Objectives
- Identify the steps and control factors of thyroid hormone biosynthesis, storage, and release.
- Describe the distribution of iodine and the metabolic pathway involved in thyroid hormone synthesis.
- Explain the importance of thyroid hormone binding in blood for free and total thyroid hormone levels.
- Understand the significance of the conversion of tetraiodothyronine (T4) to triiodothyronine (T3) and reverse T3 (rT3) in extrathyroidal tissues.
- Understand how thyroid hormones produce their cellular effects.
- Describe their effects on development and metabolism.
- Understand the causes and consequences of excess and deficiency of thyroid hormones.
Thyroid Gland: Introduction
Thyroid hormones play important roles in maintaining energy homeostasis and regulating energy expenditure. Their physiologic effects, mediated at multiple target organs, are primarily to stimulate cell metabolism and activity. The vital roles of these hormones, particularly in development, differentiation, and maturation, are underscored by the severe mental retardation observed in infants with deficient thyroid hormone function during gestation. Thyroid hormones are derived from the amino acid tyrosine and are produced by the thyroid gland in response to stimulation by thyroid-stimulating hormone (TSH) produced by the anterior pituitary. TSH, in turn, is regulated by the hypophysiotropic peptide thyrotropin-releasing hormone (TRH) (Figure 4–1). Thyroid hormone production is also under regulation by dietary iodine.
Figure 4–1.
The hypothalamic-pituitary-thyroid axis. Thyrotropin-releasing hormone (TRH) is synthesized in parvicellular neurons of the paraventricular nucleus of the hypothalamus and released from nerve terminals in the median eminence, from where it is transported via the portal capillary plexus to the anterior pituitary. TRH binds to a G protein–coupled receptor in the anterior pituitary, leading to an increase in intracellular Ca2+ concentration, which in turn results in stimulation of exocytosis and release of thyroid-stimulating hormone (TSH) into the systemic circulation. TSH stimulates the thyroid gland to increase the synthesis and secretion of tetraiodothyronine (T4) and triiodothyronine (T3) into the circulation. T4 and T3 inhibit the secretion of thyrotropin both directly and indirectly by inhibiting the secretion of TRH. Additional factors that inhibit TSH release are glucocorticoids, somatostatin, and dopamine.
Functional Anatomy
The thyroid gland is a highly vascular, ductless alveolar (acinar) gland located in the anterior neck in front of the trachea. The gland weighs 10–25 g and consists of a right and left lobe connected by the isthmus. The cellular composition of the thyroid gland is diverse, including the following:
- Follicular (epithelial) cells, involved in thyroid hormone synthesis
- Endothelial cells lining the capillaries that provide the blood supply to the follicles
- Parafollicular or C cells, involved in the production of calcitonin, a hormone involved in calcium metabolism
- Fibroblasts, lymphocytes, and adipocytes
The main function of the thyroid gland is the synthesis and storage of thyroid hormone. The secretory or functional unit of the thyroid gland is the thyroid follicle, consisting of a layer of thyroid epithelial cells arranged around a large central cavity filled with colloid. Colloid makes up approximately 30% of the thyroid gland mass and contains a protein called thyroglobulin (Tg). Tg plays a central role in the synthesis and storage of thyroid hormone, as discussed later. The thyroid epithelial cells are morphologically and functionally polarized. That is, each side or compartment of the cell has specific functions pertaining to the synthesis of thyroid hormones and their release. The apical surface of the follicular cell faces the follicular lumen, where colloid is stored. The basolateral surface faces the interstitium and is therefore exposed to the bloodstream.
The polarity of the thyroid follicular cells is important in the overall function of the cell. Thyroid hormone synthesis requires the iodination of tyrosine residues on the protein Tg, which is stored in the colloid. This process occurs in the colloid at the apical plasma membrane, yet iodine is obtained from the circulation. Whereas thyroid hormone synthesis occurs on the apical side of the thyroid epithelial cell, hormone release occurs at the basolateral side of the cell. Thus, the polarity of the thyroid epithelial cells is critical for maintaining basolaterally located iodide uptake and T4 (tetraiodothyronine) deiodination, and apically located iodide efflux and iodination mechanisms.
The parafollicular cells surrounding the follicles synthesize and secrete the hormone calcitonin, and therefore they are frequently referred to as C cells. They are preferentially located in central regions of the thyroid gland lobes, where follicular cell activity is the greatest. Parafollicular cells secrete regulatory factors other than calcitonin that modulate follicular cell activity in a paracrine fashion.
The thyroid gland is highly vascularized with arterial blood derived from the superior and inferior thyroid arteries. The veins draining the thyroid gland form a plexus on the surface of the gland and on the front of the trachea and give rise to the superior, middle, and inferior thyroid veins, which drain into the internal jugular and innominate veins. The lymphatic vessels end in the thoracic and right lymphatic trunks. Innervation to the thyroid gland is provided through the middle and inferior cervical ganglia of the sympathetic nervous system.
Regulation of Biosynthesis, Storage, and Secretion of Thyroid Hormones
Thyroid hormone synthesis and release are under negative feedback regulation by the hypothalamic-pituitary-thyroid axis (see Figure 4–1). TRH is a tripeptide synthesized in the hypothalamus and released from nerve terminals in the median eminence from where it is transported through the portal capillary plexus to the anterior pituitary. TRH binds to cell membrane Gq/11 receptors on thyrotrophs of the anterior pituitary gland, where it activates phospholipase C, resulting in the hydrolysis of phosphatidylinositol bisphosphate and the generation of inositol triphosphate and diacylglycerol. This process leads to an increase in the intracellular Ca2+ concentration, resulting in stimulation of exocytosis and release of TSH into the systemic circulation.
TSH is transported in the bloodstream to the thyroid gland, where it binds to the TSH receptor located on the basolateral membrane of thyroid follicular epithelial cells. The TSH receptor is a cell membrane G protein–coupled receptor. Adequate function of the TSH receptor is critical in the development, growth, and function of the thyroid gland. Binding of TSH to its receptor initiates signaling through cyclic 3′,5′-adenosine monophosphate (cAMP), phospholipase C, and the protein kinase A signal transduction systems. Activation of adenylate cyclase, formation of cAMP, and activation of protein kinase A regulate iodide uptake and transcription of Tg, thyroid peroxidase (TPO), and the activity of the sodium-iodide (Na+/I−) symporter. Signaling through phospholipase C and intracellular Ca2+ regulate iodide efflux, H2O2 production, and Tg iodination. The TSH receptor is an important antigenic site involved in thyroid autoimmune disease. Autoantibodies to the receptor may act as agonists mimicking the actions of TSH in the case of Graves disease; or antagonists in the case of autoimmune hypothyroidism (Hashimoto thyroiditis).
TSH receptor activation results in stimulation of all of the steps involved in thyroid hormone synthesis, including iodine uptake and organification, production and release of iodothyronines from the gland, and promotion of thyroid growth. As part of this process, TSH regulates thyroidal uptake of nutrients as well as intracellular transport of specific proteins involved in thyroid hormone synthesis, storage, and release. These cellular events involve regulation of cellular metabolic processes, morphologic differentiation, cell proliferation, and protection from apoptosis. Specifically, the biologic effects of TSH include stimulation of gene transcription of the following:
- Na+/I− symporter, the protein involved in transporting and concentrating iodide in the thyroid epithelial cell
- Tg, the glycoprotein that serves as a scaffold for tyrosine iodination and thyroid hormone synthesis, as well as storage of thyroid hormone
- TPO, the enzyme involved in catalyzing the oxidation of iodide and its incorporation into tyrosine residues of Tg
- Thyroid hormones T4 and T3 (triiodothyronine)
As mentioned earlier, both growth and function of the thyroid are stimulated by the rise in cAMP produced by TSH stimulation. Therefore, continued stimulation of the cAMP pathway by binding to the TSH receptor causes hyperthyroidism and thyroid hyperplasia.
The production and release of thyroid hormones are under negative feedback regulation by the hypothalamic-pituitary-thyroid axis. The release of TSH is inhibited mainly by T3, produced by conversion of T4 to T3 in the hypothalamus, and in the anterior pituitary by type II deiodinase. The contribution of this intracellularly derived T3 in producing the negative feedback inhibition of TSH release is greater than that of T3 derived from the circulation. Other neuroendocrine mediators that inhibit TSH release include dopamine, somatostatin, and glucocorticoids at high levels, which produce partial suppression of TSH release (see Chapter 10).
Thyroglobulin, plays an important role in the synthesis and storage of thyroid hormone (Figure 4–2). Tg is a glycoprotein containing multiple tyrosine residues. It is synthesized in the thyroid follicular epithelial cells and secreted through the apical membrane into the follicular lumen, where it is stored in the colloid. A small amount of noniodinated Tg is also secreted through the basolateral membrane into the circulation. Although circulating levels of Tg can be detected under normal conditions, levels are elevated in diseases such as thyroiditis and Graves disease.
Figure 4–2.
Mechanism of iodide concentration by the thyroid gland. Iodide is transported into the cytosol of the follicular cell by a sodium-iodide (Na+/I−) symporter. Two Na+ ions are transported inside the thyroid follicular cell with each iodide molecule. Na+ moves down its concentration gradient, which is maintained by a Na+/K+-ATPase that constantly pumps Na+ out of the cytoplasm of the thyroid follicular epithelial cell maintaining the low intracytoplasmic Na+ concentrations. Iodide must reach the colloid space, where it is used for organification of thyroglobulin. This process is achieved by efflux through the iodide channel. One of the early effects of thyroid-stimulating hormone (TSH) binding to its receptor is the opening of these channels facilitating the leak of iodide into the extracellular space. This transcellular transport of iodide relies on the functional and morphological polarization of the thyroid follicular epithelial cell.
Tg can be considered a scaffold upon which thyroid hormone synthesis takes place. Once Tg is secreted into the follicular lumen, it undergoes major post-translational modification during the production of thyroid hormones. At the apical surface of the thyroid follicular epithelial cells, multiple tyrosine residues of Tg are iodinated, followed by coupling of some of the iodotyrosine residues to form T3 and T4.
The iodide required for thyroid hormone synthesis is readily absorbed from dietary sources, primarily from iodized salt, but also from seafood and plants grown in soil that is rich in iodine. Following its absorption, iodide is confined to the extracellular fluid, from which it is removed primarily by the thyroid (20%) and the kidneys (80%). The total excretion of iodide by the kidneys is approximately equal to daily intake. The balance between dietary intake and renal excretion preserves the total extracellular pool of iodide.
Iodide is concentrated in thyroid epithelial cells by an active, saturable, energy-dependent process mediated by a Na+/I− symporter located in the basolateral plasma membrane of the follicular cell (Figure 4–3). Additional tissues that express the Na+/I− symporter include the salivary glands, the gastric mucosa, the placenta, and the mammary glands. However, transport of iodine in these tissues is not under TSH regulation.
Figure 4–3.
Biochemistry of thyroid hormone synthesis. In the follicular lumen, tyrosine residues within the thyroglobulin matrix are iodinated by iodine (I+; formed by oxidation of I− by thyroid peroxidase). Iodine bonds to carbon 3 or to carbon 5 of the tyrosine residues of thyroglobulin in a process referred to as the organification of iodine. This iodination of specific tyrosines located on thyroglobulin yields monoiodinated tyrosine (MIT) and diiodinated tyrosine (DIT) residues that are enzymatically coupled to form triiodothyronine (T3) or tetraiodothyronine (T4). The coupling of iodinated tyrosine residues, either of 2 diiodotyrosines or of 1 monoiodotyrosine and 1 diiodotyrosine residue, is catalyzed by the enzyme thyroid peroxidase.
The iodination of Tg residues is a process that occurs at the apical membrane. Thus, once inside the cell, iodide must leave the follicular cell through apical efflux by an iodide-permeating mechanism consisting of a chloride-iodide transporting protein (iodide channel) located in the apical membrane of the thyroid follicular cell. The uptake, concentration, and efflux of iodide through the iodide channel are all a function of TSH-stimulated transepithelial transport of iodide. As already mentioned, this transcellular transport of iodide is possible because of the morphologic and functional polarization of the thyroid epithelial cell.
The transport of iodine through the Na+/I− symporter can be substituted by other substances including perchlorate and pertechnetate. Radiolabeled pertechnetate (99mTcO4) can be used for imaging of the thyroid gland. Moreover, the ability of the thyroid gland to accumulate iodine allows therapeutic administration of radioactive iodine leading to ablation of thyroid tissue, which is important in the treatment of thyroid malignancies. In the follicular lumen, tyrosine residues of Tg are iodinated by iodine (I+; formed by oxidation of I−by TPO) (see Figure 4–2). This reaction requires hydrogen peroxide, which is generated by a flavoprotein Ca++-dependent reduced nicotinamide adenine dinucleotide phosphate oxidase at the apical cell surface and serves as an electron acceptor in the reaction process. As shown in the figure, iodine bonds to carbon 3 or to carbon 5 of the tyrosine residues on Tg in a process referred to as the organification of iodine. This iodination of specific tyrosines located on Tg yields monoiodinated tyrosine (MIT) and diiodinated tyrosine (DIT) residues that are enzymatically coupled to form triiodothyronine (T3) or tetraiodothyronine (T4). The coupling of iodinated tyrosine residues, either of 2 DIT residues or of 1 MIT and 1 DIT residue, is catalyzed by the enzyme thyroid peroxidase. Because not all of the iodinated tyrosine residues undergo coupling, Tg stored in the follicular lumen contains MIT and DIT residues as well as formed T3 and T4 (Figure 4–4).
Figure 4–4.
Overview of the process of thyroid hormone synthesis in the thyroid follicular epithelial cell. Thyroid hormone synthesis involves concentration of iodide by the Na+/I− symporter and the transport of iodide through the epithelial cell and into the extracellular compartment within the follicular cells where it is oxidized to iodine by thyroid peroxidase and is then used in the iodination of thyroglobulin (Tg). Iodine organification is an extracellular process that takes place inside the thyroid follicles at the apical membrane surface facing the follicular lumen. Secretion of the hormone involves the endocytosis of colloid containing thyroglobulin followed by degradation of thyroglobulin and release of tetraiodothyronine (T4) and triiodothyronine (T3). Some of the T4 produced is deiodinated in the thyroid follicle to T3, which is then released into the bloodstream. In addition, intracellular deiodination provides a mechanism for recycling of iodide to participate in the synthesis of new TH at the apical cell surface. A small fraction of Tg is released from the follicular epithelial cell into the circulation. A fraction of T4 produced is deiodinated to T3 prior to release from the thyroid. TSHR, thyroid-stimulating hormone receptor. (Reproduced with permission from Kopp PA. Reduce, recycle, reuse-iodotyrosine deiodinase in thyroid iodide metabolism. N Engl J Med. 2008;358(17):1856–1859. Copyright © Massachusetts Medical Society. All rights reserved.)
As mentioned previously, TSH controls the energy-dependent uptake and concentration of iodide by the thyroid gland and its transcellular transport through the follicular epithelial cell. However, iodine metabolism within the thyroid can also be regulated independently of TSH. This mechanism is important when plasma iodide levels are elevated (15–20-fold above normal) because this elevation inhibits the organic binding of iodine within the thyroid. This autoregulatory phenomenon consisting of inhibition of the organification of iodine by elevated circulating levels of iodide is known as the Wolff-Chaikoff effect. This effect lasts for a few days and is followed by the so-called escape phenomenon, at which point the organification of intra-thyroidal iodine resumes and the normal synthesis of T4 and T3 returns. The escape phenomenon results from a decrease in the inorganic iodine concentration inside the thyroid gland from downregulation of the Na+/I− symporter. This relative decrease in intra-thyroidal inorganic iodine allows the TPO–H2O2 system to resume normal activity. The mechanisms responsible for the acute Wolff-Chaikoff effect have not been elucidated but may be caused by the formation of organic iodocompounds within the thyroid.
The preceding sections have described the steps involved in the synthesis of thyroid hormone, TSH regulation of the iodination of tyrosine residues on Tg, and coupling of the iodinated residues for the formation of thyroid hormones (see Figure 4–4). TSH also regulates the release of thyroid hormones from the thyroid gland. The synthesis of thyroid hormone takes place in the colloid space. As mentioned previously, the apical surface of the follicular epithelial cell faces the colloid and not the interstitial space, and thus has no access to the bloodstream. Therefore, thyroid hormone release involves endocytosis of vesicles containing Tg from the apical surface of the follicular cell. The vesicles fuse with follicular epithelial phagolysosomes, leading to proteolytic digestion and cleavage of Tg. In addition to the thyroid hormones T4 and T3, the products of this reaction include iodinated tyrosine residues (MIT and DIT). MIT and DIT are deiodinated intracellularly, and iodide is transported by apical efflux into the follicular colloid space, where it is reused in thyroid hormone synthesis. T4 and T3 are released from the basolateral membrane into the circulation. The thyroid gland releases greater amounts of T4 than T3, so plasma concentrations of T4 are 40-fold higher than those of T3 (90 vs 2 nM). Most of the circulating T3 is formed peripherally by deiodination of T4, a process that involves the removal of iodine from carbon 5 on the outer ring of T4. Thus, T4 acts as a prohormone for T3. Although this deiodination occurs predominantly in the liver, some occurs in the thyroid follicular epithelial cell itself. This intrathyroidal deiodination of T4
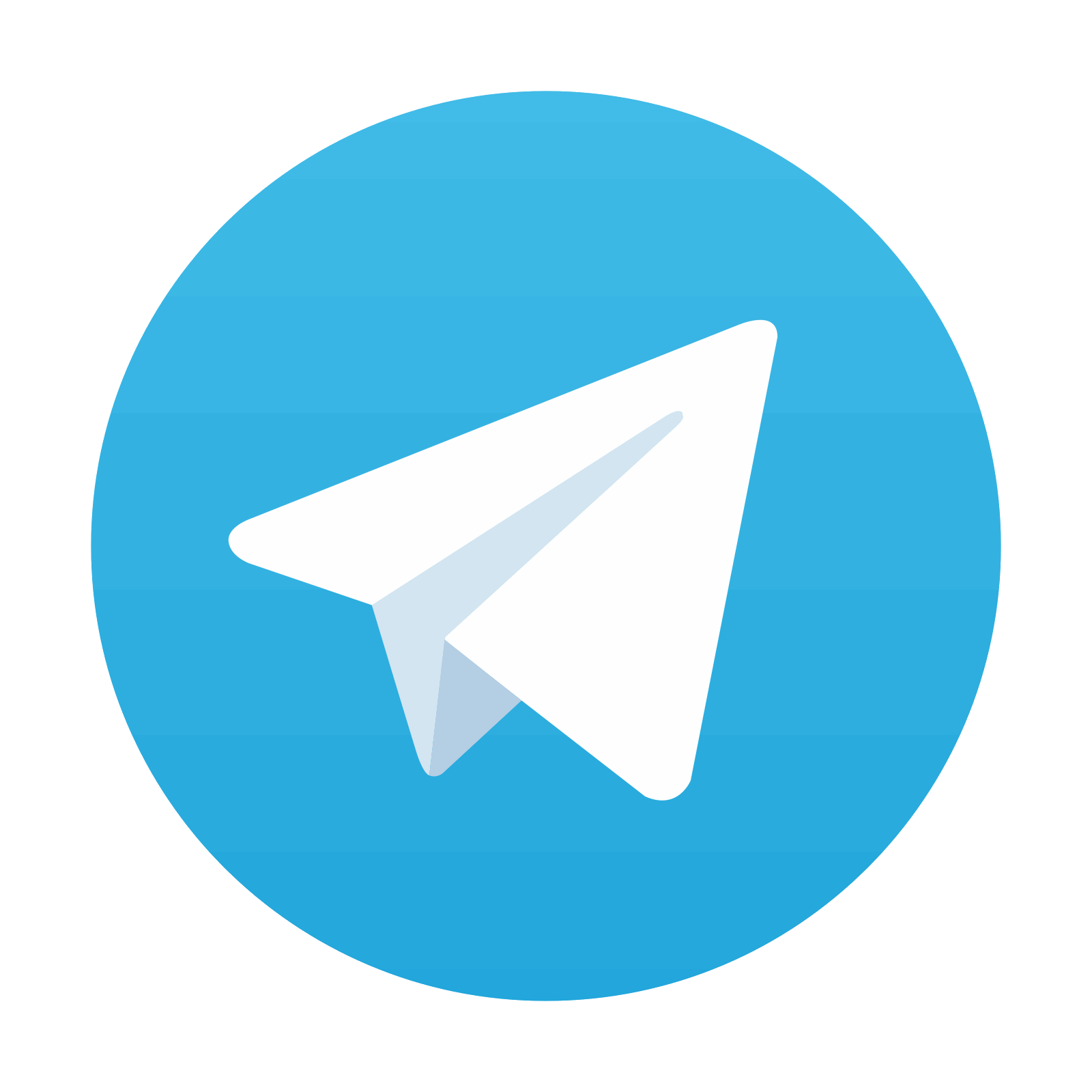
Stay updated, free articles. Join our Telegram channel

Full access? Get Clinical Tree
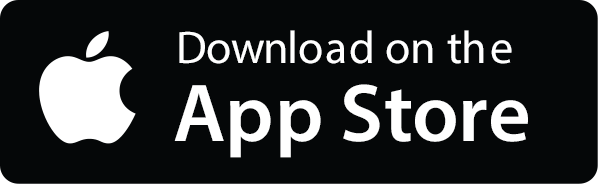
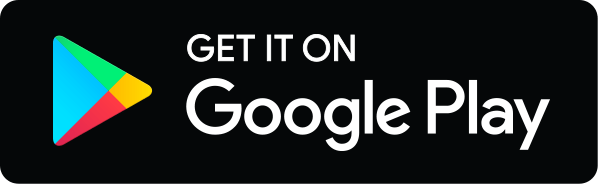
