other causes of hyperthyroidism. Technetium scintigraphy (using pertechnetate, which is trapped by the thyroid, but not organified) can also be used to determine etiology. While technetium scans results in low range of normal uptake and high background activity, total-body radiation exposure is less than that of 123I scans. If free T4 levels are normal, free T3 levels should be determined, as they often are elevated in early Graves’ disease or toxic nodules (T3 toxicosis). Anti-Tg and anti-TPO antibodies are elevated in up to 75% of patients but are not specific. Elevated TSH-R or thyroid-stimulating antibodies (TSAb) are diagnostic of Graves’ disease and are increased in about 90% of patients. MRI scans of the orbits are useful in evaluating Graves’ ophthalmopathy.
Graves’ disease may be treated by any of three treatment modalities: antithyroid drugs, thyroid ablation with radioactive 131I, and thyroidectomy. The choice of treatment depends on several factors, as discussed in the following sections.
Antithyroid medications generally are administered in preparation for RAI ablation or surgery. The drugs commonly used are propylthiouracil (PTU, 100 to 300 mg three times daily) and methimazole (10 to 30 mg three times daily, then once daily). Methimazole has a longer half-life and can be dosed once daily. Both drugs reduce thyroid hormone production by inhibiting the organic binding of iodine and the coupling of iodotyrosines (mediated by TPO). In addition, PTU also inhibits the peripheral conversion of T4 to T3, making it useful for the treatment of thyroid storm. Both drugs can cross the placenta, inhibiting fetal thyroid function, and are excreted in breast milk, although PTU has a lower risk of transplacental transfer. Methimazole also has been associated with congenital aplasia; therefore, PTU is preferred in pregnant and breastfeeding women. Side effects of treatment include reversible granulocytopenia, skin rashes, fever, peripheral neuritis, polyarteritis, vasculitis, hepatitis, and, rarely, agranulocytosis and aplastic anemia. Patients should be monitored for these possible complications and should always be warned to stop PTU or methimazole immediately and seek medical advice should they develop a sore throat or fever. Treatment of agranulocytosis involves admission to the hospital, discontinuation of the drug, and broad-spectrum antibiotic therapy. Surgery should be postponed until the granulocyte count reaches 1000 cells/mm3.
The dose of antithyroid medication is titrated as needed in accordance with TSH and T4 levels. Most patients have improved symptoms in 2 weeks and become euthyroid in about 6 weeks. Some physicians use the block-replace regimen, by adding T4 (0.05 to 0.10 mg) to prevent hypothyroidism and suppress TSH secretion, because some, but not all, studies suggest that this reduces recurrence rates. The length of therapy is debated. Treatment with antithyroid medications is associated with a high relapse rate when these drugs are discontinued, with 40% to 80% of patients developing recurrent disease after a 1- to 2-year course. Patients with small glands are less likely to recur so that treatment for curative intent is reserved for patients with (a) small, nontoxic goiters less than 40 g; (b) mildly elevated thyroid hormone levels; (c) negative or low or titers of thyroid hormone receptor antibodies; and (d) rapid decrease in gland size with antithyroid medications. The catecholamine response of thyrotoxicosis can be alleviated by administering β-blocking agents. β-Blockade should be considered in all patients with symptomatic thyrotoxicosis and is recommended for elderly patients, those with coexistent cardiac disease, and patients with resting heart rates >90 bpm. These drugs have the added effect of decreasing the peripheral conversion of T4 to T3. Propranolol is the most commonly prescribed medication in doses of about 20 to 40 mg four times daily. Higher doses are sometimes required due to increased clearance of the medication. Caution should be exercised in patients with asthma. Calcium channel blockers are useful for rate control in patients in whom β-blockers are contraindicated.
RAI forms the mainstay of Graves’ disease treatment in North America. The major advantages of this treatment are the avoidance of a surgical procedure and its concomitant risks, reduced overall treatment costs, and ease of treatment. Antithyroid drugs are given until the patient is euthyroid and then discontinued to maximize drug uptake. The 131I dose is calculated after a preliminary scan and usually consists of 8 to 12 mCi administered orally. After standard treatment with RAI, most patients become euthyroid within 2 months. However, only about 50% of patients treated with RAI are euthyroid 6 months after treatment, and the remaining are still hyperthyroid or already hypothyroid.4 After 1 year, about 2.5% of patients develop hypothyroidism each year. RAI also has been documented to lead to progression of Graves’ ophthalmopathy (33% after RAI compared to 16% after surgery), and ophthalmopathy is more common in smokers. Although there is no evidence of long-term problems with infertility, and overall cancer incidence rates are unchanged, there is a small increased risk of nodular goiter, thyroid cancer,5 and hyperparathyroidism (HPT)6 in patients who have been treated with RAI. Patients treated with RAI have an unexplained increase in their overall and cardiovascular mortality rates when compared to the general population.
RAI therapy is therefore most often used in older patients with small or moderate-sized goiters, those who have relapsed after medical or surgical therapy, and those in whom antithyroid drugs or surgery are contraindicated. Absolute contraindications to RAI include women who are pregnant (or planning pregnancy within 6 months of treatment) or breastfeeding. Relative contraindications include young patients (i.e., especially children and adolescents), those with thyroid nodules, and those with ophthalmopathy. Lack of access to a high-volume thyroid surgeon is also a consideration. The higher the initial dose of 131I, the earlier the onset and the higher the incidence of hypothyroidism.
In North America, surgery is recommended when RAI is contraindicated as in patients who (a) have confirmed cancer or suspicious thyroid nodules, (b) are young, (c) desire to conceive soon (<6 months) after treatment, (d) have had severe reactions to antithyroid medications, (e) have large goiters (>80 g) causing compressive symptoms, and (f) are reluctant to undergo RAI therapy. Relative indications for thyroidectomy include patients, particularly smokers, with moderate to severe Graves’ ophthalmopathy, those desiring rapid control of hyperthyroidism with a chance of being euthyroid, and those demonstrating poor compliance to antithyroid medications. Pregnancy is also a relative contraindication, and surgery should be used only when rapid control is needed and antithyroid medications cannot be used. Surgery is best performed in the second trimester. The goal of thyroidectomy for Graves’ disease should be the complete and permanent control of the disease with minimal morbidity. Patients should be rendered euthyroid before operation with antithyroid drugs that should be continued up to the day of surgery. Lugol’s iodide solution or saturated potassium iodide generally is administered beginning 7 to 10 days preoperatively (three drops twice daily) to reduce vascularity of the gland and decrease the risk of precipitating thyroid storm. The major action of iodine in this situation is to inhibit release of thyroid hormone. If it is not possible to render the patient euthyroid prior to surgery (if the surgery is urgent or the patient is allergic to antithyroid medications), the patient can be prepared with β-blockade and potassium iodide alone. Steroids can be a useful adjunct in this situation.
The extent of thyroidectomy to be performed used to be determined by the desired outcome (risk of recurrence vs. euthyroidism) and surgeon experience. In patients with coexistent thyroid cancer and those who refused RAI therapy or had severe ophthalmopathy or life-threatening reactions to antithyroid medications (vasculitis, agranulocytosis, or liver failure), total or near-total thyroidectomy was recommended. Ophthalmopathy has been demonstrated to stabilize or improve in most patients after total thyroidectomy, presumably from removal of the antigenic stimulus. A subtotal thyroidectomy, leaving a 4- to 7-g remnant, was recommended for all remaining patients. During subtotal thyroidectomy, remnant tissue may be left on each side (bilateral subtotal thyroidectomy), or a total lobectomy can be performed on one side with a subtotal thyroidectomy on the other side (Hartley-Dunhill procedure). Results were similar with either procedure,7 but the latter procedure was theoretically associated with fewer complications and requires re-entering only one side of the neck should recurrence require reoperation. Most studies, however, show no difference in the rates of complications with either approach, although patients undergoing a total resection had higher rates of temporary hypoparathyroidism. However, patients treated with subtotal thyroidectomy are prone to recurrence, the rates of which are dependent on remnant size. Based on the current evidence, recently published guidelines from the American Thyroid Association (ATA) and the American Association of Clinical Endocrinologists (AACE) recommend total or near-total thyroidectomy as the procedure of choice for the surgical management of Graves’ disease.8 Recurrent thyrotoxicosis usually is managed by radioiodine treatment.
Toxic multinodular goiters usually occur in older individuals, who often have a prior history of a nontoxic multinodular goiter. Over several years, enough thyroid nodules become autonomous to cause hyperthyroidism. The presentation often is insidious in that hyperthyroidism may only become apparent when patients are placed on low doses of thyroid hormone suppression for the goiter. Some patients have T3 toxicosis, whereas others may present only with atrial fibrillation or congestive heart failure. Hyperthyroidism also can be precipitated by iodide-containing drugs such as contrast media and the antiarrhythmic agent amiodarone (Jod-Basedow hyperthyroidism). Symptoms and signs of hyperthyroidism are similar to Graves’ disease, but extrathyroidal manifestations are absent.
Blood tests are similar to Graves’ disease with a suppressed TSH level and elevated free T4 or T3 levels. RAI uptake also is increased, showing multiple nodules with increased uptake and suppression of the remaining gland.
Hyperthyroidism must be adequately controlled. Both RAI and surgical resection may be used for treatment. When surgery is performed, near-total or total thyroidectomy is recommended to avoid recurrence and the consequent increased complication rates with repeat surgery. Care must be taken in identifying the RLN, which may be found laterally on the thyroid (rather than posterior) or stretched anteriorly over a nodule. RAI therapy is reserved for elderly patients who represent very poor operative risks, provided there is no airway compression from the goiter and thyroid cancer is not a concern. However, because uptake is less than in Graves’ disease, larger doses of RAI often are needed to treat the hyperthyroidism. Furthermore, RAI-induced thyroiditis has the potential to cause swelling and acute airway compromise and leaves the goiter intact, with the possibility of recurrent hyperthyroidism.
Hyperthyroidism from a single hyperfunctioning nodule typically occurs in younger patients who note recent growth of a long-standing nodule along with the symptoms of hyperthyroidism. Toxic adenomas are characterized by somatic mutations in the TSH-R gene, although G-protein–stimulating gene (gsp) mutations may occur also.9 Most hyperfunctioning or autonomous thyroid nodules have attained a size of at least 3 cm before hyperthyroidism occurs. Physical examination usually reveals a solitary thyroid nodule without palpable thyroid tissue on the contralateral side. RAI scanning shows a “hot” nodule with suppression of the rest of the thyroid gland. These nodules are rarely malignant. Smaller nodules may be managed with antithyroid medications and RAI. Larger nodules can require higher doses, which can lead to hypothyroidism. Surgery (lobectomy and isthmusectomy) is preferred to treat young patients and those with larger nodules. Percutaneous ethanol injection (PEI) has been reported to have reasonable success rates but has not been directly compared with surgery.
Thyroid storm is a condition of hyperthyroidism accompanied by fever, central nervous system agitation or depression, and cardiovascular and GI dysfunction, including hepatic failure. The condition may be precipitated by abrupt cessation of antithyroid medications, infection, thyroid or nonthyroid surgery, and trauma in patients with untreated thyrotoxicosis. Occasionally, thyroid storm may result from amiodarone administration or exposure to iodinated contrast agents or following RAI therapy. This condition was previously associated with high mortality rates but can be appropriately managed in an intensive care unit setting. β-Blockers are given to reduce peripheral T4 to T3 conversion and decrease the hyperthyroid symptoms. Oxygen supplementation and hemodynamic support should be instituted. Nonaspirin compounds can be used to treat pyrexia, and Lugol’s iodine or sodium ipodate (intravenously) should be administered to decrease iodine uptake and thyroid hormone secretion. PTU therapy blocks the formation of new thyroid hormone and reduces peripheral conversion of T4 to T3. Corticosteroids often are helpful to prevent adrenal exhaustion and block hepatic thyroid hormone conversion.
Deficiency in circulating levels of thyroid hormone leads to hypothyroidism and, in neonates, to cretinism, which is characterized by neurologic impairment and mental retardation. Hypothyroidism also may occur in Pendred’s syndrome (associated with deafness) and Turner’s syndrome. Conditions that cause hypothyroidism are listed in Table 38-2.
PRIMARY (INCREASED TSH LEVELS) | SECONDARY (DECREASED TSH LEVELS) | TERTIARY |
---|---|---|
Hashimoto’s thyroiditis | Pituitary tumor | Hypothalamic insufficiency |
RAI therapy for Graves’ disease | Pituitary resection or ablation | Resistance to thyroid hormone |
Postthyroidectomy | ||
Excessive iodine intake | ||
Subacute thyroiditis | ||
Medications: antithyroid drugs, lithium | ||
Rare: iodine deficiency, dyshormogenesis |
Failure of thyroid gland development or function in utero leads to cretinism and characteristic facies similar to those of children with Down syndrome and dwarfism. Failure to thrive and severe mental retardation often are present. Immediate testing and treatment with thyroid hormone at birth can lessen the neurologic and intellectual deficits. Hypothyroidism developing in childhood or adolescence results in delayed development and may also lead to abdominal distention, umbilical hernia, and rectal prolapse. In adults, symptoms in general are nonspecific, including tiredness, weight gain, cold intolerance, constipation, and menorrhagia. Patients with severe hypothyroidism or myxedema develop characteristic facial features due to the deposition of glycosaminoglycans in the subcutaneous tissues, leading to facial and periorbital puffiness. The skin becomes rough and dry and often develops a yellowish hue from reduced conversion of carotene to vitamin A. Hair becomes dry and brittle, and severe hair loss may occur. There is also a characteristic loss of the outer two thirds of the eyebrows. An enlarged tongue may impair speech, which is already slowed, in keeping with the impairment of mental processes. Patients may also have nonspecific abdominal pain accompanied by distention and constipation. Libido and fertility are impaired in both sexes. Cardiovascular changes in hypothyroidism include bradycardia, cardiomegaly, pericardial effusion, reduced cardiac output, and pulmonary effusions. When hypothyroidism occurs as a result of pituitary failure, other features of hypopituitarism, such as pale, waxy skin; loss of body hair; and atrophic genitalia, may be present.
Hypothyroidism is characterized by low circulating levels of T4 and T3. Raised TSH levels are found in primary thyroid failure, whereas secondary hypothyroidism is characterized by low TSH levels that do not increase following TRH stimulation. Thyroid autoantibodies are highest in patients with autoimmune disease (Hashimoto’s thyroiditis, Graves’ disease) and may also be elevated in patients with nodular goiter and thyroid neoplasms. An electrocardiogram demonstrates decreased voltage with flattening or inversion of T waves.
T4 is the treatment of choice and is administered in dosages varying from 50 to 200 μg per day, depending on the patient’s size and condition. Starting doses of 100 μg of T4 daily are well tolerated; however, elderly patients and those with coexisting heart disease and profound hypothyroidism should be started on a considerably lower dose such as 25 to 50 μg daily because of associated hypercholesterolemia and atherosclerosis. The dose can be slowly increased over weeks to months to attain a euthyroid state. A baseline electrocardiogram should always be obtained in patients with severe hypothyroidism before treatment. T4 dosage is titrated against clinical response and TSH levels, which should return to normal. The management of patients with subclinical hypothyroidism (normal T4, slightly raised TSH) is controversial. Some evidence suggests that patients with subclinical hypothyroidism and increased antithyroid antibody levels should be treated, because they will subsequently develop hypothyroidism. Patients who present with myxedema coma may require initial emergency treatment with large doses of IV T4 (300 to 400 μg), with careful monitoring in an intensive care unit setting.
Thyroiditis usually is classified into acute, subacute, and chronic forms, each associated with a distinct clinical presentation and histology.
The thyroid gland is inherently resistant to infection due to its extensive blood and lymphatic supply, high iodide content, and fibrous capsule. However, infectious agents can seed it (a) via the hematogenous or lymphatic route, (b) via direct spread from persistent pyriform sinus fistulae or thyroglossal duct cysts, (c) as a result of penetrating trauma to the thyroid gland, or (d) due to immunosuppression. Streptococcus and anaerobes account for about 70% of cases; however, other species also have been cultured.10 Acute suppurative thyroiditis is more common in children and often is preceded by an upper respiratory tract infection or otitis media. It is characterized by severe neck pain radiating to the jaws or ear, fever, chills, odynophagia, and dysphonia. Complications such as systemic sepsis, tracheal or esophageal rupture, jugular vein thrombosis, laryngeal chondritis, and perichondritis or sympathetic trunk paralysis may also occur.
The diagnosis is established by leukocytosis on blood tests and FNAB for Gram’s stain, culture, and cytology. CT scans may help to delineate the extent of infection and identify abscesses. A persistent pyriform sinus fistula should always be suspected in children with recurrent acute thyroiditis. The sensitivity of identification of fistulae in the acute setting is lowest for barium esophagography (50%) and best for direct endoscopy (100%), with CT scans being intermediate (80%). Both barium esophagogram and CT scans have improved sensitivity once the acute inflammation has resolved (100% and 83%, respectively), with CT being better at defining the accurate anatomic pathway and its relationship to the thyroid gland.11 Treatment consists of parenteral antibiotics and drainage of abscesses. Thyroidectomy may be needed for persistent abscesses or failure of open drainage. Patients with pyriform sinus fistulae require complete resection of the sinus tract, including the area of the thyroid where the tract terminates, to prevent recurrence. Transnasal flexible fiberoptic laryngoscopy is being increasingly used to identify the internal opening of the pyriform sinus tract and may also allow electrocauterization of the tract, and success rates similar to open surgery have been reported.
Subacute thyroiditis can occur in the painful or painless forms. Although the exact etiology is not known, painful thyroiditis is thought to be viral in origin or result from a postviral inflammatory response. Genetic predisposition may also play a role, as manifested by its strong association with the HLA-B35 haplotype. One model of pathogenesis suggests that viral or thyroid antigens, when presented by macrophages in the context of HLA-B35, stimulate cytotoxic T lymphocytes and damage thyroid follicular cells.
Painful thyroiditis most commonly occurs in 30- to 40-year-old women and is characterized by the sudden or gradual onset of neck pain, which may radiate toward the mandible or ear. History of a preceding upper respiratory tract infection often can be elicited. The gland is enlarged, exquisitely tender, and firm. The disorder classically progresses through four stages. An initial hyperthyroid phase, due to release of thyroid hormone, is followed by a second, euthyroid phase. The third phase, hypothyroidism, occurs in about 20% to 30% of patients and is followed by resolution and return to the euthyroid state in >90% of patients. A few patients develop recurrent disease.
In the early stages of the disease, TSH is decreased, and Tg, T4, and T3 levels are elevated due to the release of preformed thyroid hormone from destroyed follicles. The erythrocyte sedimentation rate is typically >100 mm/h. RAIU also is decreased (<2% at 24 hours), even in euthyroid patients, due to the release of thyroid hormones from destruction of the thyroid parenchyma. Painful thyroiditis is self-limited, and therefore, treatment is primarily symptomatic. Aspirin and other nonsteroidal anti-inflammatory drugs are used for pain relief, but steroids may be indicated in more severe cases. Short-term thyroid replacement may be needed and may shorten the duration of symptoms. Thyroidectomy is reserved for the rare patient who has a prolonged course not responsive to medical measures or for recurrent disease.
Painless thyroiditis is considered to be autoimmune in origin and may occur sporadically or in the postpartum period; the latter typically occurs at about 6 weeks after delivery in women with high TPO antibody titers in early pregnancy. This timing is thought to coincide with a decrease in the normal immune tolerance of pregnancy and consequent rebound elevation of antibody titers.
Painless thyroiditis also is more common in women and usually occurs between 30 and 60 years of age. Physical examination demonstrates a normal sized or minimally enlarged, slightly firm, nontender gland. Laboratory tests and RAIU are similar to those in painful thyroiditis, except for a normal erythrocyte sedimentation rate. The clinical course also parallels painful thyroiditis. Patients with symptoms may require β-blockers and thyroid hormone replacement. Thyroidectomy or RAI ablation is only indicated for the rare patient with recurrent, disabling episodes of thyroiditis.
Lymphocytic thyroiditis was first described by Hashimoto in 1912 as struma lymphomatosa—a transformation of thyroid tissue to lymphoid tissue. It is the most common inflammatory disorder of the thyroid and the leading cause of hypothyroidism.
Hashimoto’s thyroiditis is an autoimmune process that is thought to be initiated by the activation of CD4+ T (helper) lymphocytes with specificity for thyroid antigens. Once activated, T cells can recruit cytotoxic CD8+ T cells to the thyroid. Hypothyroidism results not only from the destruction of thyrocytes by cytotoxic T cells but also by autoantibodies, which lead to complement fixation and killing by natural killer cells or block the TSH-R. Antibodies are directed against three main antigens—Tg (60%), TPO (95%), and TSH-R (60%)—and, less commonly, the sodium/iodine symporter (25%). Apoptosis (programmed cell death) also has been implicated in the pathogenesis of Hashimoto’s thyroiditis. Chronic thyroiditis also has been associated with increased intake of iodine and administration of medications such as interferon-α, lithium, and amiodarone. Support for an inherited predisposition includes an increased incidence of thyroid autoantibodies in first-degree relatives of patients with Hashimoto’s thyroiditis compared to controls and the occurrence of the autoantibodies and hypothyroidism in patients with specific chromosomal abnormalities such as Turner’s syndrome and Down syndrome. Associations with HLA-B8, DR3, and DR5 haplotypes of the major histocompatibility complex also have been described.
On gross examination, the thyroid gland is usually mildly enlarged throughout and has a pale, gray-tan cut surface that is granular, nodular, and firm. On microscopic examination, the gland is diffusely infiltrated by small lymphocytes and plasma cells and occasionally shows well-developed germinal centers. Thyroid follicles are smaller than normal with reduced amounts of colloid and increased interstitial connective tissue. The follicles are lined by Hürthle or Askanazy cells, which are characterized by abundant eosinophilic, granular cytoplasm.
Hashimoto’s thyroiditis is also more common in women (male:female ratio 1:10 to 20) between the ages of 30 and 50 years old. The most common presentation is that of a minimally or moderately enlarged firm granular gland discovered on routine physical examination or the awareness of a painless anterior neck mass, although 20% of patients present with hypothyroidism, and 5% present with hyperthyroidism (Hashitoxicosis). In classic goitrous Hashimoto’s thyroiditis, physical examination reveals a diffusely enlarged, firm gland, which also is lobulated. An enlarged pyramidal lobe often is palpable.
When Hashimoto’s thyroiditis is suspected clinically, an elevated TSH and the presence of thyroid autoantibodies usually confirm the diagnosis. FNAB with ultrasound guidance is indicated in patients who present with a solitary suspicious nodule or a rapidly enlarging goiter. Thyroid lymphoma is a rare but well-recognized, ominous complication of chronic autoimmune thyroiditis and has a prevalence 80 times higher than expected frequency in this population than in a control population without thyroiditis. Recent studies of clonal similarity indicate that lymphoma may, in fact, evolve from Hashimoto’s thyroiditis.12
Thyroid hormone replacement therapy is indicated in overtly hypothyroid patients, with a goal of maintaining normal TSH levels. The management of patients with subclinical hypothyroidism (normal T4 and elevated TSH) is controversial. A systematic review of cohort studies showed that in age- and sex-adjusted analyses, subclinical hypothyroidism is associated with a hazard ratio (HR) for coronary heart disease events of 1.89 (95% confidence interval [CI], 1.28 to 2.80; P <.001) and coronary heart disease mortality of 1.58 (95% CI, 1.10 to 2.27; P = .005) for a TSH level of 10 to 19.9 mIU/L.13 The data for TSH levels of 5 to 10 mIU/L were less convincing. An evaluation of the 12 randomized controlled trials in this area only showed a trend toward improvement of some lipid parameters, and none of the included trials evaluated overall mortality or cardiac morbidity. Given the above, levothyroxine is recommended for all patients with TSH levels >10 μIU/mL and patients with levels of 5 to 10 μIU/mL in the presence of a goiter or anti-TPO antibodies. Treatment is also advised especially for middle-aged patients with cardiovascular risk factors such as hyperlipidemia or hypertension and in pregnant patients. Surgery may occasionally be indicated for suspicion of malignancy or for goiters causing compressive symptoms or cosmetic deformity.
Riedel’s thyroiditis is a rare variant of thyroiditis also known as Riedel’s struma or invasive fibrous thyroiditis that is characterized by the replacement of all or part of the thyroid parenchyma by fibrous tissue, which also invades into adjacent tissues. The etiology of this disorder is controversial, and it has been reported to occur in patients with other autoimmune diseases. This association, coupled with the presence of lymphoid infiltration and response to steroid therapy, suggests a primary autoimmune etiology. Riedel’s thyroiditis also is associated with other focal sclerosing syndromes including mediastinal, retroperitoneal, periorbital, and retro-orbital fibrosis and sclerosing cholangitis, suggesting that it may, in fact, be a primary fibrotic disorder. The disease occurs predominantly in women between the ages of 30 and 60 years old. It typically presents as a painless, hard anterior neck mass, which progresses over weeks to years to produce symptoms of compression, including dysphagia, dyspnea, choking, and hoarseness. Patients may present with symptoms of hypothyroidism and hypoparathyroidism as the gland is replaced by fibrous tissue. Physical examination reveals a hard, “woody” thyroid gland with fixation to surrounding tissues. The diagnosis needs to be confirmed by open thyroid biopsy, because the firm and fibrous nature of the gland renders FNAB inadequate.
Surgery is the mainstay of the treatment. The chief goal of operation is to decompress the trachea by wedge excision of the thyroid isthmus and to make a tissue diagnosis. More extensive resections are not advised due to the infiltrative nature of the fibrotic process that obscures usual landmarks and structures. Hypothyroid patients are treated with thyroid hormone replacement. Some patients who remain symptomatic have been reported to experience dramatic improvement after treatment with corticosteroids and tamoxifen. More recently mycophenolate mofetil has been used to attenuate the inflammatory process and led to dramatic symptom improvements in some patients.14
Any enlargement of the thyroid gland is referred to as a goiter. The causes of nontoxic goiters are listed in Table 38-3. Goiters may be diffuse, uninodular, or multinodular. Most nontoxic goiters are thought to result from TSH stimulation secondary to inadequate thyroid hormone synthesis and other paracrine growth factors.15 Elevated TSH levels induce diffuse thyroid hyperplasia, followed by focal hyperplasia, resulting in nodules that may or may not concentrate iodine, colloid nodules, or microfollicular nodules. The TSH-dependent nodules progress to become autonomous. Familial goiters resulting from inherited deficiencies in enzymes necessary for thyroid hormone synthesis may be complete or partial. The term endemic goiter refers to the occurrence of a goiter in a significant proportion of individuals in a particular geographic region. In the past, dietary iodine deficiency was the most common cause of endemic goiter. This condition has largely disappeared in North America due to routine use of iodized salt and iodination of fertilizers, animal feeds, and preservatives. However, in areas of iodine deficiency, such as Central Asia, South America, and Indonesia, up to 90% of the population have goiters. Other dietary goitrogens that may participate in endemic goiter formation include kelp, cassava, and cabbage. In many sporadic goiters, no obvious cause can be identified.
CLASSIFICATION | SPECIFIC ETIOLOGY |
---|---|
Endemic | Iodine deficiency, dietary goitrogens (cassava, cabbage) |
Medications | Iodide, amiodarone, lithium |
Thyroiditis | Subacute, chronic (Hashimoto’s) |
Familial | Impaired hormone synthesis from enzyme defects |
Neoplasm | Adenoma, carcinoma |
Resistance to thyroid hormone | — |
Most patients with nontoxic goiters are asymptomatic, although patients often complain of a pressure sensation in the neck. As the goiters become very large, compressive symptoms such as dyspnea and dysphagia ensue. Patients also describe having to clear their throats frequently (catarrh). Dysphonia from RLN injury is rare, except when malignancy is present. Obstruction of venous return at the thoracic inlet from a substernal goiter results in a positive Pemberton’s sign—facial flushing and dilatation of cervical veins upon raising the arms above the head (Fig. 38-13A). Sudden enlargement of nodules or cysts due to hemorrhage may cause acute pain. Physical examination may reveal a soft, diffusely enlarged gland (simple goiter) or nodules of various size and consistency in case of a multinodular goiter. Deviation or compression of the trachea may be apparent.
Figure 38-13.
A. Retrosternal extension of a large goiter may result in impeded flow in the superior vena cava, leading to dilated veins over the chest wall. This may become more prominent when patients raise their arms above the head—Pemberton’s sign. B. Computed tomography scan demonstrating retrosternal extension and consequent tracheal deviation and compression from a large goiter.
Patients usually are euthyroid with normal TSH and low-normal or normal free T4 levels. If some nodules develop autonomy, patients have suppressed TSH levels or become hyperthyroid. RAI uptake often shows patchy uptake with areas of hot and cold nodules. FNAB is recommended in patients who have a dominant nodule or one that is painful or enlarging, as carcinomas have been reported in 5% to 10% of multinodular goiters. CT scans are helpful to evaluate the extent of retrosternal extension and airway compression (Fig. 38-13B).
Most euthyroid patients with small, diffuse goiters do not require treatment. Some physicians give patients with large goiters exogenous thyroid hormone to reduce the TSH stimulation of gland growth; this treatment may result in decrease and/or stabilization of goiter size and is most effective for small diffuse goiters. Endemic goiters are treated by iodine administration. Surgical resection is reserved for goiters that (a) continue to increase despite T4 suppression, (b) cause obstructive symptoms, (c) have substernal extension, (d) have malignancy suspected or proven by FNAB, and (e) are cosmetically unacceptable. Near-total or total thyroidectomy is the treatment of choice, and patients require lifelong T4 therapy.
Solitary thyroid nodules are present in approximately 4% of individuals in the United States, whereas thyroid cancer has a much lower incidence of 40 new cases per 1 million. Therefore, it is of utmost importance to determine which patients with solitary thyroid nodule would benefit from surgery.
Details regarding the nodule, such as time of onset, change in size, and associated symptoms such as pain, dysphagia, dyspnea, or choking, should be elicited. Pain is an unusual symptom and, when present, should raise suspicion for intrathyroidal hemorrhage in a benign nodule, thyroiditis, or malignancy. Patients with MTC may complain of a dull, aching sensation. A history of hoarseness is worrisome, as it may be secondary to malignant involvement of the RLNs. Most importantly, patients should be questioned regarding risk factors for malignancy, such as exposure to ionizing radiation and family history of thyroid and other malignancies associated with thyroid cancer.
Low-dose therapeutic radiation has been used to treat conditions such as tinea capitis (6.5 cGy), thymic enlargement (100 to 400 cGy), enlarged tonsils and adenoids (750 cGy), acne vulgaris (200 to 1500 cGy), and other conditions such as hemangioma and scrofula. Radiation (approximately 4000 cGy) is also an integral part of the management of patients with Hodgkin’s disease. It is now known that a history of exposure to low-dose ionizing radiation to the thyroid gland places the patient at increased risk for developing thyroid cancer. The risk increases linearly from 6.5 to 2000 cGy, beyond which the incidence declines as the radiation causes destruction of the thyroid tissue. The risk is maximum 20 to 30 years after exposure, but these patients require lifelong monitoring. During the nuclear fallout from Chernobyl in 1986, 131I release was accompanied by a marked increase in the incidence of both benign and malignant thyroid lesions noted within 4 years of exposure, particularly in children.16 Most thyroid carcinomas following radiation exposure are papillary, and some of these cancers with a solid type of histology and presence of RET/PTC translocations appear to be more aggressive. In general, there is a 40% chance that patients presenting with a thyroid nodule and a history of radiation have thyroid cancer. Of those patients who have thyroid cancer, the cancer is located in the dominant nodule in 60% of patients, but in the remaining 40% of patients, the cancer is in another nodule in the thyroid gland.
A family history of thyroid cancer is a risk factor for the development of both medullary and nonmedullary thyroid cancer. Familial MTCs occur in isolation or in association with other tumors as part of multiple endocrine neoplasia type 2 (MEN2) syndromes. Nonmedullary thyroid cancers can occur in association with other known familial cancer syndromes such as Cowden’s syndrome, Werner’s syndrome (adult progeroid syndrome), and familial adenomatous polyposis (Table 38-4). Nonmedullary thyroid cancers can also occur independently of these syndromes as the predominant tumors in the families. The definition of familial nonmedullary thyroid cancer (FNMTC) is variable across the literature; however, in most studies, it is defined by the presence of two or more first-degree relatives with follicular cell–derived cancers. FNMTC is now recognized as a distinct clinical entity associated with a high incidence of multifocal tumors and benign thyroid nodules. Some studies report that these patients have higher locoregional recurrence rates and consequent shorter disease-free survival. Several candidate loci that predispose to these tumors have been identified, including MNG1 (14q32), thyroid carcinoma with oxphilia (TCO, on 19p13.2), fPTC/papillary renal neoplasia (PRN, on 1q21), NMTC (2q21), FTEN (8p23.1-p22), and the telomere-telomerase complex.17
SYNDROME | GENE | MANIFESTATION | THYROID TUMOR |
---|---|---|---|
Cowden’s syndrome | PTEN | Intestinal hamartomas, benign and malignant breast tumors | FTC, rarely PTC and Hürthle cell tumors |
FAP | APC | Colon polyps and cancer, duodenal neoplasms, desmoids | PTC cribriform growth pattern |
Werner’s syndrome | WRN | Adult progeroid syndrome | PTC, FTC, anaplastic cancer |
Carney complex type 1 | PRKAR1α | Cutaneous and cardiac myxomas, breast and adrenal tumors | PTC, FTC |
McCune-Albright syndrome | GNAS1 | Polyostotic fibrous dysplasia, endocrine abnormalities, café-au-lait spots | PTC clear cell |
The thyroid gland is best palpated from behind the patient and with the neck in mild extension. The cricoid cartilage is an important landmark, as the isthmus is situated just below it. Nodules that are hard, gritty, or fixed to surrounding structures such as the trachea or strap muscles are more likely to be malignant. The cervical chain of lymph nodes should be assessed as well as the nodes in the posterior triangle.
An algorithm for the workup of a solitary thyroid nodule is shown in Fig. 38-14.
Figure 38-14.
Management of a solitary thyroid nodule based on Bethesda criteria. a = except in patients with a history of external radiation exposure or a family history of thyroid cancer; FNAB = fine-needle aspiration biopsy; AUS = atypia of unknown significance; FLUS = follicular lesion of unknown significance; FN = follicular neoplasm.
FNAB has become the single most important test in the evaluation of thyroid masses and can be performed with or without ultrasound guidance.18 Ultrasound guidance is recommended for nodules that are difficult to palpate, for cystic or solid-cystic nodules that recur after the initial aspiration, and for multinodular goiters. A 23-gauge needle is inserted into the thyroid mass, and several passes are made while aspirating the syringe. After releasing the suction on the syringe, the needle is withdrawn and the cells are immediately placed on prelabeled dry glass slides; some are immersed in a 70% alcohol solution while others are air dried. A sample of the aspirate is also placed in a 90% alcohol solution for cytospin or cell pellet. The slides are stained by Papanicolaou’s or Wright’s stains and examined under the microscope. If a bloody aspirate is obtained, the patient should be repositioned in a more upright position and the biopsy repeated with a finer (25- to 30-gauge) needle.
After FNAB, the majority of nodules can be classified into several categories that determine further management. To address the issue of variability in the terminology of fine-needle aspiration (FNA), the National Cancer Institute (NCI) hosted the “NCI Thyroid Fine Needle Aspiration State of the Science Conference,” which then defined the Bethesda criteria for thyroid FNA.19 Accordingly, optimum cytology specimens should have at least six follicles each containing at least 10 to 15 cells from at least two aspirates.
The FNA is classified as “nondiagnostic or unsatisfactory” in 2% to 20% of cases and typically results from a virtually acellular specimen, cyst fluid, or the presence of blood or clotting artifact. The risk of malignancy in this setting ranges from 1% to 4%, and reaspiration under ultrasound guidance is recommended. A “benign” result is obtained in 60% to 70% of thyroid FNAs. The most common lesion in this setting is a follicular nodule (includes adenomatoid nodule, colloid nodule, and follicular adenoma). Other diagnoses include lymphocytic (Hashimoto’s) thyroiditis and granulomatous thyroiditis. False-negative results are reported in up to 3% of cases, and follow-up is recommended. A result of “atypia of unknown significance (AUS) or follicular lesion of unknown significance (FLUS)” is obtained in 3% to 6% of biopsies. The risk of malignancy in this scenario is difficult to determine; however, it is thought to be in the range of 10% to 35% for FLUS and 60% to 75% for AUS. Clinical correlation and a repeat FNA are recommended for AUS lesions (which often results in a more definitive interpretation), although clinical observation or surgery may be appropriate because of worrisome clinical or ultrasound findings. The category of “follicular neoplasm” is intended to identify nodules that might be follicular carcinomas. The term suspicious for a follicular neoplasm is preferred by some laboratories for this category because up to 35% of cases turn out not to be neoplasms but hyperplastic proliferations of follicular cells, most commonly those of multinodular goiter. Lobectomy is the preferred treatment for this result, and approximately 15% to 35% of lesions placed in this category prove to be malignant. Hürthle cell neoplasms are also included in this category. Most papillary and other carcinomas can be diagnosed by FNA, but the features are subtle at times, such as in follicular variant of papillary carcinomas. If the diagnosis is uncertain, the lesions are classified as “suspicious for malignancy.” Lobectomy or near-total thyroidectomy is recommended because more than 60% turn out to be malignant. This category also includes lesions suspicious for medullary carcinoma and lymphoma, and ancillary testing such as immunohistochemical analysis and flow cytometry may be helpful. The risk of malignancy in lesions classified as “malignant” by FNA is 97% to 99%, and near-total/total thyroidectomy is recommended.
Most patients with thyroid nodules are euthyroid. Determining the blood TSH level is helpful. If a patient with a nodule is found to be hyperthyroid, the risk of malignancy is approximately 1%. Serum Tg levels cannot differentiate benign from malignant thyroid nodules unless the levels are extremely high, in which case metastatic thyroid cancer should be suspected. Tg levels are, however, useful in following patients who have undergone total thyroidectomy for thyroid cancer and also for serial evaluation of patients undergoing nonoperative management of thyroid nodules. Serum calcitonin levels should be obtained in patients with MTC or a family history of MTC or MEN2. There is insufficient evidence to recommend routine calcitonin testing for all nodules. All patients with MTC should be tested for RET oncogene mutations and have a 24-hour urine collection with measurement of levels of vanillylmandelic acid (VMA), metanephrine, and catecholamine levels to rule out a coexisting pheochromocytoma. About 10% of patients with familial MTC and MEN2A have de novo RET mutations, so that their children are at risk for thyroid cancer.
Ultrasound is helpful for detecting nonpalpable thyroid nodules, differentiating solid from cystic nodules, and identifying adjacent lymphadenopathy. Ultrasound evaluation can identify features of a nodule that increase the a priori risk of malignancy, such as fine stippled calcification and enlarged regional nodes; however, a tissue diagnosis is strongly recommended before thyroidectomy.20 Ultrasound also provides a noninvasive and inexpensive method of following the size of suspected benign nodules diagnosed by FNAB and for identifying enlarged lymph nodes. Ultrasound elastography is used to evaluate tissue stiffness noninvasively. This technique takes advantage of the fact that malignant nodules tend to be harder than benign nodules and thus deform less compared with the surrounding normal thyroid parenchyma.21 Larger studies are warranted before elastography can be routinely included in the evaluation of thyroid nodules. CT and MRI are unnecessary in the routine evaluation of thyroid tumors except for large, fixed, or substernal lesions. Scanning the thyroid with 123I or 99mTc is rarely necessary, and thyroid scanning currently is recommended in the assessment of thyroid nodules only in patients who have follicular thyroid nodules on FNAB and a suppressed TSH. PET scanning does not play a major role in the primary evaluation of thyroid nodules.
Malignant tumors are treated by thyroidectomy, as discussed earlier and later in this chapter in Surgical Treatment under Malignant Thyroid Disease. Simple thyroid cysts resolve with aspiration in about 75% of cases, although some require a second or third aspiration. If the cyst persists after three attempts at aspiration, unilateral thyroid lobectomy is recommended. Lobectomy also is recommended for cysts >4 cm in diameter or complex cysts with solid and cystic components, as the latter have a higher incidence of malignancy (15%). When FNAB is used in complex nodules, the solid portion should be sampled. If a colloid nodule is diagnosed by FNAB, patients should still be observed with serial ultrasound and Tg measurements. If the nodule enlarges, repeat FNAB often is indicated. Although controversial, levothyroxine in doses sufficient to maintain a serum TSH level between 0.1 and 1.0 μU/mL may also be administered. In areas with a high prevalence of iodine deficiency, this can decrease nodule size and potentially prevent the growth of new nodules. In iodine-sufficient populations, the data are less impressive. Randomized controlled trial analyses have shown that less than 25% of benign nodules shrink more than 50% with TSH suppression in iodine-replete populations. Thyroidectomy should be performed if a nodule enlarges on TSH suppression or causes compressive symptoms, or for cosmetic reasons. An exception to this general rule is the patient who has had previous irradiation of the thyroid gland or has a family history of thyroid cancer. In these patients, total or near-total thyroidectomy is recommended because of the high incidence of thyroid cancer and decreased reliability of FNAB in this setting.
In the United States, thyroid cancer accounts for <1% of all malignancies (2% of women and 0.5% of men) and is the most rapidly increasing cancer in women. Thyroid cancer is responsible for six deaths per million persons annually. Most patients present with a palpable swelling in the neck, which initiates assessment through a combination of history, physical examination, and FNAB.
Several oncogenes and tumor suppressor genes are involved in thyroid tumorigenesis,22 as depicted in Table 38-5. The RET proto-oncogene (Fig. 38-15) plays a significant role in the pathogenesis of thyroid cancers. It is located on chromosome 10 and encodes a receptor tyrosine kinase, which binds several growth factors such as glial-derived neurotrophic factor and neurturin. The RET protein is expressed in tissues derived from the embryonic nervous and excretory systems. Therefore, RET disruption can lead to developmental abnormalities in organs derived from these systems, such as the enteric nervous system (Hirschsprung’s disease) and kidney. Germline mutations in the RET proto-oncogene are known to predispose to MEN2A, MEN2B, and familial MTCs, and somatic mutations have been demonstrated in tumors derived from the neural crest, such as MTCs (30%) and pheochromocytomas. The tyrosine kinase domain of RET can fuse with other genes by rearrangement. These fusion products also function as oncogenes and have been implicated in the pathogenesis of PTCs. At least 15 RET/PTC rearrangements have been described and appear to be early events in tumorigenesis. Young age and radiation exposure seem to be independent risk factors for the development of RET/PTC rearrangements. Up to 70% of papillary cancers in children exposed to the radiation fallout from the 1986 Chernobyl disaster carry RET/PTC rearrangements, the most common being RET/PTC1 and RET/PTC3. These rearrangements confer constitutive activation of the receptor tyrosine kinases. RET/PTC3 is associated with a solid type of PTC that appears to present at a higher stage and to be more aggressive. It has now been established that RET/PTC signaling involves the mitogen-activated protein kinase (MAPK) pathway via other signaling molecules such as Ras, Raf, and MEK. In normal cells, physiologic activation of Raf kinases occurs via direct interaction with guanosine triphosphate (GTP)–bound Ras, a membrane-bound small G protein. Activated Raf, a serine-threonine kinase, in turn phosphorylates MEK, another serine-threonine kinase. This leads to phosphorylation of ERK/MAPK, which phosphorylates regulatory molecules in the nucleus, thereby altering gene expression. Aberrant activation of the MAPK pathway leads to tumorigenesis. Aside from RET/PTC alterations, mutations in the Ras genes can also activate the MAPK pathway. Mutated RAS oncogenes have been identified in up to 20% to 40% of thyroid follicular adenomas and carcinomas, multinodular goiters, and papillary and anaplastic carcinomas. There are three Raf kinases, A-Raf, B-Raf (BRAF), and C-Raf. Mutations in BRAF also have been implicated in aberrant MAPK pathway activation and tumorigenesis. Of the various identified BRAF mutations, T1799A (V600E amino acid substitution) is the most common and occurs frequently in thyroid cancers. Interestingly, BRAF mutations occur in papillary and anaplastic tumors (average prevalence of 44% and 22%, respectively),15 but not in follicular thyroid cancers, suggesting a role in the pathogenesis of these malignancies. Studies also show that BRAF mutations are associated with more aggressive clinicopathologic features, including larger tumor size, invasion, and lymphadenopathy, and may have a role as prognostic markers.
GENE | FUNCTION | TUMOR |
---|---|---|
Oncogenes | ||
RET | Membrane receptor with tyrosine kinase activity | Sporadic and familial MTC, PTC (RET/PTC rearrangements) |
MET | Same | Overexpressed in PTC |
TRK1 | Same | Activated in some PTC |
TSH-R | Linked to heterotrimeric G protein | Hyperfunctioning adenoma |
Gsα (gsp) | Signal transduction molecule (GTP binding) | Hyperfunctioning adenoma, follicular adenoma |
Ras | Signal transduction protein | Follicular adenoma and carcinoma, PTC |
PAX8/PPARγ1 | Oncoprotein | Follicular adenoma, follicular carcinoma |
B-Raf (BRAF) | Signal transduction | PTC, tall cell and poorly differentiated, anaplastic |
CTNNB1 (β-catenin) | Signal transduction | Upregulated in poorly differentiated and anaplastic cancers |
Tumor suppressors | ||
p53 | Cell cycle regulator, arrests cells in G1, induces apoptosis | Dedifferentiated PTC, FTC, anaplastic cancers |
p16 | Cell cycle regulator, inhibits cyclin-dependent kinase | Thyroid cancer cell lines |
PTEN | Protein tyrosine phosphatase | Follicular adenoma and carcinoma |
Other genetic alterations | ||
microRNA | Small, noncoding RNA | Specific types upregulated in papillary and some follicular carcinomas |
Figure 38-15.
Structure of the RET tyrosine kinase receptor. Multiple endocrine neoplasia type 2A (MEN2A) and type 2B (MEN2B), familial medullary thyroid cancer (FMTC), and Hirschsprung’s disease result from germline mutations in the RET proto-oncogene. The extracellular domain binds the ligand glial-derived neurotrophic factor (GDNF) and contains 28 cysteine residues. Mutations in cysteine residues at codons 609, 611, 618, 620, and 634, which are in the juxtamembrane region of the receptor, are associated with MEN2A and FMTC. The ATP-binding site is located intracellularly near the site, which binds the substrate for the tyrosine kinase catalytic domain. Mutations at codon 918 (Met to Thr) alter the substrate binding pocket located in the intracellular region and cause MEN2B. FMTC is associated with mutations at codons 768 and 804. ATP = adenosine triphosphate. (Reproduced with permission from Wells S, Franz C. Medullary carcinoma of the thyroid. World J Surg. 2000;24:954. With kind permission of Springer Science + Business Media.)
The p53 gene is a tumor suppressor gene encoding a transcriptional regulator, which causes cell cycle arrest allowing repair of damaged DNA, thus helping to maintain genomic integrity. Mutations of p53 are rare in PTCs but common in undifferentiated thyroid cancers and thyroid cancer cell lines. Other cell cycle regulators and tumor suppressors such as p15 and p16 are mutated more commonly in thyroid cancer cell lines than in primary tumors. An oncogene resulting from the fusion of the DNA binding domain of the thyroid-transcription factor PAX8 gene to the peroxisome proliferator-activated receptor gamma 1 (PPARγ1) has been noted to play an important role in the development of follicular neoplasms, including follicular cancers. Thyroid cancer stem cells have also been identified; however, their role in thyroid carcinogenesis remains to be determined.24
Papillary carcinoma accounts for 80% of all thyroid malignancies in iodine-sufficient areas and is the predominant thyroid cancer in children and individuals exposed to external radiation. Papillary carcinoma occurs more often in women, with a 2:1 female-to-male ratio, and the mean age at presentation is 30 to 40 years. Most patients are euthyroid and present with a slow-growing painless mass in the neck. Dysphagia, dyspnea, and dysphonia usually are associated with locally advanced invasive disease. Lymph node metastases are common, especially in children and young adults, and may be the presenting complaint. “Lateral aberrant thyroid” almost always denotes a cervical lymph node that has been invaded by metastatic cancer. Suspicion of thyroid cancer often originates through physical examination of patients and a review of their history. Diagnosis is established by FNAB of the thyroid mass or lymph node. Once thyroid cancer is diagnosed on FNAB, a complete neck ultrasound is strongly recommended to evaluate the contralateral lobe and for lymph node metastases in the central and lateral neck compartments. Distant metastases are uncommon at initial presentation, but may ultimately develop in up to 20% of patients. The most common sites are lungs, followed by bone, liver, and brain.
On gross examination, PTCs generally are hard and whitish and remain flat on sectioning with a blade, in contrast to normal tissue or benign nodular lesions that tend to bulge. Macroscopic calcification, necrosis, or cystic change may be apparent. Histologically, papillary carcinomas may exhibit papillary projections (Fig. 38-16A), a mixed pattern of papillary and follicular structures, or a pure follicular pattern (follicular variant). The diagnosis is established by characteristic nuclear cellular features. Cells are cuboidal with pale, abundant cytoplasm, crowded nuclei that may demonstrate “grooving,” and intranuclear cytoplasmic inclusions (leading to the designation of Orphan Annie nuclei [Fig. 38-16B]), which allow diagnosis by FNAB. Psammoma bodies, which are microscopic, calcified deposits representing clumps of sloughed cells, also may be present. Mixed papillary-follicular tumors and follicular variants of papillary carcinoma are classified as papillary carcinomas because they behave biologically as papillary carcinomas. Multifocality is common in papillary carcinoma and may be present in up to 85% of cases on microscopic examination. Multifocality is associated with an increased risk of cervical nodal metastases, and these tumors may rarely invade adjacent structures such as the trachea, esophagus, and RLNs. Other variants of papillary carcinoma include tall cell, insular, columnar, diffuse sclerosing, clear cell, trabecular, and poorly differentiated types. These variants account for about 1% of all papillary carcinomas and are generally associated with a worse prognosis.
Minimal or occult/microcarcinoma refers to tumors of 1 cm or less in size with no evidence of local invasiveness through the thyroid capsule or angioinvasion, and that are not associated with lymph node metastases. They are nonpalpable and usually are incidental findings at operative, histologic, or autopsy examination. Studies have demonstrated occult PTC to be present in 2% to 36% of thyroid glands removed at autopsy. These tumors are also being identified more frequently due to the widespread use of ultrasound. These occult tumors are generally associated with a better prognosis than larger tumors, but they may be more aggressive than previously appreciated. About 25% of patients with these tumors have associated occult lymph node metastases.
In general, patients with PTC have an excellent prognosis with a >95% 10-year survival rate. Several prognostic indicators have been incorporated into various staging systems, which enable patients to be stratified into low-risk and high-risk groups. Unfortunately, all of these classification systems rely on data that are not available preoperatively.
In 1987, Hay and colleagues25 at the Mayo Clinic proposed the AGES scoring system, which incorporates Age, histologic Grade, Extrathyroidal invasion, and metastases and tumor Size to predict the risk of dying from papillary cancer. Low-risk patients are young, with well-differentiated tumors, no metastases, and small primary lesions, whereas high-risk patients are older, with poorly differentiated tumors, local invasion, distant metastases, and large primary lesions. The MACIS scale is a postoperative system modified from the AGES scale. This scale incorporates distant Metastases, Age at presentation (<40 or >40 years old), Completeness of original surgical resection, extrathyroidal Invasion, and Size of original lesion (in centimeters) and classifies patients into four risk groups based on their scores. Cady proposed the AMES system26 to classify differentiated thyroid tumors into low- and high-risk groups using Age (men <40 years old, women <50 years old), Metastases, Extrathyroidal spread, and Size of tumors (< or >5 cm). Another classification system is the TNM system (Tumor, Nodal status, Metastases; Table 38-6), which used by most medical centers in North America.27 A simplified system by DeGroot and associates28 uses four groups—class I (intrathyroidal), class II (cervical nodal metastases), class III (extrathyroidal invasion), and class IV (distant metastases)—to determine prognosis.
PAPILLARY OR FOLLICULAR TUMORS | |
---|---|
STAGE | TNM |
<45 y | |
I | Any T, any N, M0 |
II | Any T, any N, M1 |
≥45 y | |
I | T1, N0, M0 |
II | T2, N0, M0 |
III | T3, N0, M0; T1–3, N1a, M0 |
IVA | T4a, N0–1a, M0; T1–4a, N1b, M0 |
IVB | T4b, any N, M0 |
IVC | Any T, any N, M1 |
Medullary Thyroid Cancer | |
Stage | TNM |
I | T1, N0, M0 |
II | T2–3, N0, M0 |
III | T1–3, N1a, M0 |
IVA | T4a, N0–1a, M0; T1–4a, N1b, M0 |
IVB | T4b, any N, M0 |
IVC | Any T, any N, M1 |
Anaplastic Cancer | |
Stage | TNM |
IVA | T4a, Any N, M0 |
IVB | T4b, Any N, M0 |
IVC | Any T, Any M, M1 |
Several molecular and genetic markers such as tumor DNA aneuploidy, decreased cyclic adenosine monophosphate response to TSH, increased epidermal growth factor binding, presence of N-ras and gsp mutations, overexpression of c-myc, and presence of p53 mutations also have been associated with a worse prognosis. The presence of BRAF V600E mutation, as previously mentioned, is associated with aggressive tumor characteristics, including extrathyroidal extension, older age at presentation, and lymph node and distant metastases. This mutation also appears to be an independent predictor of both tumor recurrence (even for early-stage disease) and tumor-related mortality. Some studies propose that BRAF mutation status on FNAB can be used to tailor initial management including more extensive initial surgical excision, high-dose postoperative RAI therapy, increased TSH suppression, and closer follow up.29 The correlation of RET/PTC rearrangements and Ras mutations with prognosis is less clear.
Most authors agree that patients with high-risk tumors (judged by any of the classification systems discussed earlier in Prognostic Indicators) or bilateral tumors should undergo total or near-total thyroidectomy. The optimal surgical strategy in the majority of patients with low-risk (small, unilateral) cancers was controversial for many years, with the focus of the debate centering around outcome data and risks associated with extent of thyroidectomy in this group of patients.
Proponents of total thyroidectomy indicate that it enables the use of RAI to effectively detect and treat residual thyroid tissue or metastatic disease and makes serum Tg level a more sensitive marker of recurrent or persistent disease. It is also known that a significant proportion (33% to 50%) of patients who develop a recurrence die from their disease,30 and even though the data are retrospective, long-term, follow-up studies suggest that recurrence rates are lowered and that survival is improved in patients undergoing near-total or total thyroidectomy28,30,31,32,33,34 (Fig. 38-17). In addition, diminished survival is noted in patients with low-risk disease (mortality rates of 5% at 10 to 20 years), and it is not possible to accurately risk stratify patients preoperatively. More recently, a large study of >50,000 patients with papillary cancer demonstrated that, in multivariate analyses, total thyroidectomy led to a significantly improved recurrence and survival for tumors >1 cm. Furthermore, the authors also showed that patients with tumors 1 to 2 cm in diameter who were treated with lobectomy had a 24% higher risk of recurrence and a 49% higher risk of thyroid cancer mortality.35 Based on the above, current guidelines for the evidence-based management of thyroid cancers recommend a near-total or total thyroidectomy for primary cancers >1 cm unless there are contraindications to the surgery.36
Figure 38-17.
Improved survival in patients with papillary or follicular thyroid cancer following total or near-total thyroidectomy compared to those who underwent less than near-total thyroidectomy. (Reproduced with permission from Mazzaferri E, Jhiang S. Long-term impact of initial surgical and medical therapy on papillary and follicular thyroid cancer. Am J Med. 1994;97:424. Copyright Elsevier.)
When PTC is diagnosed by FNAB, the definitive operation can be done without confirming the diagnosis by frozen section during the operation. Patients with a nodule that is suspicious for papillary cancer should be treated by thyroid lobectomy, isthmusectomy, and removal of any pyramidal lobe or adjacent lymph nodes. If intraoperative frozen-section examination of a lymph node or primary tumor confirms carcinoma, completion total or near-total thyroidectomy is performed. If a definitive diagnosis cannot be made or the surgeon is concerned about the viability of the parathyroid glands or the status of the RLN, the operation is terminated. When final histology confirms carcinoma, completion thyroidectomy usually is performed. Thyroid lobectomy alone is considered sufficient treatment for small (<1 cm), incidentally discovered, low-risk, unifocal, intrathyroidal papillary carcinomas in the absence of prior head and neck irradiation or radiologically or clinically involved cervical nodal metastases.
During thyroidectomy, enlarged or obviously involved central neck nodes should be removed (therapeutic central-compartment, level VI), along with nodes with known lateral neck metastases. Some investigators recommend routine bilateral central neck dissection due to the high incidence of microscopic metastases and data showing improved rates of recurrence and survival (compared to historic controls). However, these risks need to be balanced with the increased risk of hypoparathyroidism with routine central neck dissection and the fact that some studies do not show any difference in recurrence rates or rates of low or undetectable Tg levels. The 2009 ATA guidelines for thyroid cancer management suggest that prophylactic (ipsilateral or bilateral) dissection may be performed in patients with advanced (T3 or T4) papillary thyroid carcinoma, whereas the procedure is not needed for small (T1 and T2) tumors that are clinically node negative.36 Further prospective studies are needed before definitive recommendations can be made in this regard.
Biopsy-proven lymph node metastases detected clinically or by imaging in the lateral neck in patients with papillary carcinoma are managed with modified radical or functional neck dissection,36 as described later in this chapter in Thyroid Surgery. Dissection of the posterior triangle and suprahyoid dissection usually are not necessary unless there is extensive metastatic disease in levels 2, 3, and 4, but should be performed when appropriate. Prophylactic lateral neck node dissection is not necessary in patients with PTC, because these cancers do not appear to metastasize systemically from lymph nodes, and micrometastases often can be ablated with RAI therapy.
Follicular carcinomas account for 10% of thyroid cancers and occur more commonly in iodine-deficient areas. The overall incidence of this tumor is declining in the United States, probably due to iodine supplementation and improved histologic classification. Women have a higher incidence of follicular cancer, with a female-to-male ratio of 3:1, and a mean age at presentation of 50 years old. Follicular cancers usually present as solitary thyroid nodules, occasionally with a history of rapid size increase, and long-standing goiter. Pain is uncommon, unless hemorrhage into the nodule has occurred. Unlike papillary cancers, cervical lymphadenopathy is uncommon at initial presentation (about 5%), although distant metastases may be present. In <1% of cases, follicular cancers may be hyperfunctioning, leading patients to present with signs and symptoms of thyrotoxicosis. FNAB is unable to distinguish benign follicular lesions from follicular carcinomas. Therefore, preoperative clinical diagnosis of cancer is difficult unless distant metastases are present. Large follicular tumors (>4 cm) in older men are more likely to be malignant.
Due to the limitations inherent in the FNAB diagnosis, a number of studies have focused on identifying molecular markers to distinguish benign from malignant follicular lesions. Many of these genetic changes can be identified using tissue obtained during FNAB. While no single marker has met the ideal characteristics of being simple to use, reproducible, and cost-effective, several studies have explored combinations of markers that appear to be useful in differentiating benign from malignant lesions. Nikiforov and colleagues demonstrated the utility of examining a combination of DNA-based markers including BRAF, RAS, RET/PTC, and PAX/PPARγ abnormalities in the diagnosis of indeterminate nodules. In their study, the presence of any mutation in an FNAB sample was a strong indicator of cancer.37,38 Expression arrays also have been used to investigate the role of microRNAs, which are a new class of small, noncoding RNAs that have been implicated in carcinogenesis. The specific microRNAs miR-197 and miR-346 are upregulated in follicular thyroid cancers39 and have the potential to be used as diagnostic markers. Additional studies have also demonstrated the feasibility of studying a panel of microRNAs in a small number of FNA samples. Peripheral blood measurement of TSH-R mRNA levels was able to predict malignancy with a sensitivity of 90% and specificity of 80%; however, its widespread application requires further study.40 A recently published prospective, multicenter study validated a novel diagnostic test that measures the expression of 167 genes (Veracyte). The gene expression classifier correctly classified 78 of 85 indeterminate nodules as suspicious with a sensitivity of 92%. Additional studies are needed to assess its utility in the broader clinical setting.41
Follicular carcinomas usually are solitary lesions, and the majority are surrounded by a capsule. Histologically, follicles are present, but the lumen may be devoid of colloid. Architectural patterns depend on the degree of differentiation demonstrated by the tumor. Malignancy is defined by the presence of capsular and vascular invasion (Fig. 38-18
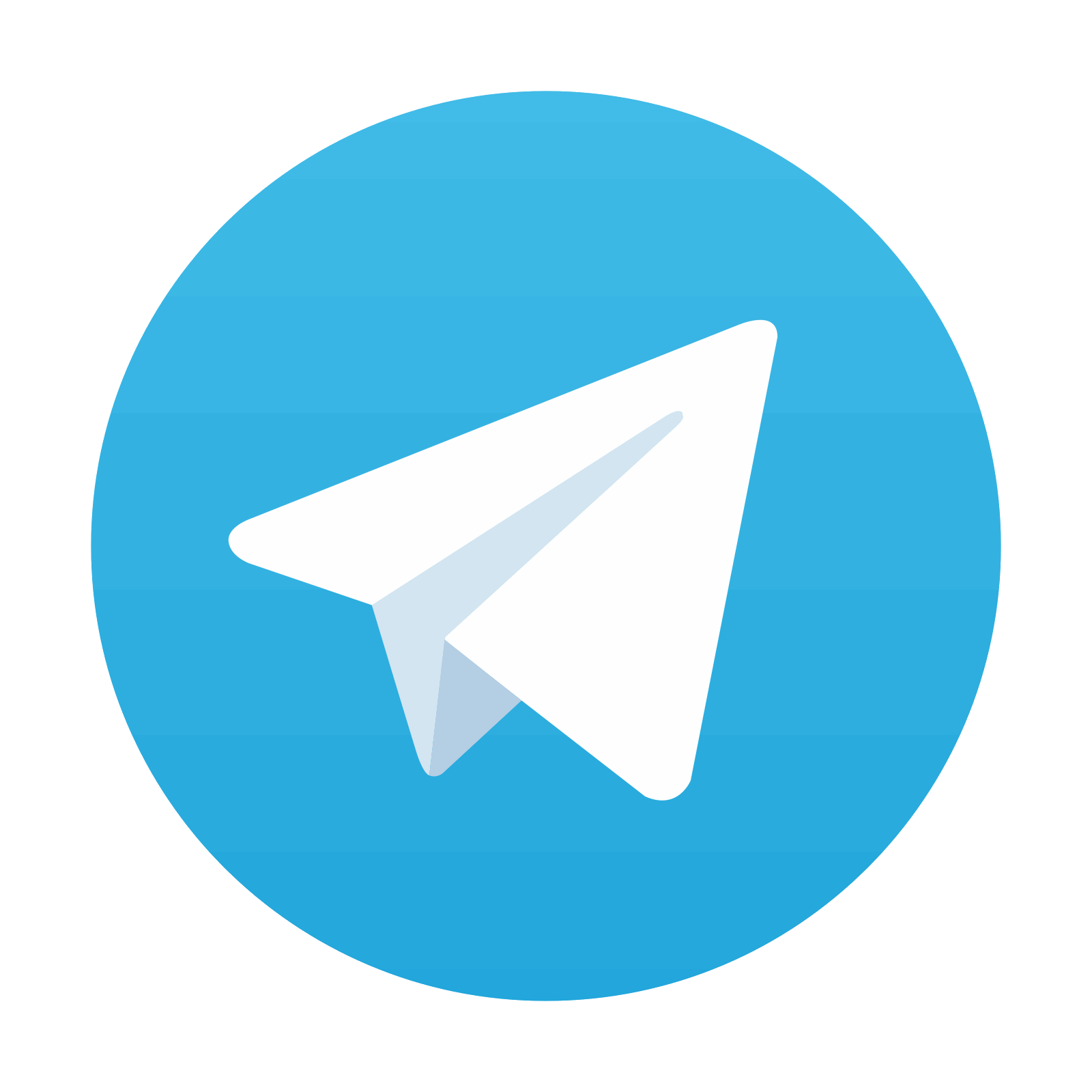
Stay updated, free articles. Join our Telegram channel

Full access? Get Clinical Tree
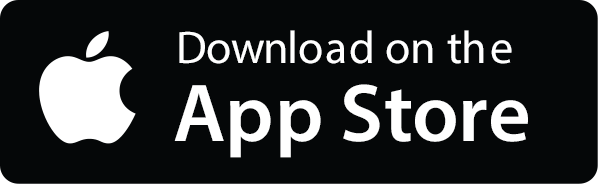
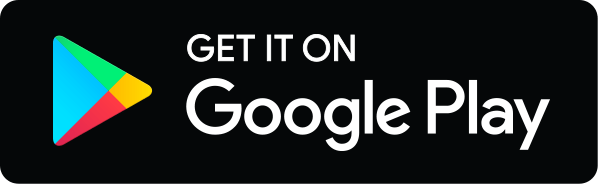
