The Parietal Cell
It has been more than 100 years since it was suggested that the secretory canaliculus of the parietal cell was the site of gastric acid secretion by Camillo Golgi. The pump responsible for acid secretion was identified some 20 years ago, and, recently, the high-resolution crystals of the SERCA ATPase have rationalized many of the structure-function studies on this enzyme. However, analysis of the complexity of the processes that regulate acid secretion by the parietal cell in terms of signaling pathways that alter cell morphology, the regulation of trafficking of the pump to the apical membrane, and activation of the necessary KCl conductance is only now becoming possible with the advent of modern cell and molecular biology and genomics and proteomics. Much remains to be discovered.
Early work on understanding parietal cell biology was based on data derived from the exteriorized dog flap in a hemi-chamber. More exact physiologic data were obtained on isolated amphibian mucosae in Ussing chambers. The major technical breakthrough in defining the stimulatory pathways in the mammalian parietal cell was the development of the rabbit gastric gland preparation with measurement of acid secretion by uptake of the weak-base 14C-aminopyrine.
In amphibia and birds, the acid-secreting cell is called the oxyntic cell; in mammals it is the parietal cell. These are epithelial cells, characterized by a difference between their mucosal or apical surface and their serosal or basolateral surface. These two domains are separated by a specialized region of the membrane, the tight junction.
The major function of the parietal cell at the neck of the gastric gland is the polarized secretion of HCl across its mucosal surface; in some species, this cell also secretes intrinsic factor, a protein vital for the absorption of cyanocobalamin. More mature parietal cells closer to the base of the gastric gland are also able to secrete Cl−, resulting in water flow by coupling this Cl− flux to Na+ flux across the tight junction. This secretion of water independent of acid may be useful in flushing out secreted pepsinogen from the lumen of the gland in this region.
As has been discussed, acid secretion is a regulated process, and the target for this regulation is the parietal cell, with a variety of receptors on its cell surface. But beyond this cellular target is the proton pump itself, the H,K ATPase. All natural stimuli and inhibitors converge to activate or inhibit this P-type ATPase. The parietal cell has developed extraordinary measures to be able to regulate the act of acid secretion. Regulation of acid secretion at the cellular
level involves three stages: activation of receptors on the basolateral surface, cytoplasmic signaling events consequent to ligand interaction with these receptors, and thence regulation of the H,K ATPase itself and a pathway enabling KCl efflux into the secretory canaliculus.
level involves three stages: activation of receptors on the basolateral surface, cytoplasmic signaling events consequent to ligand interaction with these receptors, and thence regulation of the H,K ATPase itself and a pathway enabling KCl efflux into the secretory canaliculus.
Development of the parietal cell
The parietal cell is an epithelial cell derived from stem cells in the isthmus of the gastric gland. These cells give rise to progenitor cells, which differentiate into parietal cells, forming three helical chains spiraling down to the base of the gland. Markers of parietal cell differentiation are an abundance of mitochondria and the presence of the H,K ATPase. Although the parietal cell itself is a terminally differentiated cell, there are differences between the parietal cells in the neck of the gland and in the lower part of the gland.
For example, stimulation of acid secretion affects the superficial cells more than the deeper cells. Also, the deeper cells, although having similar levels of the gastric H,K ATPase, also express the NaKCl2 cotransporter (NKCC2), which the more superficial cells do not. This finding implies that the deeper cells can act as Cl− secreting cells as well as HCl secreting cells. Perhaps there is a need for fluid secretion, independent of acid, for elution of secreted pepsinogen.
Morphology of the parietal cell
The morphology of the parietal cell is unique. It is conical, with a small apical and large basolateral membrane. Thirty-four percent of cell volume is occupied by mitochondria dedicated to the synthesis of ATP as an energy source for acid secretion. A large percentage of resting cell volume is also occupied by smooth-surfaced membranes called tubulovesicles that, when observed by freeze-smash electron microscopy, are actually elongated tubules. Also, there is a small infolding even in the resting cell of the apical membrane named the secretory canaliculus.
![]() Electron micrographs of a resting and stimulated parietal cell showing the conversion of the cytoplasmic tubulovesicles to the microvilli of the secretory canaliculus. |
On stimulation, the tubules decrease in number and transform into microvilli, decorating the secretory canaliculus, which expands in surface area and volume as acid is secreted into the canalicular space. The microvilli contain actin fibers that have moved from the cytoplasm, as shown by phallucidin staining. However, the ratio of globular (G) to filamentous (F) actin stays approximately the same, suggesting that eversion of the tubules is by a process of addition of actin at the growing tip and removal of actin from the base of the tubule.
These morphologic events in the parietal cell reflect stimulation-secretion coupling in the parietal cell; much of this chapter will be devoted to a discussion of the mechanisms underlying activation of acid secretion.
Receptors
The parietal cell appears to possess a variety of receptors for both stimulatory and inhibitory ligands. The majority of these have been defined functionally. Because most of the evidence for the action of modulating agents has been obtained in preparations that are heterogeneous with respect to cell type, some caution must be exercised in concluding that the effects are direct rather than indirect. There are few studies reported using specific receptor antibodies or in situ probes.
With the advent of viable isolated gastric gland preparations that can be stimulated or inhibited under superfusion, direct evidence has been obtained for the presence or absence of a specific receptor on the basolateral surface of the parietal cell. Because the gastric gland is composed of several cell layers, confocal microscopy provides a means of determining the presence or absence of a Ca2+ signal after addition of a ligand. An alternative approach in the case of isolated, dispersed cells is the use of single-cell video-imaging techniques when direct visualization can provide positive identification of parietal cells and assure that cells are sufficiently well separated to dilute any substances that might be released by potentially intervening cell types. The stimulatory receptors all belong to the seven transmembrane segment trimeric G protein type of receptor, as illustrated in the figure. These receptors function by releasing the bound trimeric G protein after ligand binding, resulting in a lower-affinity state of the receptor. The three subunits, a with GDP bound, β, and γ, are bound to the receptor or to a member of the RGS protein family.
After ligand binding, the α subunit releases GDP and binds GTP. The GTP-liganded trimer dissociates into α monomers and β-γ dimers. The α-GTP monomer then hydrolyzes GTP to GDP, enabling reassociation of the trimer and termination of the signal. These trimer G proteins are responsible for the effects of ligand binding to the receptor. The nature of the protein constituents of the trimeric G protein determines whether there is activation of adenylate cyclase or phospholipase C or inhibition of these enzymes. This determines whether there is an increase of cAMP-dependent protein kinase A activity, an elevation of intracellular Ca, or an inhibition of cell responses.
Most receptors are promiscuous in terms of which G proteins are bound. Antagonists of these receptors can be divided into pure antagonists that can only displace agonist or inverse agonists that are able to affect the conformation of the receptor in the absence of agonist. The latter class can therefore affect cell function in the absence of ligand.
The majority of receptors affecting acid secretion belong to the G7 (seven transmembrane segments) class of receptors (H2, muscarinic, CCK2, somatostatin, prostaglandin). The EGF/TGF-α receptor, which also inhibits acid secretion, is a tyrosine kinase receptor (single transmembrane segment with an intracellular ATP kinase domain).
In receptors such as the β-adrenergic receptor or others that bind biogenic amines, such as histamine, an aspartyl residue in the third transmembrane domain is thought to bind the positive charge of the ligand, and two serines in the fifth transmembrane domain (TM5) bind the aromatic residue. A phenylalanine residue in TM6 is also conserved in the biogenic amine-responsive G protein-coupled receptors. Antagonists may bind in different regions of the receptor, such as that close to the seventh transmembrane domain. Peptide agonists have a larger binding domain, which includes the second transmembrane segment. Nonpeptide antagonists at the peptide receptor bind at the top of TM5 and 6.
Histamine 2 receptor
The stimulatory action of histamine is mediated by the H2-subtype receptor. Histamine stimulation of acid secretion is inhibited competitively by selective H2 receptor antagonists but is not inhibited by agents acting at other receptors, indicating that histamine acts directly on the parietal cell. The cellular localization of H2 receptors using both in situ hybridization and autoradiographic localization of H2-receptor antagonist binding (125I-aminopotentidine) shows that the H2 receptor is located on the parietal cell. Also, elevation of intracellular Ca2+ can be observed directly in the parietal cell on addition of histamine to the superfusion medium.
H2 receptor antagonists inhibit gastrin stimulation fully but not that induced by carbachol (cholinomimetic), showing that the former, but not the latter, stimulant
depends on histamine release from the ECL cell. Histamine may be permissive for gastrin, and removal of this permissive action may also be responsible but to a lesser extent for the inhibition of gastrin stimulation by H2 antagonists. The histamine H2 receptor has been cloned and expressed, and the cDNA-derived amino acid sequence shows typical features of a G protein-coupled receptor with seven transmembrane segments. The quantitative correlation between stimulation of acid secretion, activation of adenylyl cyclase, and accumulation of cAMP, shown by a variety of H2 receptor agonists, provides strong evidence that the H2 receptor is coupled to activation of adenylate cyclase via phosphokinase A (PKA). The nature of the G trimeric proteins coupled to this receptor has not been determined. Addition of histamine also generates Ca2+ signals in the parietal cell. Perhaps both cAMP and Ca pathways must be activated for generation of the morphologic transformation essential for acid secretion.
depends on histamine release from the ECL cell. Histamine may be permissive for gastrin, and removal of this permissive action may also be responsible but to a lesser extent for the inhibition of gastrin stimulation by H2 antagonists. The histamine H2 receptor has been cloned and expressed, and the cDNA-derived amino acid sequence shows typical features of a G protein-coupled receptor with seven transmembrane segments. The quantitative correlation between stimulation of acid secretion, activation of adenylyl cyclase, and accumulation of cAMP, shown by a variety of H2 receptor agonists, provides strong evidence that the H2 receptor is coupled to activation of adenylate cyclase via phosphokinase A (PKA). The nature of the G trimeric proteins coupled to this receptor has not been determined. Addition of histamine also generates Ca2+ signals in the parietal cell. Perhaps both cAMP and Ca pathways must be activated for generation of the morphologic transformation essential for acid secretion.
Acetylcholine receptor
Extracts of belladonna have been used to treat dyspepsia since the Roman Empire, and its major component, atropine, was a primary medical treatment for peptic ulcer before development of the H2 receptor antagonists. Because atropine is a nonselective muscarinic antagonist, it is not possible, based solely on in vivo inhibition, to conclude that the parietal cell contains a cholinergic receptor. In vitro, particularly in the presence of H2 receptor antagonists, cholinergic stimulation of acid secretion is weak and often transient. That portion of the cholinergic stimulation that is not inhibited by H2 receptor antagonists is blocked by atropine. These results suggest that there is indeed direct action of ACh on the parietal cell in addition to the observed interaction with histamine. Inhibition of acid secretion by atropine indicates that ACh acts through a muscarinic-type cholinergic receptor but does not define the specific subtype of muscarinic receptor involved. To date, five muscarinic receptor subtypes
have been cloned and sequenced. All are G-protein coupled and exhibit structural similarities, although they couple to different intracellular signaling mechanisms. The M1, M3, and M5 receptors are activated via enhancement of intracellular Ca2+. The M2 and M4 receptors are inhibitory. Pharmacologic characterization of cholinergic stimulation of acid secretion in vitro indicates that the parietal cell contains an M3-subtype muscarinic receptor. Accordingly, carbachol stimulation of acid formation by isolated gastric glands is found to be inhibited by subtype-selective antagonists with a potency order of 4-DAMP followed by pirenzepine followed by AF-DX 116. An identical order of potency was found also for displacement of N-methyl scopolamine binding and elevation of intracellular calcium. This is the same order of potency exhibited by the cloned M3 receptor. The presence of an M3-subtype receptor on the parietal cell was further evidenced by detection of mRNA encoding the M3 receptor in parietal cells, whereas no evidence was found for expression of M1 or M2 subtypes. Thus, the direct action of ACh on the parietal cell appears to be mediated by an M3 muscarinic receptor. The M1 antagonist, pirenzepine, is an effective inhibitor of acid secretion. Presumably this occurs at a site in the peripheral regulatory pathway before convergence of stimuli on the parietal cell.
have been cloned and sequenced. All are G-protein coupled and exhibit structural similarities, although they couple to different intracellular signaling mechanisms. The M1, M3, and M5 receptors are activated via enhancement of intracellular Ca2+. The M2 and M4 receptors are inhibitory. Pharmacologic characterization of cholinergic stimulation of acid secretion in vitro indicates that the parietal cell contains an M3-subtype muscarinic receptor. Accordingly, carbachol stimulation of acid formation by isolated gastric glands is found to be inhibited by subtype-selective antagonists with a potency order of 4-DAMP followed by pirenzepine followed by AF-DX 116. An identical order of potency was found also for displacement of N-methyl scopolamine binding and elevation of intracellular calcium. This is the same order of potency exhibited by the cloned M3 receptor. The presence of an M3-subtype receptor on the parietal cell was further evidenced by detection of mRNA encoding the M3 receptor in parietal cells, whereas no evidence was found for expression of M1 or M2 subtypes. Thus, the direct action of ACh on the parietal cell appears to be mediated by an M3 muscarinic receptor. The M1 antagonist, pirenzepine, is an effective inhibitor of acid secretion. Presumably this occurs at a site in the peripheral regulatory pathway before convergence of stimuli on the parietal cell.
Gastrin receptor
The function of a receptor for gastrin on the parietal cell has been the subject of controversy. The central observation is the total absence or marginal stimulation of acid secretion by gastrin in the presence of H2 receptor antagonists. This has led most investigators to conclude that gastrin acts indirectly on the parietal cell by stimulation of histamine release from ECL cells. Favoring a direct action of gastrin on the parietal cell are reports indicating the presence of gastrin-binding sites on gastric mucosal membranes and enriched preparations of parietal cells. These binding sites are characterized as gastrin or CCK2-type sites in that they show equal affinity for gastrin and sulfated CCK. The presence of gastrin receptors on the parietal cell received further support by the cloning and expression of a CCK2-type receptor from a cDNA library derived from purified parietal cell mRNA.
Single-cell video imaging has provided direct evidence for a functional CCK2 receptor on the parietal cell. In particular, gastrin has been shown to produce an elevation of intracellular calcium in individual parietal cells, which is blocked by the CCK2 antagonist, L365,260. The pattern of intracellular calcium increase, consisting of a biphasic response, indicates a direct action of gastrin on the parietal cell, as opposed to mediation by histamine. Although parallel responses of acid secretion were observed, most of these experiments were not done in the presence of H2 receptor antagonists, and, therefore, one cannot rule out a permissive effect of H2 receptor activity on either the secretory or the cell calcium
response. Using confocal imaging, it was then shown that H2 receptor antagonists did not block the action of gastrin on ECL cells in rabbit or rat gastric glands but blocked the action of gastrin on parietal cell Ca2+. Further experiments showed that, whereas physiologic levels of gastrin stimulated Ca signaling in ECL cells and then parietal cells, the latter also blocked by H2 receptor antagonists, supraphysiologic levels of gastrin were able to elicit Ca signals in the parietal cells of the gastric gland. This suggests that there is a low-affinity gastrin response in the parietal cell, but this may not be to the presence of a CCK2 receptor.
response. Using confocal imaging, it was then shown that H2 receptor antagonists did not block the action of gastrin on ECL cells in rabbit or rat gastric glands but blocked the action of gastrin on parietal cell Ca2+. Further experiments showed that, whereas physiologic levels of gastrin stimulated Ca signaling in ECL cells and then parietal cells, the latter also blocked by H2 receptor antagonists, supraphysiologic levels of gastrin were able to elicit Ca signals in the parietal cells of the gastric gland. This suggests that there is a low-affinity gastrin response in the parietal cell, but this may not be to the presence of a CCK2 receptor.
Miscellaneous receptors
A variety of agents has been reported to stimulate or inhibit acid secretion by a direct action on the parietal cell. Many of these (e.g., cAMP derivatives, forskolin) do not act through cellular receptors, whereas others are suggested to reflect the presence of parietal cell receptors. Although no direct evidence for a parietal cell location exists, the ability of some substances to inhibit histamine stimulation of acid secretion argues for the presence of a parietal cell receptor, because the action of histamine is direct. Even in these cases, caution is necessary to interpret the inhibition as being direct rather than due to the release of another inhibitor. With this caveat in mind, the parietal cell appears to contain inhibitory receptors for somatostatin, prostaglandins (PG, EP3), and EGF. Each of these has been shown to inhibit histamine stimulation of acid formation by isolated cell preparations.
Somatostatin is thought to act through an inhibitory G protein to interfere with receptor-mediated second messenger production, explaining the inhibition of histamine stimulation. In the case of EGF, the inhibition appears to occur at a site beyond the H2 receptor, because EGF also inhibits dibutyryl cAMP stimulation of acid formation. The PG EP3 receptor has been shown to reduce cAMP levels, and, presumably, bonding to this receptor by relatively nonselective PGE2 compounds accounts for their inhibition of acid secretion.
The physiologic significance of inhibitory receptors on the parietal cell is not at all clear. A possible role for fundic or circulating somatostatin in controlling
gastric acidity is discussed above, but this function could be served fully by the action of somatostatin on histamine release from ECL cells. Because the putative direct action of somatostatin on the parietal cell is selective for histamine stimulation, dual inhibition of ECL and parietal cells would seem redundant. In the case of EGF and prostaglandins, it may be speculated that these inhibitors, which are known mediators of wound healing, act only during periods of mucosal injury to reduce acidity and allow more rapid healing. A model showing these receptors on the parietal cell is shown in the figure.
gastric acidity is discussed above, but this function could be served fully by the action of somatostatin on histamine release from ECL cells. Because the putative direct action of somatostatin on the parietal cell is selective for histamine stimulation, dual inhibition of ECL and parietal cells would seem redundant. In the case of EGF and prostaglandins, it may be speculated that these inhibitors, which are known mediators of wound healing, act only during periods of mucosal injury to reduce acidity and allow more rapid healing. A model showing these receptors on the parietal cell is shown in the figure.
Intracellular signals
Intracellular messengers
The gastric histamine H2 receptor is coupled to the formation of cAMP, whereas activation of the parietal cell M3 cholinergic receptor or the putative CCK2 receptor on the parietal cell leads to an elevation of intracellular calcium. The elevation of cAMP by the H2 receptor appears to result from a direct coupling, via a G protein, to adenylate cyclase; the elevation of [Ca]in proceeds by a more complex mechanism. The stimulation of a Gq-coupled receptor results in dissociation of the trimeric protein. Thus, there is activation of phospholipase C with breakdown of phosphatidylinositol 4,5-biphosphate (PIP2) to inositol 1,4,5-triphosphate (IP3) and diacylglycerol. The former results in the release of Ca from intracellular stores, perhaps aided by binding of the β-γ complex. The latter activates protein C kinase. Stimulants of C kinase, such as the phorbol esters, do not activate acid secretion in gastric glands. Activation of Ca entry accompanies release from intracellular stores, perhaps due to the interaction of the IP3 receptor with a membrane-located calcium channel.
Using fluorescent indicators with single-cell video imaging, the [Ca]in responses of individual parietal cells are typically found to be biphasic, consisting of an initial peak elevation that rapidly declines to a sustained steady-state elevation. This pattern of [Ca]in response is seen in many cell types and is interpreted to result from an initial release of calcium from intracellular storage sites followed by a sustained influx of calcium from the extracellular fluid via a receptor-operated Ca channel. Similar mechanisms appear to be involved in the agonist-induced elevation of [Ca]in of parietal cells. Accordingly,
removal of extracellular calcium or blockade of influx with La3+ abolishes the steady-state elevation but not the initial peak response.
removal of extracellular calcium or blockade of influx with La3+ abolishes the steady-state elevation but not the initial peak response.
In analogy with other cells, it is assumed that the intracellular pool of calcium is stored in specialized regions of the endoplasmic reticulum (ER), where it is released through calcium channels and resequestered by a calcium pump in the ER. Thapsigargin, an inhibitor of the ER calcium pump binding to the cytoplasmic loop between TM4 and TM5, raises [Ca]in in parietal cells, indicating that a typical calcium pump is involved in maintaining the intracellular pool. The release of [Ca]in appears to occur via a regulated calcium channel, two types of which have been identified. In muscle, the ER calcium channel binds ryanodine and is regulated by voltage and possibly by various ligands. In nonexcitable cells, presumably including the parietal cell, the predominant ER calcium channel appears to be one regulated by IP3.
The observations that cholinergic agonists elevate IP3 levels in gastric cells and that IP3
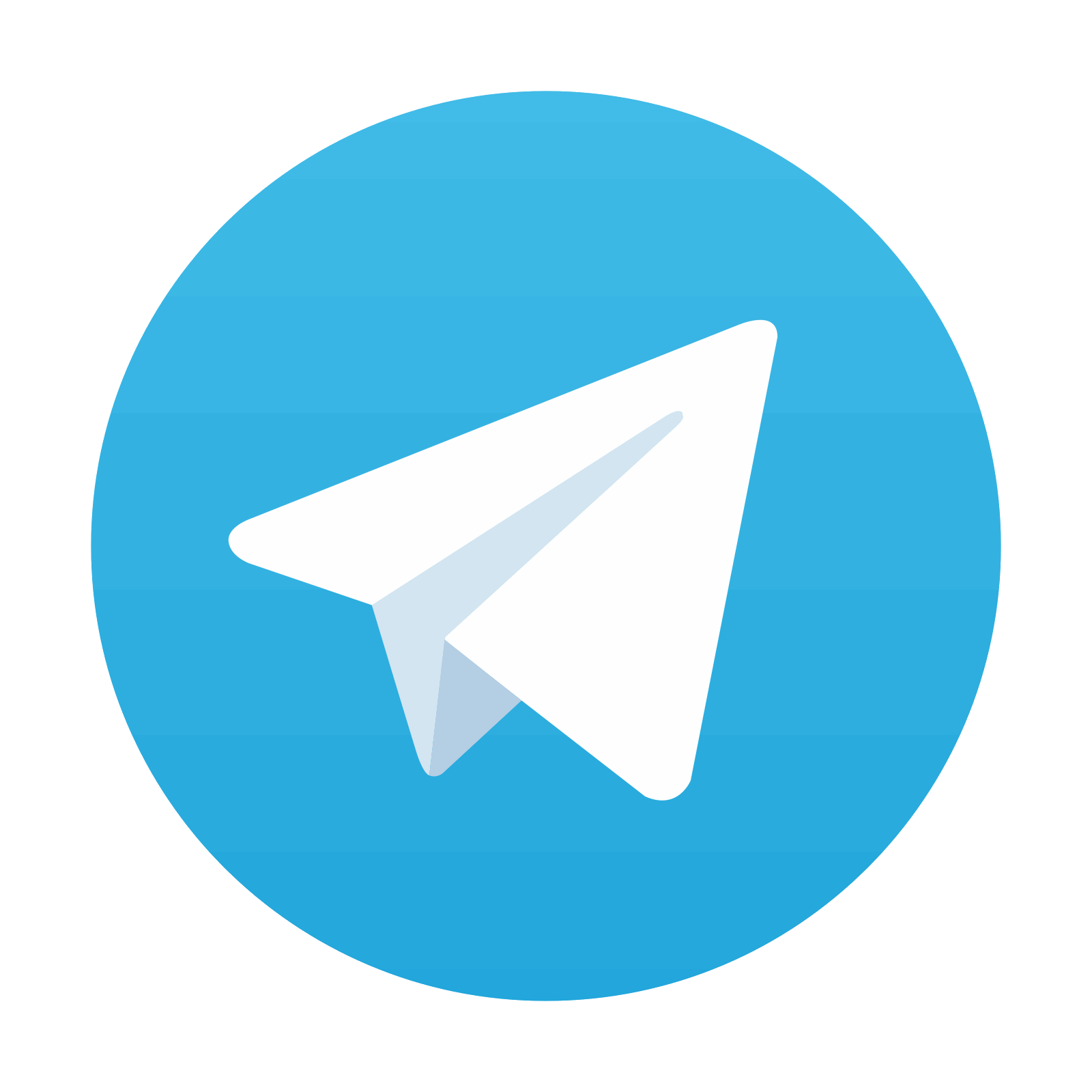
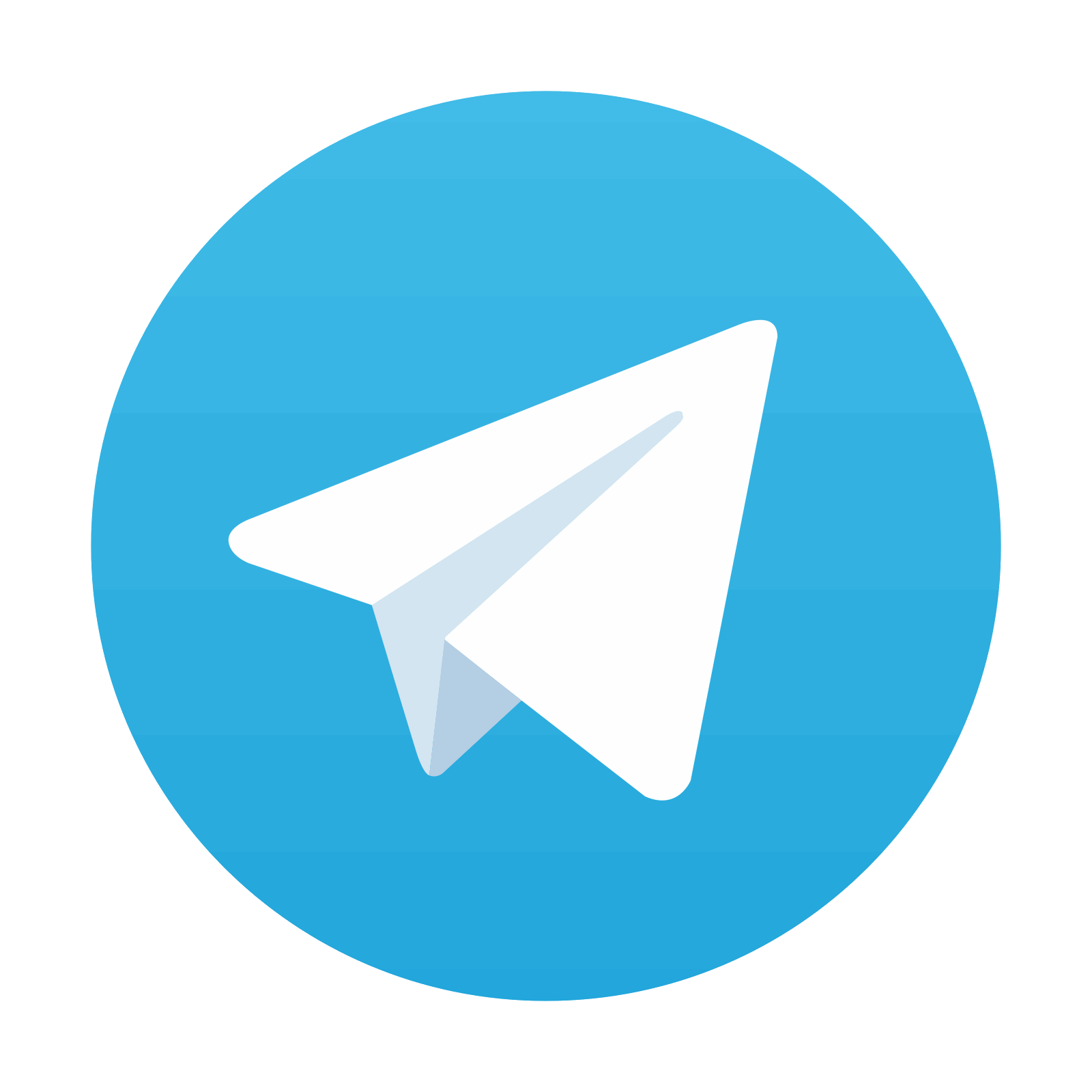
Stay updated, free articles. Join our Telegram channel

Full access? Get Clinical Tree
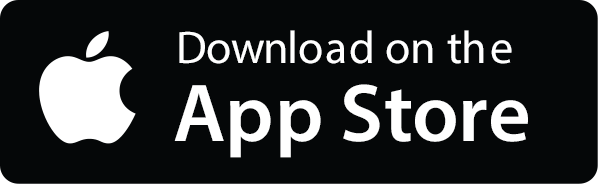
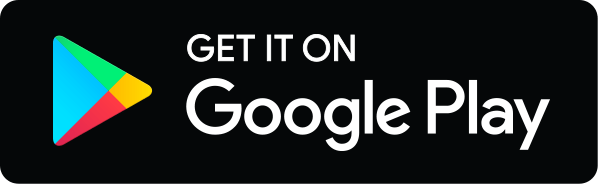
