A full anthology of the development of surgical robotics would require a complete textbook. Perhaps we could start the story with Leonardo da Vinci and his genius with a 16th century drawing of a mechanical knight ( Fig. 1.1 ). The Intuitive Surgical Company recognized the genius of Leonardo by naming their robotic systems in his honor, including the current and most well-known surgical robot, the “da Vinci.” For the purposes of this discussion, we will start our history in the 20th century. We direct readers who are interested in earlier developments to explore the scholarly manuscript by American Urologist Michael Moran, which documents a thorough history of robotic development from antiquity to the present 21st century surgical robotics systems.

The word robot was coined by the 20th century by Czech playwright Karel Cacek and used in his play named Rossums Universal Robots , written in 1920. The term robot was used by Cacek to describe unpleasant manual labor. In a further literary association, Isaac Asimov in his novel Runaround (1941) described the three laws of robots: “One: A robot may not injure a human being or, through inaction, allow a human being to come to harm. Two: A robot must obey the orders given it by human beings except where such orders would conflict with the First Law. Three: A robot must protect its own existence as long as such protection does not conflict with the First or Second Laws.” 1a Wonderfully, the conception of the robot has devolved from art, literature, and science.
More recently in 1979, The Robot Institute of America defines a robot as a reprogrammable, multifunctional device, designated to manipulate and/or transport material through variable program motions for the performance of a variety of tasks. Various classification systems exist to categorize robots according to their functionality.
The development of a functioning surgical robot was made possible by the development of industrial robotics. Goertz invented the first real working industrial robot in 1949. He described and developed an operator-robot system, which was used to handle radioactive substances. There were further major developments and improvements in industrial robot design over the next 40 years. Use of the industrial robot has permeated manufacturing processes in many industries, particularly the automotive industry.
Success has many parents, and the development of the contemporary surgical system of robotics is no exception. It seems reasonable to suggest that the development of safe open surgery occurred after introduction of antisepsis, anesthesia, and transfusion to surgery from the late 19th to the early 20th century. Leonard Murphy published his volume The History of Urology in 1972. He includes a history he translated from the French written by Desnos, describing urologic surgery from ancient times to the 19th century. In reading this marvelous book, it becomes clear to the reader that there is nothing new under the sun. Many of the procedures performed after safe surgery was introduced had been tried previously by our surgical forebears in the 19th and early 20th centuries but failed because of lack of intravenous transfusion, antisepsis, antibiotics, and appropriate anesthesia. The practice of surgery via open technique was maintained exclusively until the 1940s, when the practices of endoscopic surgery in urology (transurethral resection of the prostate [TURP]) and laparoscopic minimal access surgery primarily in gynecology were introduced. Celioscopy described by internists for peritoneoscopy or laparoscopy described by gynecologists was first introduced in the 1940s. Laparoscopy was first used for the visual diagnosis of pathologic conditions of female reproductive organs. Contributions to the improvements in gynecologic laparoscopy were reported by Cohen in the late 1950s which popularized gynecologic laparoscopy. Laparoscopic development paved the way for robotic adaptation, with improvement in lenses, light sources, and the ability to raise and maintain intra-abdominal pressure with a pneumoperitoneum by insufflation of the abdomen with carbon dioxide. The ability to achieve hemostasis using diathermy was also vastly improved.
Early surgical robotics
My first acquaintance with robotics in surgery occurred when I read the description by London urologist, John Wickham, of a new surgical machine (Probot) that could perform TURP. TURP is a difficult operation both to teach and to learn. To enable this to be performed hands-free using a robot was an interesting concept. The system made by Wickham and his engineers combined the PUMA robot with a Wickham Endoscope Liquidizer and Aspirator to perform a hands-free robotic transurethral resection for benign prostatic enlargement ( Fig. 1.2 ). The tissue to be removed was mapped by using transrectal ultrasound coordinates. Problems with hemostasis precluded its further success. Robotic technology using a water jet for cutting with ultrasound-mapped coordinates (Aquablation) has been recently described.

Laparoscopic surgery was embraced enthusiastically in the 1990s. Previously this surgery was mainly in the remit of the gynecologist. Laparoscopic techniques were applied in general surgery with cholecystectomy and appendicectomy and in urology with pelvic lymph node dissection. In 1991, Clayman described a single case of successful laparoscopic nephrectomy. This was ground breaking, enabling further expansion of the urologic laparoscopic surgery repertoire into more major procedures than simple pelvic lymph node dissection for staging in bladder and prostate cancer.
A key step toward using robotics in laparoscopic surgery was Computer Motion’s invention of the Automated Endoscopic System for Optimal Positioning (AESOP). AESOP controlled laparoscopic camera positioning rather than requiring a human bedside assistant for this task. Its first model was controlled using foot pedals, but this was later upgraded to a voice control system.
Birth of the contemporary surgical robot
Rival entities, Intuitive Surgical and Computer Motion, raced to develop the contemporary surgical robot. Intuitive finally won when the first robotic operation (cholecystectomy) was performed with their robot “Mona” in 1997 in Belgium.
Much of the driving force behind the development of robotic surgery came from the US Army’s desire to create a technology that would allow surgery to be performed on soldiers in the battlefield, by surgeons remote from the battlefield using telerobotics. The US Defense Advanced Research Projects Agency (DARPA) provided grants to stimulate development of robotic surgery. Similarly, a role for telemanipulation was also imagined in space, and NASA’s Ames Research Center provided funding for proposals toward projects for remote surgical intervention on astronauts.
Intuitive Surgical Company was established in 1995, after it licensed patents from Stanford Research Institute (SRI) (Palo Alto, CA), International Business Machines, and the Massachusetts Institute of Technology. Frederick Moll and his colleagues wanted to build a system of telerobotic surgery, which included three key elements:
- 1.
An operator-robot, software driven system that provided intuitive control of a suite of seven-degree-of-freedom laparoscopic instruments.
- 2.
Stereoscopic vision system displayed in an immersive format.
- 3.
An elaborate system of sensors to eliminate the possibility of erroneous movement and maximize safety during operation.
Intuitive Surgical, building on the original telemanipulation work by Phil Green of SRI, launched their first prototype, Lenny (after the young Leonardo da Vinci). Lenny, used only in animal trials (1996), comprised three separate robotic arms to be attached to the operating table: two working arms and one camera arm. Shortly thereafter came Mona (after Mona Lisa ), Intuitive’s second-generation model that enabled exchange of instruments while maintaining sterility of the surgical field ( Figs. 1.3 and 1.4 ). At a similar time, competitor Computer Motion developed the ZEUS system, which incorporated AESOP’s robotic arm with two arms for laparoscopic instrumentation. ZEUS was used in 1998 for uterine tubal reanastomosis and cardiac bypass surgery.


The Intuitive Surgical robot was further improved and enhanced, and I was fortunate to see it in 1999 as a prototype at the American Urological Association meeting. The first da Vinci which gained US Food and Drug Administration (FDA) approval for use in surgery (in 2000) was a three-part system with a patient cart, surgeon console, and image system. All three robotic arms emanated from the patient cart rather than mounted on the operating table as the previous systems had. The viewing and operating console delivered a three-dimensional (3D) view provided by a novel binocular telescope. Containing two video camera control boxes with two light sources for the cameras, a synchronizer keeps the video frames of the video cameras in phase. The vision system afforded by the original da Vinci was truly outstanding when compared with the two-dimensional view of standard laparoscopic cameras . Another key innovation that led to the success of da Vinci was the design of EndoWrist surgical instruments that afforded seven degrees of freedom, enhancing dexterity and operative precision.
After much legal friction, Computer Motion and Intuitive Surgical merged (2003). Soon afterward, ZEUS was discontinued in favor of da Vinci.
There have been multiple generations of da Vinci systems engineered by Intuitive along the journey to the present Xi model. Vision was further improved with a high-definition 3D camera in the 2006 da Vinci S model, and the introduction of a dual console greatly enhanced training. More recently, Intuitive has also built and marketed a single-port system with flexible instruments and a rigid telescope inserted through one central port. Thus far, this new system has failed to generate the enthusiasm required for widespread use.
After many years of Intuitive Surgical dominating the field of robotics, we are beginning to see an increasing number of new vendors appearing. Cambridge Medical Robotics (CMR) has developed an open console program where the surgeon looks at a flat screen television and uses 3D glasses for depth perception. The system designed by the Cambridge group has no foot controls and is hand controlled only. This allows the surgeon to stand for the surgery. The open system may also allow easier communication between the operating room team at the bedside and the console surgeon. Additional vendors are due to launch their own platforms in the very near future, discussed in brief later in this chapter, as well as in further detail in Chapter 2 .
A frequent criticism of the technology has been the lack of haptic feedback provided to the surgeon in the console. Interestingly, unlike the currently available systems, SRI’s research prototype included haptic feedback. Unfortunately, this aspect was rudimentary, taking into account only force feedback (pressure), not other important sensations such as vibration, friction, and stress. Ultimately the high-definition images delivered by later robots provided visual cues that replaced tactile feedback, and the lack of traditional haptics did not pose a significant barrier to adoption of an excellent surgical dissection technique. Mastery of the learning curve includes developing muscle memory for force required for different instrument use in performance of tasks such as the force applied in suturing to avoid suture breakage.
Adoption of robotic surgery
After the first surgery was performed robotically in 1997 in Belgium, how did robotic surgery become a viable alternative to laparoscopic and open surgery? Although the laparoscopic method had become popular through the 1990s in general surgery, gynecology, and urology, performance of many laparoscopic surgical procedures remained technically challenging. Robotic introduction of 3D vision, 10 times magnified surgical field, and intuitive wristed hand movements with increased dexterity and elimination of tremor heralded the demise of the laparoscopic method. Many of even the most ardent proponents of laparoscopic surgery have modified their enthusiasm and taken up robotics.
Menon, with great foresight, after observing the cardiothoracic surgeons in Detroit using an early da Vinci model, believed this technology could be applied to deep pelvic surgery. Claude Abbou, a very skillful French laparoscopic surgeon, was also instrumental in early robotic prostate surgery, publishing his experience of a case of radical prostatectomy he performed robotically in 2000. Radical prostatectomy is one of the more difficult operations for a urologist to learn. It requires extirpative and reconstructive skill for the surgeon. The siting of the prostate deep in the pelvis anterior to the rectum and under the pubic symphysis makes access and reconstruction very difficult, thus lending itself well to robotic application thanks to the benefits of the seven degrees of freedom with the da Vinci instruments, as well as improved visualization of the relevant anatomy. The operation of open retropubic prostatectomy was made safe only in the 1980s by Walsh, who was first to describe the essential step of suture ligation of the dorsal vein of the penis. Before that, the operation was extremely hazardous for the patient due to major blood loss from this vein located under the pubic arch. He also discovered and reported the neuroanatomy involved in male erectile function and published this in 1982. Until then, surgeons simply cut the neurovascular bundles, destroying erectile function in 100% of surgeries. Contemporary key metrics used to assess technical expertise in this operation are related to preservation of these neurovascular bundles for erectile function, maintaining urinary continence by superbly visualized dissection of the external sphincter, while achieving negative cancer margins. Menon described how to perform the operation robotically using Walsh’s method, preserving the erectile nerve bundles and urinary sphincter. He was able to demonstrate equivalence of the robotic method to the open method in what remains a technically challenging operation. He appreciated that using a robot with its minimally invasive approach, the surgeon could visualize the key anatomic structures in three dimensions and drive the robot to places where the human hand could not easily reach. A clear example of this was the ability to perform a running sutured watertight anastomosis robotically. In the open method surgery, the vesicourethral anastomosis was created by use of four to six interrupted sutures put in blindly by the surgeon. The stricture or bladder neck stenosis rate of the interrupted suture in the open method was historically as high as 17% in some series. Van Velthoven 20 described the running watertight robotic suturing method, the stricture rate reduced to less than 1%. By 2008 surgeons could report robotic-assisted radical prostatectomy outcomes with equivalence in oncologic and quality-of-life parameters. The operation became one that could be performed safely robotically as day case compared with what was previously a 3- to 5-day hospital stay for open surgery. A very positive by-product of introduction of robotic radical retropubic prostatectomy was that many more patients who had previously been denied surgery for their cancer could be offered radical prostatectomy, due to the reduced morbidity associated with the procedure. The minimally invasive robotic approach is associated with significantly less blood loss compared with a mean 1300-mL loss in open prostatectomy. This has allowed urologists to offer surgery to men who warranted radical therapy but were not suited to external beam radiation and previously would not have been eligible for open surgery due to body habitus or comorbidities such as prior mesh hernia repair or previous extensive pelvic surgery.
This new benchmark for radical prostatectomy heralded introduction of the robotic method of surgery in urology. We now have seen similar revolutions in cardiothoracic surgery, gynecology, general and colorectal surgery, and ear, nose, and throat surgery. The robotic approach is also used in some centers of excellence to perform pediatric surgery. The widespread dissemination of robotic method into so many surgical specialties formed the basis for the design of this textbook to be a multidisciplinary one, not exclusively urologic. This book covers both the foundations of robotic surgery for all surgeons and then embraces robotic surgery for the individual surgical craft groups.
Barriers to widespread adoption of robotic surgery
If robotic surgical technology provides an attractive alternative to open and laparoscopic surgery, why has there not been a wider adoption of robotics internationally? The two key obstacles are cost and education.
However, it would be remiss not to also describe the controversy associated with the introduction of this new technology. Indeed, part of the marketing pitch from hospitals during the technology arms race in the early 2000s, when robotic surgery was introduced, was encouraging a belief in patients that if it was robotic, it must be better. The concept of a robot performing your surgery was certainly beguiling. Hospitals with robots were the haves, and those without robots were the have-nots. The vendors, hospitals, and surgeons did not help the cause of introduction of robotic surgery by making extravagant claims about the safety, efficacy, and superiority of robotic method over open surgery and laparoscopy in order to attract patients. One study on outcomes from radical prostatectomy reported that 97% of men were potent following robotic radical prostatectomy. Considering the cohort’s age was around 60 years and men at 60 have a 40% likelihood of erectile dysfunction, it suggested that robotic prostatectomy was actually improving erectile function.
Early scientific reports are few and far between, in part due to the disbelief within the medical and scientific fraternity that extended to major journals. Dr. Himpens, who performed the first robotic cholecystectomy in Belgium in 1997, was refused publication in The New England Journal of Medicine and The Lancet and had to resort to documenting this landmark event via a letter to the editor in Surgical Endoscopy. In contrast, many emotive editorials have been published, with titles such as “Gizmo Idolatry and the Robot.” In fact, the first urologic randomized trial in robotics comparing the open to robotic approach in a 200-patient cohort was reported only as recently as 2016 by Yaxley and his group in Brisbane. The study showed equivalence in quality of life and early cancer outcomes between both robotic and open radical prostatectomy.
Cost
The high initial capital outlay, as well as ongoing instrument and service costs, have deterred hospitals from introducing robotic systems. Although there are more than 3000 da Vinci machines operating around the world, most of these are placed in developed countries. Within these developed countries, robotic surgery is mainly provided in the private sector to insured patients. The capital cost of the da Vinci robot in 2003 in Australia was $3.2 million dollars. Instruments were engineered to have a life of 10 uses, and after this, the instrument software precluded further use. The cost of instruments in 2003 per case was $4200. The cost of the latest Xi da Vinci machine in Australia in 2021 is $4 million with equivalent instrumentation costs, and the 10 use per instrument maximum persists. Therefore surgeries, which are done cost effectively laparoscopically or open, such as appendicectomy, hernia repair, and cholecystectomy, are prohibitively expensive if performed using a robot. This has meant the introduction of robotic surgery to many of the very common abdominal and pelvic surgeries has not occurred. Many surgeons trained in open and laparoscopic surgery still decry the use of robots as no better for the patient than traditional methods and far more expensive. In most of these more minor surgeries, they are probably correct. However, if cost equivalence between open, laparoscopic, and robotic methods occurs, then the minimally invasive, intuitive approach of the robotics becomes more feasible.
Education
The lack of a formal, validated proficiency-based curriculum for robotic surgery education has hampered surgeon uptake. Many of our surgical educators were trained in the era of open and laparoscopic surgery. That has meant that our trainers or surgical educators have had to learn to operate the robot themselves while mentoring trainees on the dual console system. Beane reported the problem of this inadequate learning method. He observed that trainers who are insecure or underconfident in their robotic surgical ability found it difficult to hand over the controls of the robot to their mentored trainees. The concept of uneven training experiences he described as “shadow learning.” By this he meant that learning robotic surgery for trainees is often opportunistic, infrequent, and haphazard.
It has been often the case in the digital age that introduction of fancy new technology outstrips the ingenuity and ability of those who are destined to use it. Nowhere was this more obvious than in the introduction of telerobotic surgery. The manufacturers of the robot developed a brilliant new technology. In the main the manufacturers were engineers not surgeons. Intuitive has provided an online “buttonology” guide and service manual but was not equipped to teach the individual surgeon how to operate this exciting new instrument. An often used analogy is for the Boeing aircraft company to manufacture an airplane, deliver it to the pilot, and suggest they go fly it without a full simulation exercise program. The airlines themselves provide the education, and Boeing makes the excellent airplanes. Until now, there is no recognized or standardized curriculum endorsed by appropriate authorities such as surgical boards or colleges for comprehensive linear proficiency-based robotic surgical education. It has been left to the vendor and some individual robotic surgeons to provide education. In many instances, this has been inadequate. In some situations in Australia the requirement has been to watch an online instructional video. The surgeon then will perform cadaver or live animal surgery usually as a single event and proceed to be mentored in early case experience (one to three cases) by someone with more experience, but not much more, in robotic surgery. There is no standardized credentialing with regard to proficiency. A recent publication from the United Kingdom estimated that the learning curve for the performance of robotic prostatectomy was 300 cases. Clearly, the early proponents of robotic surgery were rapid adapters and those who were more inclined to undertake risky tasks. For the majority, it does take a long period to educate a naïve robotic trainee to proficiency and safety in the performance of their craft group operations. However, not all surgeons have the hand-eye coordination to adapt to the use of robotic surgery. This has been well shown in many of the simulation training studies; up to 6% could not achieve proficiency required in hand-eye coordination and visual spatial aptitude to be able to drive the robot satisfactorily. , It is also reported that surgical skill degradation occurred after 4 weeks of no activity. Many surgeons who have adopted robotics are performing very few surgeries, and thus each operation represents a relearning of the coordination and technical proficiency necessary for that task.
There is a dramatic underscoring of surgical failure to heed lessons of aviation education and instruction. After the introduction of simulation to aviation training, the accident rate in airlines dropped dramatically. Since 1999 the overall aviation accident rate is less than 1%, a reduction of 95%. Even the aviation industry has not learned these lessons adequately. When Boeing sold the 737 Max to airlines in 2018, it failed to provide an upgraded simulation program to train the pilots. This resulted in 600 passengers perishing in two airline crashes because of pilot ignorance of the software and handling capacity of this aircraft. The sequelae from this oversight have dealt a devastating blow to the reputation and financial health of the Boeing aircraft company.
The future
Between 2000 and 2021 we have witnessed the emergence of a surgical robot which has transformed many surgical disciplines. We are by no means at the end of this journey. Barriers to adoption, as discussed in this chapter, include cost and an absence of a formal education curriculum. The future of robotics will need to tackle both of these issues to continue to thrive.
Disruption by emergence of new vendors
Goldman Sachs has predicted that from a 2019 base of $5.3 billion, the worldwide robotics market mainly occupied by the Intuitive Surgical Company will increase incrementally with the emergence of many new competitors. They predict that the market will be worth $14.1 billion in 2025 and $24 billion in 2030. The existing market will likely be divided into segments occupied by the new vendors and Intuitive. It is predicted that Intuitive will retain around 50% of market share through this period.
The robotic surgery landscape will be disrupted significantly by the emergence of new vendors and systems, hopefully leading to a more competitive lower-cost market. The first of these is the CMR system launched in 2021. This system differs from the existing da Vinci construction by being open console, the surgeon using 3D glasses to look at a flat screen, and modular robotic arms and hand controls only, with no foot control. Similarly, Medtronic in 2022 will market the Hugo robot which will provide an open platform with modular instrument arms similar to the Cambridge technology, while including both hand and foot controls. These companies hope that this new open console design will lead to improved operating room communication over the da Vinci. When operating the da Vinci, the surgeon has their head in the da Vinci console, and clear closed loop communication with the operating team can be problematic. In 2022, it is likely that the Japanese company Kawasaki will launch a new surgical robot named Hinotori. This machine looks similar to the da Vinci, where the surgeon operates remotely with their head in a “cockpit.”
Interestingly, Wickham (of the revolutionary Probot) commented that moving from automated robots to operator-robot systems (e.g., da Vinci) has been a retrograde step. A return to integration of active or semiactive surgical robots is something we may see in the future. Johnson & Johnson has announced they are building a disruptor robot through their company Verb Surgical that may have significant artificial intelligence overlay, facilitating some degree of autonomy for the robot in certain parts of surgical operations. It is not difficult to conceive of the robot being programmed to be capable of insertion of the laparoscopic ports and have an ability to suture wounds and maybe fashion anastomoses. However, it is unlikely we will have operating rooms without surgeons in the near future. That said, already there are driverless vehicles in industry and drones, which have been weaponized and piloted remotely. Perhaps closer in sight is the possibility of augmenting robotic surgery precision by overlaying images from magnetic resonance imaging (MRI), computed tomography (CT), or even detail on a microscopic level.
High-fidelity models: Repetitive practice to proficiency
A new approach to robotic surgical training shifting from the Halsted apprenticeship model to the digital era is pressing. Surgeons’ ability to have their hand movements digitized by a robot and reproduced inside the body cavity forces a rethink of how we educate surgeons in the digital age. Training a surgeon at the bedside potentially puts patients at greater risk of an adverse outcome, as during the early learning curve operative time increases as does the rate of complications. The trainee in 2021 undertakes their learning curve experience on live patients in the operating room until proficiency occurs. Surgical proficiency can now be measured adequately away from the operating room using simulation scoring and virtual reality. As previously stated, the aviation education method which uses simulation is one which can be readily adopted into surgical education. There are now very sophisticated simulators manufactured by companies such as Mimic PIC Portable Sim and 3D Systems which allow repetitive training of surgical tasks which can be measured to proficiency. Use of simulators provides a linear proficiency-based learning system, allowing those students who adapt more rapidly to progress in their surgical training more quickly. It takes 4 to 7 years to train a surgeon in Australia once the surgeon enters a training program. Some would consider this too long. At the moment, a one-size-fits-all training program exists. However, a dedicated robotic surgical curriculum using simulation may shorten the training time for suitable surgeons.
It is no longer feasible ethically and financially to use live animals and cadavers as single episode surgical training experiences. Training can be augmented with use of 3D printed high-fidelity polyvinyl alcohol human organ models generated from CT and MRI images , (described by Ghazi later in this book, Chapter 8 ) that obviate the need for animal and cadaver specimens. These much-cheaper synthetic models allow repetitive practice away from the operating room to achieve surgical proficiency. Away from the operating room, surgeons can learn in a much less stressful environment where there is no risk of an adverse outcome to a patient. All surgical trainees have experienced the anxiety and pressure to perform live surgery while desperately trying to avoid a complication. The operating room environment is high pressured and probably not best suited for a maximally beneficial education experience. Surgeon trainees learn much better in a nonthreatening environment. Any surgeon trained in the 20 th century will be empathetic to these sentiments. Once the trainee has satisfactorily mastered surgery on synthetic organ models to proficiency, they can then progress to mentorship in the operating room.
Further limiting education opportunities is the absence of sufficient numbers of skilled robotic surgical trainers. From the sporting analogy, many great athletes do not make great coaches. Similarly, many great surgeons do not make great surgical trainers. We have yet to fully recognize and recruit those expert and super expert robotic trainers most suited to educate young surgeons away from the operating room. We need to identify those surgeons to lead robotic laboratory and operating room training, ideally in a dual console.
New adaptations and applications
We have already seen exponential growth in the breadth of application of robotic surgery in the past 20 years, as evidenced by the extent of procedures outlined in this textbook. We anticipate that with new robotic systems becoming available, this trend will continue within fields where robotic surgery is familiar, and rapidly spill over into new surgical applications. It is also likely that medical disciplines including gastroenterology and pulmonology will become robotic procedural disciplines similar to cardiology, where a robot is being used to insert cardiac stents with the cardiologist as the driver. Large flexible endoscopes have been developed to be used in conjunction with laparoscopic and robotic instruments to perform esophageal, gastric, colonic, and thoracic surgery. We live in a brave new surgical world.
References
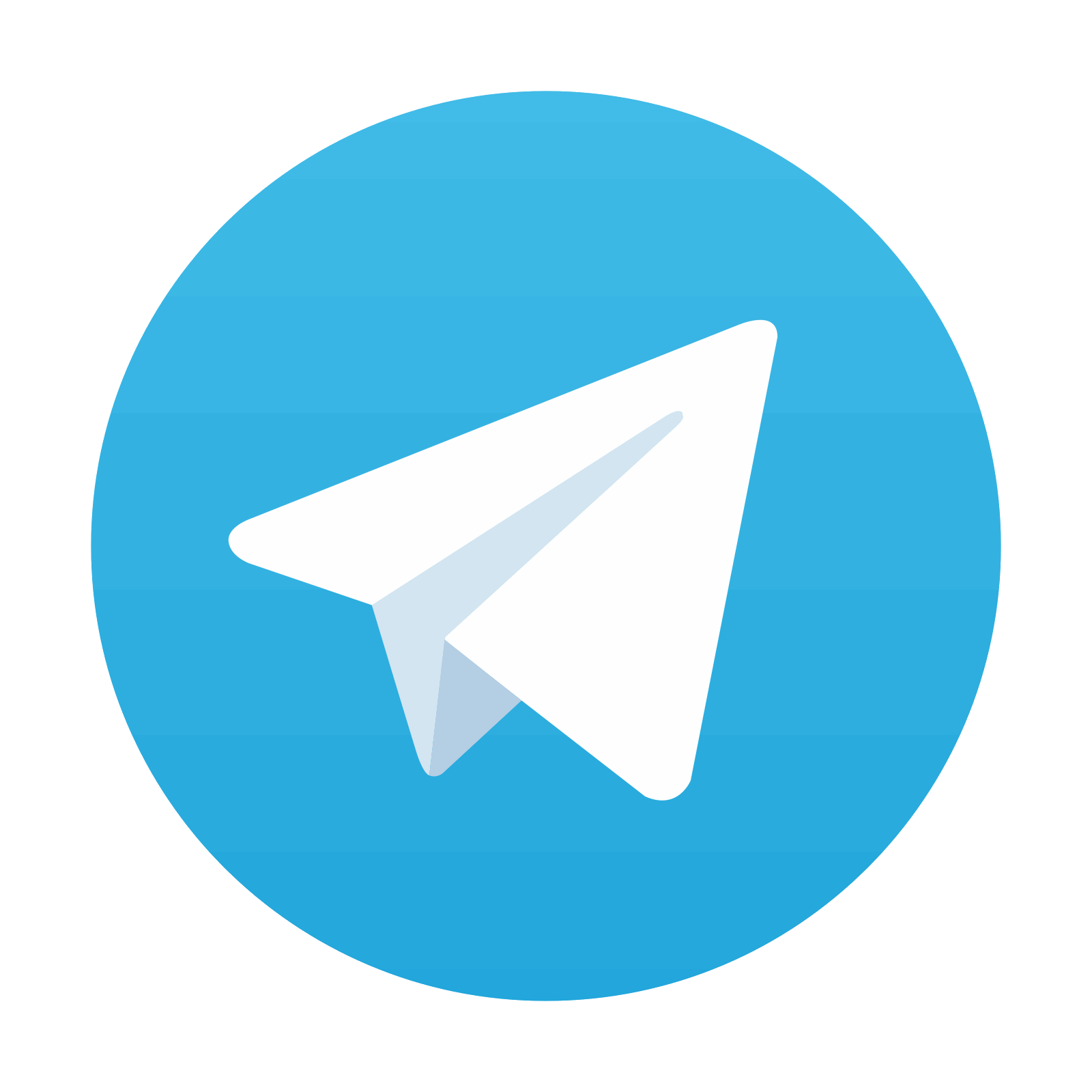
Stay updated, free articles. Join our Telegram channel

Full access? Get Clinical Tree
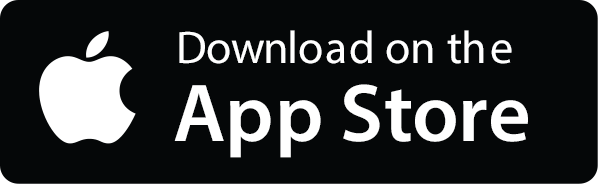
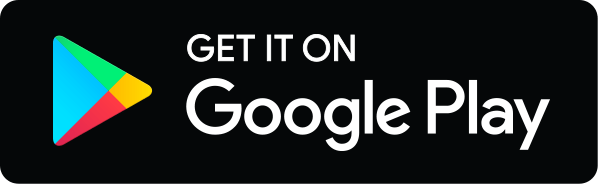
