KEY POINTS
Assessing urgency of repair is essential to developing the appropriate management plan. Although emergent repair carries greater operative risk than does elective repair, any inappropriate delay of repair risks death.
The clinical progression of an aortic aneurysm is continued expansion and eventual rupture. Hence, regular noninvasive imaging studies, as part of a lifelong surveillance plan, are necessary to ensure long-term patient health. Even small asymptomatic aneurysms should be routinely imaged to assess overall growth and yearly rate of expansion.
Endovascular repair devices are approved for the treatment of descending thoracic aortic aneurysms, and some of the newer devices are also approved for the treatment of aortic trauma and penetrating aortic ulcer.
Practice guidelines were recently published that have helped to standardize the decision-making process and select an appropriate surgical intervention, as well as to standardize the use of imaging studies for patients with thoracic aortic disease.
Ascending aortic aneurysms that are symptomatic or >5.5 cm should be repaired. This threshold is lowered for patients with connective tissue disorders.
Surgical repair involves the development of a patient-tailored plan based on careful preoperative medical evaluation. When appropriate, optimizing a patient’s health status—to mitigate existing comorbidities—is important before surgical intervention.
The development and use of surgical adjuncts like antegrade selective cerebral perfusion and cerebrospinal fluid drainage have significantly reduced the morbidity rates traditionally associated with complex aortic repair.
Proximal aortic dissection is a life-threatening condition, and immediate operative repair is generally indicated, although definitive aortic repair may be delayed until after severe malperfusion has been treated.
ANATOMY OF THE AORTA
The aorta consists of two major segments—the proximal aorta and the distal aorta—whose anatomic characteristics affect both the clinical manifestations of disease in these segments and the selection of treatment strategies for such disease (Fig. 22-1). The proximal aortic segment includes the ascending aorta and the transverse aortic arch. The ascending aorta begins at the aortic valve and ends at the origin of the innominate artery. The first portion of the ascending aorta is the aortic root, which includes the aortic valve annulus and the three sinuses of Valsalva; the coronary arteries originate from two of these sinuses. The aortic root joins the tubular portion of the ascending aorta at the sinotubular ridge. The transverse aortic arch is the area from which the brachiocephalic branches arise. The distal aortic segment includes the descending thoracic aorta and the abdominal aorta. The descending thoracic aorta begins distal to the origin of the left subclavian artery and extends to the diaphragmatic hiatus, where it joins the abdominal aorta. The descending thoracic aorta gives rise to multiple bronchial and esophageal branches, as well as to the segmental intercostal arteries, which provide circulation to the spinal cord.
Figure 22-1.
Illustration of normal thoracic aortic anatomy. The brachiocephalic vessels arise from the transverse aortic arch and are used as anatomic landmarks to define the aortic regions. The ascending aorta is proximal to the innominate artery, whereas the descending aorta is distal to the left subclavian artery.
The volume of blood that flows through the thoracic aorta at high pressure is far greater than that found in any other vascular structure. For this reason, any condition that disrupts the integrity of the thoracic aorta, such as aortic dissection, aneurysm rupture, or traumatic injury, can have catastrophic consequences.
Historically, open surgical repair of such conditions has been an intimidating undertaking associated with significant morbidity and mortality. Strategies for protecting the brain and spinal cord during such repairs have become critical in preventing devastating complications. In recent years, endovascular therapy for thoracic aortic disease in selected patients has become accepted practice, producing fewer adverse outcomes than traditional approaches do.
THORACIC AORTIC ANEURYSMS
Aortic aneurysm is defined as a permanent, localized dilatation of the aorta to a diameter that is at least 50% greater than is normal at that anatomic level.1 The annual incidence of thoracic aortic aneurysms is estimated to be 5.9 per 100,000 persons.2 The clinical manifestations, methods of treatment, and treatment results in patients with aortic aneurysms vary according to the cause and the aortic segment involved. Causes of thoracic aortic aneurysms include degenerative disease of the aortic wall, aortic dissection, aortitis, infection, and trauma. Aneurysms can be localized to a single aortic segment, or they can involve multiple segments. Thoracoabdominal aortic aneurysms, for example, involve both the descending thoracic aorta and the abdominal aorta. In the most extreme cases, the entire aorta is aneurysmal; this condition is often called mega-aorta.
Aortic aneurysms can be either “true” or “false.” True aneurysms can take two forms: fusiform and saccular. Fusiform aneurysms are more common and can be described as symmetrical dilatations of the aorta. Saccular aneurysms are localized outpouchings of the aorta. False aneurysms, also called pseudoaneurysms, are leaks in the aortic wall that are contained by the outer layer of the aorta and/or the periaortic tissue; they are caused by disruption of the aortic wall and lead blood to collect in pouches of fibrotic tissue.
Aneurysms of the thoracic aorta consistently increase in size and eventually progress to cause serious complications. These include rupture, which is usually a fatal event. Therefore, aggressive treatment is indicated in all but the poorest surgical candidates. Small, asymptomatic thoracic aortic aneurysms can be followed, especially in high-surgical-risk patients, and can be treated surgically later if symptoms or complications develop, or if progressive enlargement occurs. Meticulous control of hypertension is the primary medical treatment for patients with small, asymptomatic aneurysms.
Elective resection with graft replacement is indicated in asymptomatic patients with an aortic diameter of at least twice normal in the involved segment (5–6 cm in most thoracic segments). Elective repair is contraindicated by extreme operative risk due to severe coexisting cardiac or pulmonary disease and by other conditions that limit life expectancy, such as malignancy. An emergency operation is performed for any patient in whom a ruptured aneurysm is suspected.
Patients with thoracic aortic aneurysm often have coexisting aneurysms of other aortic segments. A common cause of death after repair of a thoracic aortic aneurysm is rupture of a different aortic aneurysm. Therefore, staged repair of multiple aortic segments often is necessary. As with any major operation, careful preoperative evaluation for coexisting disease and subsequent medical optimization are important for successful surgical treatment.
An alternative to traditional open repair of a descending thoracic aortic aneurysm is endovascular stent grafting. Certain anatomic criteria need to be satisfied for this treatment option to be considered, including the presence of at least a 2-cm landing zone of healthy aortic tissue proximally and distally to the aneurysm to be excluded. Although data on long-term outcomes are still lacking, endovascular repair of a descending thoracic aortic aneurysm has become an accepted practice that produces excellent midterm results.
The normal aorta derives its elasticity and tensile strength from the medial layer, which contains approximately 45 to 55 lamellae of elastin, collagen, smooth muscle cells, and ground substance. Elastin content is highest within the ascending aorta, as would be expected because of its compliant nature, and decreases distally into the descending and abdominal aorta. Maintenance of the aortic matrix involves complex interactions among smooth muscle cells, macrophages, proteases, and protease inhibitors. Any alteration in this delicate balance can lead to aortic disease.
Thoracic aortic aneurysms have a variety of causes (Table 22-1). Although these disparate pathologic processes differ in biochemical and histologic terms, they share the final common pathway of progressive aortic expansion and eventual rupture.
Nonspecific medial degeneration Aortic dissection Genetic disorders Marfan syndrome Loeys-Dietz syndrome Ehlers-Danlos syndrome Familial aortic aneurysms Aneurysms-Osteoarthritis syndrome Congenital bicuspid aortic valve Bovine aortic arch Poststenotic dilatation Infection Aortitis Takayasu arteritis Giant cell arteritis Rheumatoid aortitis Trauma |
Hemodynamic factors clearly contribute to the process of aortic dilatation. The vicious cycle of increasing diameter and increasing wall tension, as characterized by Laplace’s law (tension = pressure × radius), is well established. Turbulent blood flow is also recognized as a factor. Poststenotic aortic dilatation, for example, occurs in some patients with aortic valve stenosis or coarctation of the descending thoracic aorta. Hemodynamic derangements, however, are only one piece of a complex puzzle.
Atherosclerosis is commonly cited as a cause of thoracic aortic aneurysms. However, although atherosclerotic disease often is found in conjunction with aortic aneurysms, the notion that atherosclerosis is a distinct cause of aneurysm formation has been challenged. In most thoracic aortic aneurysms, atherosclerosis appears to be a coexisting process, rather than the underlying cause.
Research into the pathogenesis of abdominal aortic aneurysms has focused on the molecular mechanisms of aortic wall degeneration and dilatation. For example, imbalances between proteolytic enzymes (e.g., matrix metalloproteinases) and their inhibitors contribute to abdominal aortic aneurysm formation. Building on these advances, current investigations are attempting to determine whether similar inflammatory and proteolytic mechanisms are involved in thoracic aortic disease, in hope of identifying potential molecular targets for pharmacologic therapy.
Nonspecific medial degeneration is the most common cause of thoracic aortic disease. Histologic findings of mild medial degeneration, including fragmentation of elastic fibers and loss of smooth muscle cells, are expected in the aging aorta. However, an advanced, accelerated form of medial degeneration leads to progressive weakening of the aortic wall, aneurysm formation, and eventual dissection, rupture, or both. The underlying causes of medial degenerative disease remain unknown.
An aortic dissection usually begins as a tear in the inner aortic wall, which initiates a progressive separation of the medial layers and creates two channels within the aorta. This event profoundly weakens the outer wall. As the most common catastrophe involving the aorta, dissection represents a major, distinct cause of thoracic aortic aneurysms and is discussed in detail in the second half of this chapter.
Marfan syndrome is an autosomal dominant genetic disorder characterized by a specific connective tissue defect that leads to aneurysm formation. The phenotype of patients with Marfan syndrome typically includes a tall stature, high palate, joint hypermobility, eye lens disorders, mitral valve prolapse, and aortic aneurysms. The aortic wall is weakened by fragmentation of elastic fibers and deposition of extensive amounts of mucopolysaccharides (a process previously called cystic medial degeneration or cystic medial necrosis). Patients with Marfan syndrome have a mutation in the fibrillin gene located on the long arm of chromosome 15. The traditionally held view is that abnormal fibrillin in the extracellular matrix decreases connective tissue strength in the aortic wall and produces abnormal elasticity, which predisposes the aorta to dilatation from wall tension caused by left ventricular ejection impulses.3 More recent evidence, however, shows that the abnormal fibrillin causes degeneration of the aortic wall matrix by increasing the activity of transforming growth factor beta (TGF-β).4 Between 75% and 85% of patients with Marfan syndrome have dilatation of the ascending aorta and annuloaortic ectasia (dilatation of the aortic sinuses and annulus).5 Such aortic abnormalities are the most common cause of death among patients with Marfan syndrome.6 Marfan syndrome also is frequently associated with aortic dissection.
Loeys-Dietz syndrome is phenotypically distinct from Marfan syndrome. It is characterized as an aneurysmal syndrome with widespread systemic involvement. Loeys-Dietz syndrome is an aggressive, autosomal dominant condition that is distinguished by the triad of arterial tortuosity and aneurysms, hypertelorism (widely spaced eyes), and bifid uvula or cleft palate. It is caused by heterozygous mutations in the genes encoding TGF-β receptors.7,8 Patients with Loeys-Dietz syndrome—including young children—are at increased risk of aortic rupture and aortic dissection; diameter-based thresholds of repair tend to be lower for patients with this syndrome than for patients with other connective tissue disorders.
Ehlers-Danlos syndrome includes a spectrum of inherited connective tissue disorders of collagen synthesis. The subtypes represent differing defective steps of collagen production. Vascular type Ehlers-Danlos syndrome is characterized by an autosomal dominant defect in type III collagen synthesis, which can have life-threatening cardiovascular manifestations. Spontaneous arterial rupture, usually involving the mesenteric vessels, is the most common cause of death in these patients. Thoracic aortic aneurysms and dissections are less commonly associated with Ehlers-Danlos syndrome, but when they do occur, they pose a particularly challenging surgical problem because of the reduced integrity of the aortic tissue.9 An Ehlers-Danlos variant of periventricular heterotopia associated with joint and skin hyperextensibility and aortic dilation has been described as being caused by mutations in the gene encoding filamin A (FLNA), an actin-binding protein that links the smooth muscle cell contractile unit to the cell surface.10
Families without the heritable connective tissue disorders described earlier also can be affected by genetic conditions that cause thoracic aortic aneurysms. In fact, it is estimated that at least 20% of patients with thoracic aortic aneurysms and dissections have a genetic predisposition to them. The involved mutations are characterized by autosomal dominant inheritance with decreased penetrance and variable expression. Thus far, mutations involving the genes for TGF-β receptors (TGFβR1 and TGFβR2), TGF-β2, β-myosin (MYH11 and MYLK), and α-smooth muscle cell actin (ACTA2)have been identified as causes of familial thoracic aortic aneurysms and dissection.11,12,13ACTA2 mutations are present in approximately 14% of families with familial thoracic aortic aneurysms and dissections.
Aneurysms-osteoarthritis syndrome is a recently identified autosomal dominant disorder. Patients with this syndrome suffer from aortic and arterial aneurysms, arterial tortuosity, aortic dissection, mild craniofacial abnormalities, and early onset osteoarthritis. Aneurysms-osteoarthritis syndrome is caused by mutations in the gene encoding SMAD3, a transcription factor for TGF-β. Affected patients have a high incidence of aortic dissection, which often occurs in a mildly dilated aorta (4–4.5 cm) and causes sudden death.14
Bicuspid aortic valve is the most common congenital malformation of the heart or great vessels, affecting up to 2% of Americans.15 Compared to patients with a normal, trileaflet aortic valve, patients with bicuspid aortic valve have an increased incidence of ascending aortic aneurysm formation and, often, a faster rate of aortic enlargement.16 The location of the fused leaflet, or raphe, may be predictive of aortic dilation and other abnormalities.17 About 50% to 70% of adults with bicuspid aortic valve, but without significant valve dysfunction, have echocardiographically detectable aortic dilatation.18,19 This dilatation usually is limited to the ascending aorta and root.20 Dilation occasionally is found in the arch and only rarely in the descending or abdominal aorta. In addition, aortic dissection occurs 10 times more often in patients with bicuspid valves than in the general population.21 Recent findings suggest that aneurysms associated with bicuspid aortic valve have a fundamentally different pathobiologic cause than aneurysms that occur in patients with trileaflet valves.22
Although the exact mechanism responsible for aneurysm formation in patients with bicuspid aortic valve remains unclear, evidence suggests that these patients have a congenital connective tissue abnormality that predisposes the aorta to medial degeneration.22,23,24,25,26,27,28 For example, fibrillin 1 content is significantly lower and matrix metalloproteinase activity is significantly higher in the aortic media in patients with bicuspid aortic valve than in persons with a normal, tricuspid aortic valve.22,23,24 Further, the process of medial degeneration in patients with bicuspid aortic valve may be exacerbated by the presence of chronic turbulent flow through the deformed valve.
Bovine aortic arch—a common origin of the innominate and left common carotid arteries—has been considered a normal anatomic variant. Recent studies from Yale University have identified a higher prevalence of bovine aortic arch in patients with thoracic aortic disease; an association was found between this anomaly and a generalized increase in aortic aneurysmal disease (without any predisposition to a particular aortic region). However, bovine aortic arch was not associated distinctly with bicuspid aortic valve or aortic dissection, but with a higher mean aortic growth rate: 0.29 cm/year in patients with bovine aortic arch, compared with 0.09 cm/year in controls. Therefore, bovine aortic arch may be better characterized as a precursor of aortic aneurysm than as a simple normal anatomic variant.29 Further studies are needed to delineate the underlying mechanism for this association.
Primary infection of the aortic wall resulting in aneurysm formation is rare. Although these lesions are termed mycotic aneurysms, the responsible pathogens usually are bacteria rather than fungi. Bacterial invasion of the aortic wall may result from bacterial endocarditis, endothelial trauma caused by an aortic jet lesion, or extension from an infected laminar clot within a preexisting aneurysm. The most common causative organisms are Staphylococcus aureus, Staphylococcus epidermidis, Salmonella, and Streptococcus.30,31 Unlike most other causes of thoracic aortic aneurysms, which generally produce fusiform aneurysms, infection often produces saccular aneurysms located in areas of aortic tissue destroyed by the infectious process.
Although syphilis was once the most common cause of ascending aortic aneurysms, the advent of effective antibiotic therapy has made syphilitic aneurysms a rarity in developed nations. In other parts of the world, however, syphilitic aneurysms remain a major cause of morbidity and mortality. The spirochete Treponema pallidum causes an obliterative endarteritis of the vasa vasorum that results in medial ischemia and loss of the elastic and muscular elements of the aortic wall. The ascending aorta and arch are the most commonly involved areas. The emergence of HIV infection in the 1980s was associated with a substantial increase in the incidence of syphilis in both HIV-positive and HIV-negative patients. Because syphilitic aortitis often presents 10 to 30 years after the primary infection, the incidence of associated aneurysms may increase in the near future.
In patients with preexisting degenerative thoracic aortic aneurysms, localized transmural inflammation and subsequent fibrosis can develop. The dense aortic infiltrate responsible for the fibrosis consists of lymphocytes, plasma cells, and giant cells. The cause of the intense inflammatory reaction is unknown. Although the severe inflammation is a superimposed problem rather than a primary cause, its onset within an aneurysm can further weaken the aortic wall and precipitate expansion.
Systemic autoimmune disorders also cause thoracic aortitis. Aortic Takayasu arteritis generally produces obstructive lesions related to severe intimal thickening, but associated medial necrosis can lead to aneurysm formation. In patients with giant cell arteritis (temporal arteritis), granulomatous inflammation may develop that involves the entire thickness of the aortic wall, causing intimal thickening and medial destruction. Rheumatoid aortitis is an uncommon systemic disease that is associated with rheumatoid arthritis and ankylosing spondylitis. The resulting medial inflammation and fibrosis can affect the aortic root, causing annular dilatation, aortic valve regurgitation, and ascending aortic aneurysm formation.
Pseudoaneurysms of the thoracic aorta usually represent chronic leaks that are contained by surrounding tissue and fibrosis. By definition, the wall of a pseudoaneurysm is not formed by intact aortic tissue; rather, the wall develops from organized thrombus and associated fibrosis. Pseudoaneurysms can arise from primary defects in the aortic wall (e.g., after trauma or contained aneurysm rupture) or from anastomotic or cannulation site leaks that occur after cardiovascular surgery. Anastomotic pseudoaneurysms can be caused by technical problems or by deterioration of the native aortic tissue, graft material, or suture. Commonly, they occur in patients with Marfan syndrome.32 Tissue deterioration usually is related to either progressive degenerative disease or infection. Improvements in sutures, graft materials, and surgical techniques have decreased the incidence of thoracic aortic pseudoaneurysms. Should thoracic aortic pseudoaneurysms occur, they typically require expeditious surgical or other intervention because they are associated with a high incidence of morbidity and rupture.
Treatment decisions in cases of thoracic aortic aneurysm are guided by our current understanding of the clinical history of these aneurysms, which classically is characterized as progressive aortic dilatation and eventual dissection, rupture, or both. An analysis by Elefteriades of data from 1600 patients with thoracic aortic disease has helped quantify these well-recognized risks.33 Average expansion rates were 0.07 cm/y in ascending aortic aneurysms and 0.19 cm/y in descending thoracic aortic aneurysms. As expected, aortic diameter was a strong predictor of rupture, dissection, and mortality. For thoracic aortic aneurysms >6 cm in diameter, annual rates of catastrophic complications were 3.6% for rupture, 3.7% for dissection, and 10.8% for death. Critical diameters, at which the incidence of expected complications significantly increased, were 6.0 cm for aneurysms of the ascending aorta and 7.0 cm for aneurysms of the descending thoracic aorta; the corresponding risks of rupture after reaching these diameters were 31% and 43%, respectively.34
Certain types of aneurysms have an increased propensity for expansion and rupture. For example, aneurysms in patients with Marfan or Loeys-Dietz syndrome dilate at an accelerated rate and rupture or dissect at smaller diameters than non–connective tissue disorder-related aneurysms. Before the era of surgical treatment for aortic aneurysms, this aggressive form of aortic disease resulted in an average life expectancy of 32 years for Marfan patients; aortic root complications caused the majority of deaths.35 Saccular aneurysms, which commonly are associated with aortic infection and typically affect only a discrete small section of the aorta, tend to grow more rapidly than fusiform aneurysms, which are associated with more widespread degenerative changes and generally affect a larger section of the aorta.
One common clinical scenario deserves special attention. A moderately dilated ascending aorta (i.e., 4–5 cm) often is encountered during aortic valve replacement or coronary artery bypass operations. The clinical history of these ectatic ascending aortas has been defined by several studies. Michel and colleagues36 studied patients whose ascending aortic diameters were >4 cm at the time of aortic valve replacement; 25% of these patients required reoperation for ascending aortic replacement. Prenger and colleagues37 reported that aortic dissection occurred in 27% of patients who had aortic diameters of >5 cm at the time of aortic valve replacement. Recently, attention has been directed toward whether or not a mildly dilated aortic root should be replaced in patients with bicuspid aortic valve who are undergoing isolated valve replacement, and at what threshold to intervene. Although this is a controversial issue, many surgeons believe that the tendency toward late aortic dilatation in these patients warrants aggressive treatment.38,39 Current practice guidelines indicate that such early replacement should be considered in these patients when the ascending aorta is 4.0 to 4.5 cm.40
In many patients with thoracic aortic aneurysms, the aneurysm is discovered incidentally when imaging studies are performed for unrelated reasons. Therefore, patients often are asymptomatic at the time of diagnosis. However, thoracic aortic aneurysms that initially go undetected eventually create symptoms and signs that correspond with the segment of aorta that is involved. These aneurysms have a wide variety of manifestations, including compression or erosion of adjacent structures, aortic valve regurgitation, distal embolism, and rupture.
Initially, aneurysmal expansion and impingement on adjacent structures causes mild, chronic pain. The most common symptom in patients with ascending aortic aneurysms is anterior chest discomfort; the pain is frequently precordial in location but may radiate to the neck and jaw, mimicking angina. Aneurysms of the ascending aorta and transverse aortic arch can cause symptoms related to compression of the superior vena cava, the pulmonary artery, the airway, or the sternum. Rarely, these aneurysms erode into the superior vena cava or right atrium, causing acute high-output failure. Expansion of the distal aortic arch can stretch the recurrent laryngeal nerve, which results in left vocal cord paralysis and hoarseness. Descending thoracic and thoracoabdominal aneurysms frequently cause back pain localized between the scapulae. When the aneurysm is largest in the region of the aortic hiatus, it may cause middle back and epigastric pain. Thoracic or lumbar vertebral body erosion typically causes severe, chronic back pain; extreme cases can present with spinal instability and neurologic deficits from spinal cord compression. Although mycotic aneurysms have a peculiar propensity to destroy vertebral bodies, spinal erosion also occurs with degenerative aneurysms. Descending thoracic aortic aneurysms may cause various degrees of airway obstruction, manifesting as cough, wheezing, stridor, or pneumonitis. Pulmonary or airway erosion presents as hemoptysis. Compression and erosion of the esophagus cause dysphagia and hematemesis, respectively. Thoracoabdominal aortic aneurysms can cause duodenal obstruction or, if they erode through the bowel wall, gastrointestinal bleeding. Jaundice due to compression of the liver or porta hepatis is uncommon. Erosion into the inferior vena cava or iliac vein presents with an abdominal bruit, widened pulse pressure, edema, and heart failure.
Ascending aortic aneurysms can cause displacement of the aortic valve commissures and annular dilatation. The resulting deformation of the aortic valve leads to progressively worsening aortic valve regurgitation. In response to the volume overload, the heart remodels and becomes increasingly dilated. Patients with this condition may present with progressive heart failure, a widened pulse pressure, and a diastolic murmur.
Thoracic aortic aneurysms—particularly those involving the descending and thoracoabdominal aorta—are commonly lined with friable, atheromatous plaque and mural thrombus. This debris may embolize distally, causing occlusion and thrombosis of the visceral, renal, or lower-extremity branches.
Patients with ruptured thoracic aortic aneurysms often experience sudden, severe pain in the anterior chest (ascending aorta), upper back or left chest (descending thoracic aorta), or left flank or abdomen (thoracoabdominal aorta). When ascending aortic aneurysms rupture, they usually bleed into the pericardial space, producing acute cardiac tamponade and death. Descending thoracic aortic aneurysms rupture into the pleural cavity, producing a combination of severe hemorrhagic shock and respiratory compromise. External rupture is extremely rare; saccular syphilitic aneurysms have been observed to rupture externally after eroding through the sternum.
Diagnosis and characterization of thoracic aneurysms require imaging studies, which also provide critical information that guides the selection of treatment options. Although the best choice of imaging technique for the thoracic and thoracoabdominal aorta is somewhat institution-specific, varying with the availability of imaging equipment and expertise, efforts have been made to standardize key elements of image acquisition and reporting. Recent practice guidelines40 recommend that aortic imaging reports plainly state the location of aortic abnormalities (including calcification and the extent to which abnormalities extend into branch vessels), the maximum external aortic diameters (rather than internal, lumen-based diameters), internal filling defects, and any evidence of rupture. Whenever possible, all results should be compared to those of prior imaging studies.
Plain radiographs of the chest, abdomen, or spine often provide enough information to support the initial diagnosis of thoracic aortic aneurysm. Ascending aortic aneurysms produce a convex shadow to the right of the cardiac silhouette. The anterior projection of an ascending aneurysm results in the loss of the retrosternal space in the lateral view. An aneurysm may be indistinguishable from elongation and tortuosity.41 Above all, chest radiographs (CXRs) may appear normal in patients with thoracic aortic disease and, thus, cannot exclude the diagnosis of aortic aneurysm. Aortic root aneurysms, for example, often are hidden within the cardiac silhouette. Plain CXRs may reveal convexity in the right superior mediastinum, loss of the retrosternal space, or widening of the descending thoracic aortic shadow, which may be highlighted by a rim of calcification outlining the dilated aneurysmal aortic wall. Aortic calcification also may be seen in the upper abdomen on a standard radiograph made in the anteroposterior or lateral projection (Fig. 22-2). Once a thoracic aortic aneurysm is detected on plain radiographs, additional studies are required to define the extent of aortic involvement.
Ascending aortic aneurysms are commonly discovered during echocardiography in patients presenting with symptoms or signs of aortic valve regurgitation. Both transthoracic and transesophageal echocardiography provide excellent visualization of the ascending aorta, including the aortic root.42 Transesophageal echocardiography also allows visualization of the descending thoracic aorta but is not ideal for evaluating the transverse aortic arch (which is obscured by air in the tracheobronchial tree) or the upper abdominal aorta. Effective echocardiography requires considerable technical skill, both in obtaining adequate images and in interpreting them. This imaging modality has the added benefit of assessing cardiac function and revealing any other abnormalities that may be present. During ultrasound evaluation of a suspected infrarenal abdominal aortic aneurysm, if a definitive neck cannot be identified at the level of the renal arteries, the possibility of thoracoabdominal aortic involvement should be suspected and investigated by using other imaging modalities. Caution should be exercised while interpreting aneurysm dimensions from ultrasound imaging because intraluminal measurements are often reported, whereas external measurements are usually used in other imaging modalities.
Computed tomographic (CT) scanning is widely available, provides visualization of the entire thoracic and abdominal aorta, and permits multiplanar and 3-dimensional aortic reconstructions. Consequently, CT is the most common—and arguably the most useful—imaging modality for evaluating thoracic aortic aneurysms.43 In addition to establishing the diagnosis, CT provides information about an aneurysm’s location, extent, anatomic anomalies, and relationship to major branch vessels. CT is particularly useful in determining the absolute diameter of the aorta, especially in the presence of a laminated clot, and also detects aortic calcification. Contrast-enhanced CT provides information about the aortic lumen and can detect mural thrombus, aortic dissection, inflammatory periaortic fibrosis, and mediastinal or retroperitoneal hematoma due to contained aortic rupture. To increase consistency and ensure uniform reporting, current practice guidelines suggest that measurements be taken perpendicular to blood flow and at standard anatomic locations40 (Fig. 22-3); this should reduce the likelihood of erroneous measurements, especially during serial imaging surveillance.
Figure 22-3.
Current practice guidelines40 seek to standardize the reporting of aortic diameters by indicating key locations of measurement. These include (1) the sinuses of Valsalva, (2) the sinotubular junction, (3) the mid-ascending aorta, (4) the proximal aortic arch at the origins of the innominate artery, (5) the mid-aortic arch, which is between the left common carotid and left subclavian arteries, (6) the proximal descending thoracic aorta, which begins at the isthmus (approximately 2 cm distal to the origins of the left subclavian artery), (7) the mid-descending thoracic artery, (8) the aorta at the diaphragm, and (9) the abdominal aorta at the origins of the celiac axis. (Used with permission of Baylor College of Medicine.)
The major disadvantage of contrast-enhanced CT scanning is the possibility of contrast-induced acute renal failure in patients who are at risk (e.g., patients with preexisting renal disease or diabetes).44 If possible, surgery is performed ≥1 day after contrast administration to allow time to observe renal function and to permit diuresis of the contrast agent. If renal insufficiency occurs or is worsened, elective surgery is postponed until renal function returns to normal or stabilizes.
Magnetic resonance angiography (MRA) is becoming widely available and can facilitate visualization of the entire aorta. This modality produces aortic images comparable to those produced by contrast-enhanced CT but does not necessitate exposure to ionizing radiation. In addition, MRA offers excellent visualization of branch vessel details, and it is useful in detecting branch vessel stenosis.45 However, MRA is limited by high expense and a susceptibility to artifacts created by ferromagnetic materials, and gadolinium—the contrast agent for MRA—may be linked to nephrogenic systemic fibrosis and acute renal failure in patients with advanced renal insufficiency.46 Furthermore, the MRA environment is not appropriate for many critically ill patients, and unlike CT imaging, MRA imaging is suboptimal in patients with extensive aortic calcification.
Although catheter-based contrast aortography was previously considered the gold standard for evaluating thoracic aortic disease, cross-sectional imaging (i.e., CT and MRA) has largely replaced this modality. Technologic improvements have enabled CT and MRA to provide excellent aortic imaging while causing less morbidity than catheter-based studies do, so CT and MRA are now the primary modes for evaluating thoracic aortic disease. Today, the use of invasive aortography in patients with thoracic aortic disease is generally limited to those undergoing endovascular therapies or when other types of studies are contraindicated or have not provided satisfactory results.
Unlike standard aortography, cardiac catheterization continues to play an important role in diagnosis and preoperative planning, especially in patients with ascending aortic involvement. Proximal aortography can reveal not only the status of the coronary arteries and left ventricular function but also the degree of aortic valve regurgitation, the extent of aortic root involvement, coronary ostial displacement, and the relationship of the aneurysm to the arch vessels.
The value of the information one can obtain from catheter-based diagnostic studies should be weighed against the established limitations and potential complications of such studies. A key limitation of aortography is that it images only the lumen and may therefore underrepresent the size of large aneurysms that contain laminated thrombus. Manipulation of intraluminal catheters can result in embolization of laminated thrombus or atheromatous debris. Proximal aortography carries a 0.6% to 1.2% risk of stroke. Other risks include allergic reaction to contrast agent, iatrogenic aortic dissection, and bleeding at the arterial access site. In addition, the volumes of contrast agent required to adequately fill large aneurysms can cause significant renal toxicity. To minimize the risk of contrast nephropathy, patients receive periprocedural intravenous (IV) fluids for hydration, mannitol for diuresis, and acetylcysteine.47,48 As with contrast-enhanced CT, surgery is performed ≥1 day after angiography whenever possible to ensure that renal function has stabilized or returned to baseline.
Once a thoracic aortic aneurysm is detected, management begins with patient education, particularly if the patient is asymptomatic, because aortic disease may progress rapidly and unexpectedly in some patients. A detailed medical history is collected, a physical examination is performed, and a systematic review of medical records is carried out to clearly assess the presence or absence of pertinent symptoms and signs, despite any initial denial of symptoms by the patient. Signs of genetic diseases such as Marfan syndrome or Loeys-Dietz syndrome are thoroughly reviewed. If clinical criteria are met for such a genetic condition, confirmatory laboratory tests are conducted. Patients with such genetic diseases are best treated in a dedicated aortic clinic where they can be appropriately followed up. Surveillance imaging and aggressive blood pressure control are the mainstays of initial management for asymptomatic patients. When patients become symptomatic or their aneurysms grow to meet certain size criteria, the patients become surgical candidates.
Although long-term data are still lacking, endovascular therapy has become an accepted treatment for thoracic aortic aneurysms.49 Its role in treating proximal aortic disease and thoracoabdominal aortic aneurysms remains experimental. Nonetheless, endoluminal stenting is approved by the U.S. Food and Drug Administration for the treatment of isolated descending thoracic aortic aneurysms, and some newer devices are approved for the treatment of blunt aortic injury and penetrating aortic ulcer. In practice, however, the off-label application of aortic stent grafts is widespread and accounts for well over half their use.50 Endovascular approaches may be helpful in emergent aneurysm repair, such as for patients with aortic rupture.51 Recently, endovascular therapy has evolved to include hybrid repairs, which combine open “debranching” techniques (to reroute branching vessels) with endovascular aortic repair. Despite these advances, for the repair of aneurysms with proximal aortic involvement and of thoracoabdominal aortic aneurysms, open procedures remain the gold standard and preferred approach.
Cross-sectional imaging with reconstruction is critical when one is evaluating a thoracic aneurysm, determining treatment strategy, and planning necessary procedures. Note that, commonly, patients with a thoracic aortic aneurysm also have a remote aneurysm.2 In such cases, the more threatening lesion usually is addressed first. In many patients, staged operative procedures are necessary for complete repair of extensive aneurysms involving the ascending aorta, transverse arch, and descending thoracic or thoracoabdominal aorta.52 When the descending segment is not disproportionately large (compared with the proximal aorta) and is not causing symptoms, the proximal aortic repair is carried out first. An important benefit of this approach is that it allows treatment of valvular and coronary artery occlusive disease at the first operation.
Proximal aneurysms (proximal to the left subclavian artery) usually are addressed via a sternotomy approach. Aneurysms involving the descending thoracic aorta are evaluated in terms of criteria (described later) for potential endovascular repair; those unsuitable for an endovascular approach are repaired with open techniques through a left thoracotomy. A CT scan can reveal detailed information about aortic calcification and luminal thrombus. These details are important in preventing embolization during surgical manipulation.
Thoracic aortic aneurysms are repaired to prevent fatal rupture. Therefore, on the basis of the natural history studies and other data, practice guidelines for thoracic aortic disease40 recommend elective operation in asymptomatic patients when the diameter of an ascending aortic aneurysm is >5.5 cm, when the diameter of a descending thoracic aortic aneurysm is >6.0 cm, or when the rate of dilatation is >0.5 cm/y. In patients with connective tissue disorders such as Marfan and Loeys-Dietz syndromes, the threshold for operation is based on a smaller aortic diameter (4.0–5.0 cm for the ascending aorta and 5.5 to 6.0 cm for the descending thoracic aorta). For women with connective tissue disorders who are considering pregnancy, prophylactic aortic root replacement is considered because the risk of aortic dissection or rupture increases at an aortic diameter of 4.0 cm or greater. For low-risk patients with chronic aortic dissection, descending thoracic repair is recommended at an aortic diameter of 5.5 cm or greater. For patients undergoing aortic valve replacement or repair, smaller ascending aortic aneurysms (>4.5 cm) are considered for concomitant repair.
The acuity of presentation is a major factor in decisions about the timing of surgical intervention. Many patients are asymptomatic at the time of presentation, so there is time for thorough preoperative evaluation and improvement of their current health status, such as through smoking cessation and other optimization programs. In contrast, patients who present with symptoms may need urgent operation. Symptomatic patients are at increased risk of rupture and warrant expeditious evaluation. The onset of new pain in patients with known aneurysms is especially concerning, because it may herald significant expansion, leakage, or impending rupture. Emergent intervention is reserved for patients who present with aneurysm rupture or superimposed acute dissection.53
As noted earlier, endovascular repair of thoracic aortic aneurysms has become an accepted treatment option in selected patients, particularly patients with isolated degenerative descending thoracic aortic aneurysms; in fact, practice guidelines recommend that endovascular repair be strongly considered for patients with descending thoracic aneurysm at an aortic diameter of 5.5 cm (which is slightly below the 6.0-cm threshold for open repair).40 For endovascular repairs to produce optimal outcomes, several anatomic criteria must be met. For one, the proximal and distal neck diameters should fall within a range that will allow proper sealing. Also, the proximal and distal landing zones should ideally be at least 20 mm long so that an appropriate seal can be made. Note that the limiting structures proximally and distally are the brachiocephalic vessels and celiac axis, respectively. Another anatomic limitation for this therapy relates to vascular access: The femoral and iliac arteries have to be wide enough to accommodate the large sheaths necessary to deploy the stent grafts, although newer devices use a smaller sheath (or are “sheathless” self-deployed stent grafts) to accommodate smaller arteries. Tortuosity of the iliac vessels and abdominal aorta can make these procedures technically challenging. Occasionally, a “side graft” anastomosed to the iliac artery through a retroperitoneal incision is used because of poor distal access. When any of these anatomic criteria are not met, an open approach is preferable to an endovascular approach.
Of note, attempts have been made to extend the use of endovascular therapy to aortic arch aneurysms and thoracoabdominal aortic aneurysms. Although reports of purely endovascular repair of the aortic arch remain limited, Greenberg and colleagues54 have reported their experience with a large series of purely endovascular thoracoabdominal aortic repairs. Additionally, there have been numerous reports of small series of off-label, experimental hybrid procedures that involve debranching the aortic arch or the visceral vessels of the abdominal aorta, followed by endovascular exclusion of the aneurysm. The majority of hybrid approaches involve repairing the aortic arch. In its simplest form, hybrid arch repair involves an open bypass from the left subclavian to the left common carotid artery, which is followed by deliberate coverage of the origins of the left subclavian artery by the stent graft. In its most complex form, hybrid arch repair involves rerouting all of the brachiocephalic vessels, followed by proximal placement of the stent graft in the ascending aorta and extending repair distally into the aortic arch and descending thoracic aorta.
The patients who theoretically may benefit more from an endovascular approach than from traditional open techniques are those who are of advanced age or have significant comorbidities. For example, the open repair of a descending thoracic aortic aneurysm can result in significant pulmonary morbidity. Therefore, patients with borderline pulmonary reserve may better tolerate an endovascular procedure than standard open repair. In contrast, patients with significant intraluminal atheroma may be better served by an open approach because of the risk of embolization and stroke posed by catheter manipulation. Similarly, patients with connective tissue disorders generally are not considered candidates for elective endovascular repair. Endovascular repair in patients with connective tissue disorders has produced poor results, which are mainly due to progressive dilatation, stent graft migration, and endoleak.55
Given the impact of comorbid conditions on perioperative complications, a careful preoperative assessment of physiologic reserve is critical in assessing operative risk. Therefore, most patients undergo a thorough evaluation—with emphasis on cardiac, pulmonary, and renal function—before undergoing elective surgery.56,57
Coronary artery disease is common in patients with thoracic aortic aneurysm and is responsible for a substantial proportion of early and late postoperative deaths in such patients. Similarly, valvular disease and myocardial dysfunction have important implications when one is planning anesthetic management and surgical approaches for aortic repair. Transthoracic echocardiography is a satisfactory noninvasive method for evaluating both valvular and biventricular function. Dipyridamole-thallium myocardial scanning identifies regions of myocardium that have reversible ischemia, and this test is more practical than exercise testing in older patients with concomitant lower-extremity peripheral vascular disease. Cardiac catheterization and coronary arteriography are performed in patients who have evidence of coronary disease—as indicated by either the patient’s history or the results of noninvasive studies—or who have a left ventricular ejection fraction of ≤30%. If significant valvular or coronary artery disease is identified before a proximal aortic operation, the disease can be addressed directly during the procedure. Patients who have asymptomatic distal aortic aneurysms and severe coronary occlusive disease undergo percutaneous transluminal angioplasty or surgical revascularization before the aneurysmal aortic segment is replaced.
Pulmonary function screening with arterial blood gas measurement and spirometry is routinely performed before thoracic aortic operations. Patients with a forced expiratory volume in 1 second of >1.0 L and a partial pressure of carbon dioxide of <45 mmHg are considered surgical candidates. In suitable patients, borderline pulmonary function can be improved by implementing a regimen that includes smoking cessation, weight loss, exercise, and treatment of bronchitis for a period of 1 to 3 months before surgery. Although surgery is not withheld from patients with symptomatic aortic aneurysms and poor pulmonary function, adjustments in operative technique should be made to maximize these patients’ chances of recovery. In such patients, preserving the left recurrent laryngeal nerve, the phrenic nerves, and diaphragmatic function is particularly important.
Renal function is assessed preoperatively by measuring serum electrolyte, blood urea nitrogen, and creatinine levels. Information about kidney size and perfusion can be obtained from the imaging studies used to evaluate the aorta.
Obtaining accurate information about baseline renal function has important therapeutic and prognostic implications. For example, perfusion strategies and perioperative medications are adjusted according to renal function. Patients with severely impaired renal function frequently require at least temporary hemodialysis after surgery. These patients also have a mortality rate that is significantly higher than normal. Patients with thoracoabdominal aortic aneurysms and poor renal function secondary to severe proximal renal occlusive disease undergo renal artery endarterectomy, stenting, or bypass grafting during the aortic repair.
Traditional open operations to repair proximal aortic aneurysms—which involve the ascending aorta, transverse aortic arch, or both—are performed through a midsternal incision and require cardiopulmonary bypass. The best choice of aortic replacement technique depends on the extent of the aneurysm and the condition of the aortic valve. The spectrum of operations (Fig. 22-4) ranges from simple graft replacement of the tubular portion of the ascending aorta (Fig. 22-4A) to graft replacement of the entire proximal aorta, including the aortic root, and reattachment of the coronary arteries and brachiocephalic branches. The options for treating aortic valve disease, repairing aortic aneurysms, and maintaining perfusion during repair procedures each deserve detailed consideration (Table 22-2).
Options for treating aortic valve disease Aortic valve annuloplasty (annular plication) Aortic valve replacement with mechanical or biologic prosthesis Aortic root replacement Composite valve graft Aortic homograft Stentless porcine root Pulmonary autograft (Ross procedure) Valve-sparing techniques Options for graft repair of the aortic aneurysm Patch aortoplasty Ascending replacement only Beveled hemiarch replacement Total arch replacement with reattachment of brachiocephalic branches Total arch replacement with bypass grafts to the brachiocephalic branches (Y-graft approaches) Elephant trunk technique with island reattachment Elephant trunk technique with Y-graft approach Perfusion options Standard cardiopulmonary bypass Profound hypothermic circulatory arrest without adjuncts Hypothermic circulatory arrest with adjuncts Retrograde cerebral perfusion Antegrade cerebral perfusion Balloon perfusion catheters Right axillary artery cannulation Innominate artery cannulation Combined antegrade and retrograde cerebral perfusion |
Figure 22-4.
Illustrations of proximal aortic repairs in which the native aortic root is left intact. A. Graft replacement of the tubular portion of the ascending aorta with the aortic arch left intact. B. Hemiarch beveled graft replacement, in which the ascending aorta and a portion of the lesser curvature of the aortic arch are replaced. C. A modified hemiarch with additional graft replacement of the innominate artery. D. Patch repair of the aortic arch. E. Traditional total arch replacement using an island approach to reattach the brachiocephalic vessels. F. The branched graft approach, which replaces the brachiocephalic vessels by following their original anatomic location. G. The elephant trunk approach with a concomitant island brachiocephalic artery reattachment. Contemporary Y-graft arch repairs include H. the single Y-graft approach, I. the double Y-graft approach, J. the elephant trunk approach with a single Y-graft, and K. the elephant trunk approach with a double Y-graft. (Used with permission of Baylor College of Medicine.)
Many patients undergoing proximal aortic operations have aortic valve disease that requires concomitant surgical correction. When such disease is present and the sinus segment is normal, separate repair or replacement of the aortic valve and graft replacement of the tubular segment of the ascending aorta are carried out. In such cases, mild to moderate valve regurgitation with annular dilatation can be addressed by plicating the annulus with mattress sutures placed below each commissure, thereby preserving the native valve. In patients with more severe valvular regurgitation or with valvular stenosis, the valve is replaced with a stented biologic or mechanical prosthesis. Mechanical prostheses necessitate following a lifelong anticoagulation regimen. Separate replacement of the aortic valve and ascending aorta is not performed in patients with Marfan syndrome, because progressive dilatation of the remaining sinus segment eventually leads to complications that necessitate reoperation. Therefore, patients with Marfan syndrome or those with annuloaortic ectasia require some form of aortic root replacement.58
In many cases, the aortic root is replaced with a mechanical or biologic graft that has both a valve and an aortic conduit. Currently, three graft options are commercially available: composite valve grafts, which consist of a bileaflet mechanical valve attached to a polyester tube graft; aortic root homografts, which are harvested from cadavers and cryopreserved; and stentless porcine aortic root grafts.59,60 Another option for surgeons is to construct a bioprosthetic composite valve graft during the operation by suturing a stented tissue valve to a polyester graft tube.
Although select patients may be offered the Ross procedure, in which the patient’s pulmonary artery root is excised and placed in the aortic position and then the right ventricular outflow tract is reconstructed by using a cryopreserved pulmonary homograft, this option is rarely used. This is largely because it is a technically demanding procedure, and there are concerns about the potential for autograft dilatation in patients with connective tissue disorders.61
An additional option is valve-sparing aortic root replacement, which has evolved substantially during the past decade.62 The valve-sparing technique that is currently favored is called aortic root reimplantation and involves excising the aortic sinuses, attaching a prosthetic graft to the patient’s annulus (Fig. 22-5), and resuspending the native aortic valve inside the graft. The superior hemodynamics of the native valve and the avoidance of anticoagulation are major advantages of the valve-sparing approach. Long-term results in carefully selected patients have been excellent.63 Although the durability of this procedure in patients with either Marfan syndrome or bicuspid aortic valve has been questioned, reports suggest that long-term durability is possible for patients with Marfan syndrome who undergo the procedure at experienced centers.64,65 Further, acceptable midterm outcomes have been reported for patients with bicuspid aortic valve.66 Patients who have structural leaflet deterioration or excessive annular dilatation are typically deemed unsuitable for valve-sparing repair.
Figure 22-5.
Illustration of our current valve-sparing procedure for replacing the aortic root and ascending aorta for treatment of A. aortic root aneurysm. B. The ascending aorta is opened after cardiopulmonary bypass and cardioplegic arrest are established and the distal ascending aorta is clamped. The diseased aortic tissue (including the sinuses of Valsalva) is excised. Buttons of surrounding tissue are used to mobilize the origins of the coronary arteries. C. A synthetic graft is sewn to the distal ascending aorta with continuous suture. D. After the distal anastomosis is completed, six sutures reinforced with Teflon pledgets are placed in the plane immediately below the aortic valve annulus. E. The subannular sutures are placed through the base of a synthetic aortic root graft, which is then is parachuted down around the valve.F. After the root graft is cut to an appropriate length, the valve commissures and leaflets are positioned within the graft. The annular sutures are then tied. G. Each of the three commissures is then secured near the top of the graft. H. The supra-annular aortic tissue is sewn to the graft in continuous fashion. I. The button surrounding the origin of the left main coronary artery is sewn to an opening cut in the root graft. J. The two aortic grafts are sewn together with continuous suture. K. The button surrounding the origin of the right coronary artery is sewn to an opening cut in the root graft. L. The completed valve-sparing aortic root replacement and graft repair of the ascending aorta is shown. (Used with permission of Baylor College of Medicine.)
Regardless of the type of conduit used, aortic root replacement requires reattaching the coronary arteries to openings in the graft. In the original procedure described by Bentall and De Bono,67 this was accomplished by suturing the intact aortic wall surrounding each coronary artery to the openings in the graft. The aortic wall was then wrapped around the graft to establish hemostasis. However, this technique frequently produced leaks at the coronary reattachment sites that eventually led to pseudoaneurysm formation. The Cabrol modification, in which a separate, small tube graft is sutured to the coronary ostia and the main aortic graft, achieves tension-free coronary anastomoses and reduces the risk of pseudoaneurysm formation.68 Kouchoukos’s button modification of the Bentall procedure is currently the most widely used technique.69 The aneurysmal aorta is excised, and buttons of aortic wall are left surrounding both coronary arteries, which are then mobilized and sutured to the aortic graft (Fig. 22-6). The coronary suture lines may be reinforced with polytetrafluoroethylene felt or pericardium to enhance hemostasis. When the coronary arteries cannot be mobilized adequately because of extremely large aneurysms or scarring from previous surgery, the Cabrol technique or a related modification can be used. Another option, originally described by Zubiate and Kay,70 is the construction of bypass grafts by using interposition saphenous vein or synthetic grafts.
Figure 22-6.
Illustration of a modified Bentall procedure for replacing the aortic root and ascending aorta. The aortic valve and entire ascending aorta, including the sinuses of Valsalva, have been replaced by a mechanical composite valve graft. The coronary arteries with buttons of surrounding aortic tissue have been mobilized and are being reattached to openings in the aortic graft.
Several options are also available for handling aneurysms that extend into the transverse aortic arch (Fig. 22-4). The surgical approach depends on the extent of involvement and the need for cardiac and cerebral protection. Saccular aneurysms that arise from the lesser curvature of the distal transverse arch and that encompass <50% of the aortic circumference can be treated by patch graft aortoplasty. For fusiform aneurysms, when the distal portion of the arch is a reasonable size, a single, beveled replacement of the lower curvature (hemiarch) is performed. More extensive arch aneurysms require total replacement involving a distal anastomosis to the proximal descending thoracic aorta and separate reattachment of the brachiocephalic branches. The brachiocephalic vessels are reattached to one or more openings made in the graft, or if these vessels are aneurysmal, they are replaced with separate, smaller grafts. Recently, Y-graft approaches to aortic arch repair have been introduced71 that essentially debranch the brachiocephalic vessels and move them forward. This permits the distal anastomosis to be brought forward and aids in hemostasis. When the aneurysm involves the entire arch and extends into the descending thoracic aorta, it is approached by using Borst’s elephant trunk technique of staged total arch replacement.72 The distal anastomosis may be constructed by using a collared graft to accommodate any discrepancy in aortic diameter73 and is performed such that a portion of the graft is left suspended within the proximal descending thoracic aorta (Fig. 22-7). During a subsequent operation, this “trunk” is used to facilitate repair of the descending thoracic aorta through a thoracotomy incision. This technique permits access to the distal portion of the graft during the second operation without the need for dissection around the distal transverse aortic arch; this reduces the risk of injuring the left recurrent laryngeal nerve, esophagus, and pulmonary artery if an open approach is used at the second stage. As described in the section on hybrid repair of arch aneurysms (see later), the elephant trunk can be completed by using a hybrid endovascular approach (Fig. 22-8) in certain settings.
Figure 22-7.
Illustration of a contemporary Y-graft approach to total arch replacement for aortic arch aneurysm. A. The proximal portions of the brachiocephalic arteries are exposed. B. The first two branches of the graft are sewn end-to-end to the transected left subclavian and left common carotid arteries. The proximal ends of the transected brachiocephalic arteries are ligated. C. A balloon-tipped perfusion cannula is placed inside the double Y-graft and used to deliver antegrade cerebral perfusion. After systemic circulatory arrest is initiated, the innominate artery is clamped, transected, and sewn to the distal end of the main graft. D. The proximal aspect of the Y-graft is clamped. This directs flow from the axillary artery to all three brachiocephalic arteries. The arch is then replaced with a collared elephant trunk graft. E. The distal anastomosis between the elephant trunk graft and the aorta is created between the innominate and left common carotid arteries. The collared graft accommodates any discrepancy in aortic diameter. F. The aortic graft is clamped, and a second limb from the arterial inflow tubing of the cardiopulmonary bypass circuit is used to deliver systemic perfusion through a side-branch of the arch graft while the proximal portion of the ascending aorta is replaced. Once the proximal aortic anastomosis is completed, the main trunk of the double Y-graft is cut to an appropriate length, and the beveled end is then sewn to an oval opening created in the right anterolateral aspect of the ascending aortic graft, which completes the repair G. (Adapted from LeMaire et al,73 Fig. 2. Used with permission. Copyright The Society of Thoracic Surgeons.)
Figure 22-8.
Illustration of Borst’s elephant trunk technique using a contemporary Y-graft approach. A. Stage 1: The proximal repair includes replacing the ascending aorta and entire arch, with Y-graft reattachment of the brachiocephalic vessels. The distal anastomosis is facilitated by using a collared elephant trunk graft to accommodate the larger diameter of the distal aorta. A section of the graft is left suspended within the proximal descending thoracic aorta. B. Stage 2: The distal repair uses the floating “trunk” for the proximal anastomosis. C. An alternate “hybrid” approach may be used in patients with less extensive distal aortic disease. Endovascular stent grafts are placed within the elephant trunk to complete the repair. (Used with permission of Baylor College of Medicine.)
Like the operations themselves, perfusion strategies used during proximal aortic surgery depend on the extent of the repair. Aneurysms that are isolated to the ascending segment can be replaced by using standard cardiopulmonary bypass and distal ascending aortic clamping. This provides constant perfusion of the brain and other vital organs during the repair. Aneurysms involving the transverse aortic arch, however, cannot be clamped during the repair, which necessitates the temporary withdrawal of cardiopulmonary bypass support; this is called circulatory arrest. To protect the brain and other vital organs during the circulatory arrest period, hypothermia must be initiated before pump flow is stopped. However, hypothermia is not without risk, and coagulopathy is associated with deep levels of hypothermia (below 20°C), which have been traditionally used in open arch repair. Recently, more moderate levels of hypothermia (often between 22°C and 24°C) have been introduced that appear to decrease risks associated with deep hypothermia while still providing sufficient brain protection. Nonetheless, although brief periods of circulatory arrest generally are well tolerated, even this recently modified technique continues to have substantial limitations; as the duration of circulatory arrest increases, the well-recognized risks of brain injury and death increase dramatically. Additionally, some authors have raised the concern that reducing the degree of hypothermia narrows the safety margin that deep hypothermia provides, because it increases the risk of ischemic complications involving the spinal cord, kidneys, and other organs that now receive less hypothermic protection.74
Because of the inherent complexity of aortic arch repairs and their general tendency to require longer periods of hypothermic circulatory arrest, two cerebral perfusion strategies—retrograde cerebral perfusion (RCP) and antegrade cerebral perfusion (ACP)—were developed to supplement this process by delivering cold, oxygenated blood to the brain and further reduce the risks associated with repair. Retrograde cerebral perfusion involves directing blood from the cardiopulmonary bypass circuit into the brain through the superior vena cava.75 However, RCP is thought to be less beneficial than ACP,76 and although it may be helpful in the retrograde flushing of air and debris from the arch, most centers have stopped using RCP.
In contrast, ACP delivers blood directly into the brachiocephalic arteries to maintain cerebral flow. Although its use was cumbersome in the past, contemporary ACP techniques (Fig. 22-9) have been simplified and commonly involve cannulating either the right axillary artery or the innominate artery and subsequent connection to the cardiopulmonary bypass circuit.77,78 Often, a small section of graft is used as a conduit to ease cannulation, but there remains a small procedure-related risk of brachial plexus or vascular injury. Upon initiation, cold blood is delivered into the brain via the right common carotid artery. Note that, with this technique, blood flow to the left side of the brain requires an intact circle of Willis.
Figure 22-9.
Illustration of a contemporary technique for delivering antegrade cerebral perfusion during aortic arch repair. A. A graft sewn to the right axillary artery is used to return oxygenated blood from the cardiopulmonary bypass circuit. B. After adequate hypothermia is established, the innominate artery is occluded with a tourniquet (inset) so that flow is diverted to the right common carotid artery, which maintains cerebral circulation. (Images adapted from Gravlee GP, Davis RF, Stammers AH, et al, eds. Cardiopulmonary Bypass: Principles and Practice, 3rd ed. Philadelphia: Lippincott Williams & Wilkins; 2008, Chap. 32, Fig. 1A,B. Copyright Wolters Kluwer Health.)
Methods to help determine the adequacy of unilateral ACP to deliver cerebral cross-circulation include preoperative imaging and intraoperative monitoring. Our preferred method of intraoperative monitoring is brain near-infrared spectroscopy (NIRS), which measures cerebral oxygenation. If NIRS monitoring indicates inadequate perfusion, an additional perfusion catheter can be inserted into the left common carotid artery to provide blood flow to the left side of the brain. Because of the use of more moderate levels of hypothermia, some groups supplement ACP with additional perfusion strategies that provide flow to the descending aorta during arch repair.79,80
Experience with purely endovascular treatment of proximal aortic disease remains limited and only investigational. The unique anatomy of the aortic arch and the need for uninterrupted cerebral perfusion pose difficult challenges. There are reports of the use of “homemade” grafts to exclude arch aneurysms; however, these grafts are highly experimental at this time. For example, in 1999, Inoue and colleagues81 reported placing a triple-branched stent graft in a patient with an aneurysm of the aortic arch. The three brachiocephalic branches were positioned by placing percutaneous wires in the right brachial, left carotid, and left brachial arteries. The patient underwent two subsequent procedures: surgical repair of a right brachial pseudoaneurysm and placement of a distal stent graft extension to control a major perigraft leak. Since then, efforts to employ endovascular techniques in the treatment of the proximal aorta have been essentially limited to the use of approved devices for off-label indications, such as the exclusion of pseudoaneurysms in the ascending aorta.
Unlike purely endovascular approaches, hybrid repairs of the aortic arch have entered the mainstream clinical arena, although they remain controversial. Hybrid arch repairs involve some form of “debranching” of the brachiocephalic vessels (which are not unlike Y-graft approaches), followed by endovascular exclusion of some or all of the aortic arch (Fig. 22-10). Although this technique has many variants, they often involve sewing a branched graft to the proximal ascending aorta with the use of a partial aortic clamp. The branches of the graft are then sewn to the arch vessels. Once the arch is “debranched,” the arch aneurysm can be excluded with an endograft. Commonly, a zone 0 approach (Fig. 22-11) is undertaken, in which the proximal end of the endograft lies between the ascending aorta and the origins of the innominate artery. Other hybrid approaches aim to extend repair into the distal arch and descending thoracic aorta (see later). The arguments for using a hybrid approach to treat aortic arch aneurysms include the elimination of cardiopulmonary bypass, circulatory arrest, and cardiac ischemia, although in practice, these adjuncts are frequently used during hybrid proximal aortic repairs.82
Figure 22-10.
Illustration of a hybrid arch repair. A. A distal arch aneurysm, which extends into the proximal aspect of the descending thoracic aorta, is shown. B. The brachiocephalic vessels are debranched onto a double Y-graft, and a separate graft is used as a conduit for antegrade endovascular deployment of the stent-graft. C. The completed repair. The proximal landing zone of the endograft is within zone 0. (Used with permission from Baylor College of Medicine.)
Figure 22-11.
Illustration of the Criado landing zones, which are used to describe aortic anatomy during thoracic endovascular repair. The arch is the short segment that includes the origins of the three brachiocephalic arteries—the innominate artery, the left common carotid artery, and the left subclavian artery. Zone 0 includes the ascending aorta and the origin of the innominate artery. Zone 1 includes the origin of the left common carotid artery. Zone 2 includes the left subclavian artery origin. Zone 3 is a short section of the aorta that comprises the 2 cm immediately distal to the origin of the left subclavian artery, and Zone 4 begins where Zone 3 ends. (Used with permission from Baylor College of Medicine.)
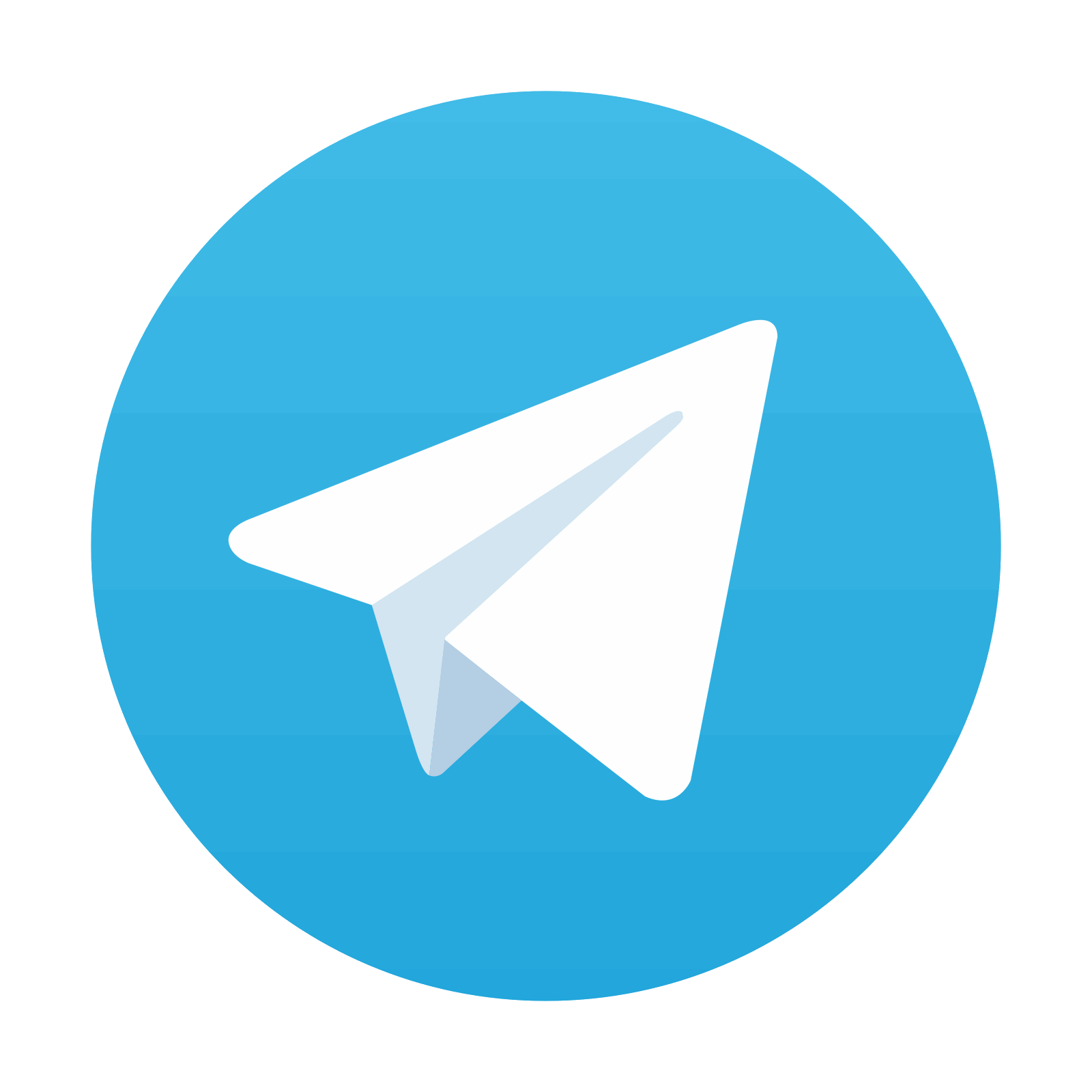
Stay updated, free articles. Join our Telegram channel

Full access? Get Clinical Tree
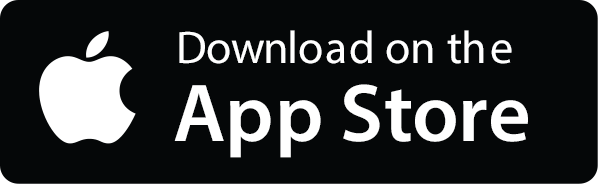
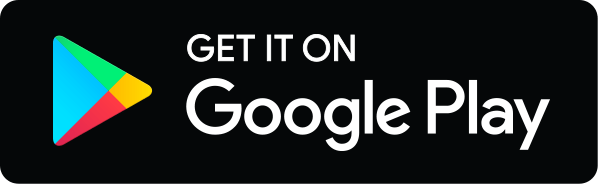
