Structural Collapse, Extrication, Crush Syndrome, and Rhabdomyolysis
Adam H. Miller
In the new era since 9/11, our world faces not only the constant threats of natural disasters but also man-made tragedies. Health care providers, and the study of disaster medicine, must seek to understand these events and plan for their medical and surgical consequences. By coupling predisaster preparedness with the best available techniques in search, rescue, and health care, the best results can be achieved. This chapter looks at the importance of building structure design. It then continues with the medical consequences of these structural flaws when they are faced with natural and man-made disasters.
A review of the disaster literature suggests that some of the morbidity and mortality of past world events could have been prevented, particularly if structures were reinforced and medical intervention had been initiated more expeditiously. Whether it is from an earthquake or a bomb blast, loss of life may result either from the direct effect of trauma or the metabolic consequences of crushing weight upon the body. As we shall see, this excessive weight damages muscle and other tissues and results in various complications.
The development of firm guidelines for building construction and the improvement in the organization of urban search and rescue are the essential types of prevention required to ensure improved outcomes. Key medical features include renal failure, electrolyte disturbances, and the potential for loss of one or more limbs. Acute renal failure (ARF), a deadly consequence of rhabdomyolysis, is mostly preventable by timely rehydration therapy. Hyperkalemia and infection are the commonest causes of death in victims who survive the direct effect of trauma (1). In addition, fasciotomy can be limb saving if it is done in the early hours. Each of these features will be described.
BUILDING STRUCTURE AND THE RISK OF CRUSH INJURIES
Many papers have been written pertaining to natural and man-made disasters, including descriptions of earthquakes, bombings, gas explosions, and derailments and collisions of trains, along with other types of trauma-related events (2,3,4). As building structures age, the risk of their collapse and injury to human life increases. This chapter explores several past events, which may provide insight that may be applicable to modern events.
The Turkish earthquake literature describes the primary mechanism of injury and death in earthquakes as attributable to the physical trauma from collapse of human-made structures (2). This literature points out the vulnerability of certain types of building structures. The most likely to collapse were the midrise, unreinforced masonry buildings (MUMBs) and the one-story adobe structures with soft ground floor construction. These buildings were typically designed with a ground floor intended for commercial use, incorporating large shop-front windows. The higher floors were used as residential apartments.
In the Turkish earthquake of March 13, 1992, the epicenter occurred 5 km (3 miles) east of the city of Erzincan, at the intersection of the North and Northeast Anatolian fault lines in response to movement of the Arabian, Anatolian, and Northeast Anatolian tectonic plates. Of the 526 mortalities, 92% were attributed to the MUMB collapses characterized by a ground floor collapse pattern. Being on the first floor was an important predictor of death. In addition, the Turkish literature looked at the disaster response effort, discussing a detailed account of local response, which included the city administration, the national response, and the substantial international participation, especially crews from neighboring cities and countries. There was typically a delay of more than 24 hours in the arrival of appropriate search and rescue personnel and equipment. This prolonged response contributed to a significant number of deaths, which otherwise may have been preventable with immediate rescue and medical response.
The literature on the Hanshin-Awaji Japanese earthquake of 1995 describes 14 patients who sustained crush injuries (3). They had been buried under collapsed houses for an average
of 6.7 ± 5.7 (SD) hours (5,6,7,8). Victims who arrived at major treatment centers later (2 of the 6 victims), were more likely to have developed renal failure than those who were brought earlier. The major clinical problems encountered in the treatment of these patients included deciding whether to use the fluid resuscitation regimen to prevent ARF and determining whether there were clear indications for fasciotomy in an attempt to prevent the loss of limbs.
of 6.7 ± 5.7 (SD) hours (5,6,7,8). Victims who arrived at major treatment centers later (2 of the 6 victims), were more likely to have developed renal failure than those who were brought earlier. The major clinical problems encountered in the treatment of these patients included deciding whether to use the fluid resuscitation regimen to prevent ARF and determining whether there were clear indications for fasciotomy in an attempt to prevent the loss of limbs.
CRUSH SYNDROME
A syndrome associated with myoglobinuria was first described by Meyer-Betz in 1910 (28). However, it was recorded earlier in association with crush injuries after the earthquake in Messina, Italy, in 1909, and again later by Bywaters and Beall after observations of crush victims during World I (29,30). In the era since the London Blitz in World War II, the term “crush syndrome” has been applied to the ischemia-induced syndrome of myonecrosis, myoglobinuria, and renal failure.
In 1941, Bywaters described a syndrome of “being trapped under the rubble for hours.” After release, patients developed “swelling and later muscle weakness in the injured limb, vasoconstriction and hypotension after several hours, decreased urine output, changes in urine sediment, hemoconcentration, hyperkalemia, acidosis, and persistence of loss of blood supply and gangrene in the affected limb even after correction of blood pressure” (9).
Crush syndrome is the general manifestation of a crush injury involving one or more limbs or abdomen under extreme pressure for a few hours. The prolonged pressure to the limb or abdomen leads to a breakdown of the integrity of the muscle cell membrane and derangement of cellular transport and occurs when individuals are trapped under fallen debris during earthquakes, bombings, and other disasters (1,10). This in turn may cause a syndrome of rhabdomyolysis and ARF, hyperkalemia, disseminated intravascular coagulation (DIC), cardiomyopathy, and other systemic manifestations, which may be fatal if not treated promptly and properly (11).
In crush syndrome, the damaged muscle cells lose the capacity to pump water out of cells by active transport, which is necessary to maintain their internal environment. When a myocyte exhausts adenosine triphosphate (ATP), its normal homeostatic, synthetic, and reparative functions deteriorate (12,13). The result is loss of sarcolemmal and cellular membrane integrity and leakage of intracellular contents. Prolonged pressure on a muscle group can produce intracompartmental pressures reaching up to 240 mm Hg. This interrupts the normal blood supply, cutting off essential nutrients for oxidative phosphorylation. In turn, too little ATP, which is essential for cells to live, is produced (14). Cell rupture releases potassium and muscle pigment, and then the body’s water begins to move into this area of injury, known as “third spacing.” Therefore, hypovolemia is a major problem. Along with the deposition of myoglobin from the injured muscles, the hypovolemia causes acute tubular necrosis (ATN) and acute renal failure. This can be mitigated by early administration of intravenous fluids. In addition, patients may require acute dialysis therapy if ARF is severe. In fact, if kidney failure is present, death may result within a week if dialysis is not instituted (9).
Since the original description by Bywaters in 1941, crush injury has been examined extensively; in general, the clinical course and subsequent prognosis are significantly altered by multiple factors such as the severity of the crush injury, the timing of treatment, and the character of initial treatment provided to the victim. Delivery of fluids to a victim of crush injury is the critical feature in the proper resuscitation of the patient. Other interventions are often promoted including mannitol (15,16,17) and bicarbonate (15,16,18,19); however, animal and human data are not conclusive, and currently the literature in inconclusive.
The 1988 Armenian earthquake experience underscores the importance of fluid resuscitation, where hundreds of victims had delays to treatment of 24 to 48 hours (20). This holdup resulted in a large number of patients with renal failure and subsequently a very large demand for hemodialysis among these victims. The time to recovery is a significant factor in determining the success of extrication from collapsed structures (Fig. 21-1) (21).
Better et al. (21,22) reported that administration of an average of 12 L/day of fluid with sodium bicarbonate and mannitol to 75-kg adults successfully prevented renal failure in severe crush syndrome cases. In this report, the patients received initial infusion of fluid on site, and most were admitted to the hospital within 30 minutes where they were given massive fluid, sodium bicarbonate, and mannitol. In the Hanshin-Awaji earthquake paper (24), the intravenous fluids were given in conjunction with sodium bicarbonate during the initial few hours (to correct only metabolic acidosis) and low dose dopamine for several days to maintain urine output above 200 mL/hour without mannitol-alkaline diuresis.
Crush victims may have a variety of causes of renal failure, including acute dehydration or bleeding. Patients may have hypotension, prerenal azotemia, decreased urine output, and hemoconcentration. Conditions leading to shock should not be confused with crush syndrome because they have a different pathogenesis. Experience from earthquakes indicates that hypotension and hyperkalemia are the top two causes of death in the first day following rescue (3) (Fig. 21-2). Hypotension in patients with upper extremity crush syndrome usually cannot be explained solely by third spacing, so other sources of shock must be considered. Calculation of the fractional excretion of sodium (FENa) is a classic way to determine if the patient is simply dehydrated; in this case, the renal tubule conservation of sodium is maximized (Fig. 21-3). The ATN disrupts cell function; hence, sodium conservation is compromised leading to a high fractional sodium excretion. A low fractional sodium excretion can be misleading in ATN because of rhabdomyolysis. The creatinine level may be initially normal but rises rapidly after the rhabdomyolysis of crush injury. Acute renal failure from other causes may manifest with a more slowly rising creatinine. Metabolic acidosis, hyperphosphatemia, hypocalcemia, hyperuricemia, and a low fractional sodium excretion are more likely to be present in ATN secondary to rhabdomyolysis. Limb paralysis in a trauma victim may be from acute nerve trauma not necessarily related to rhabdomyolysis.
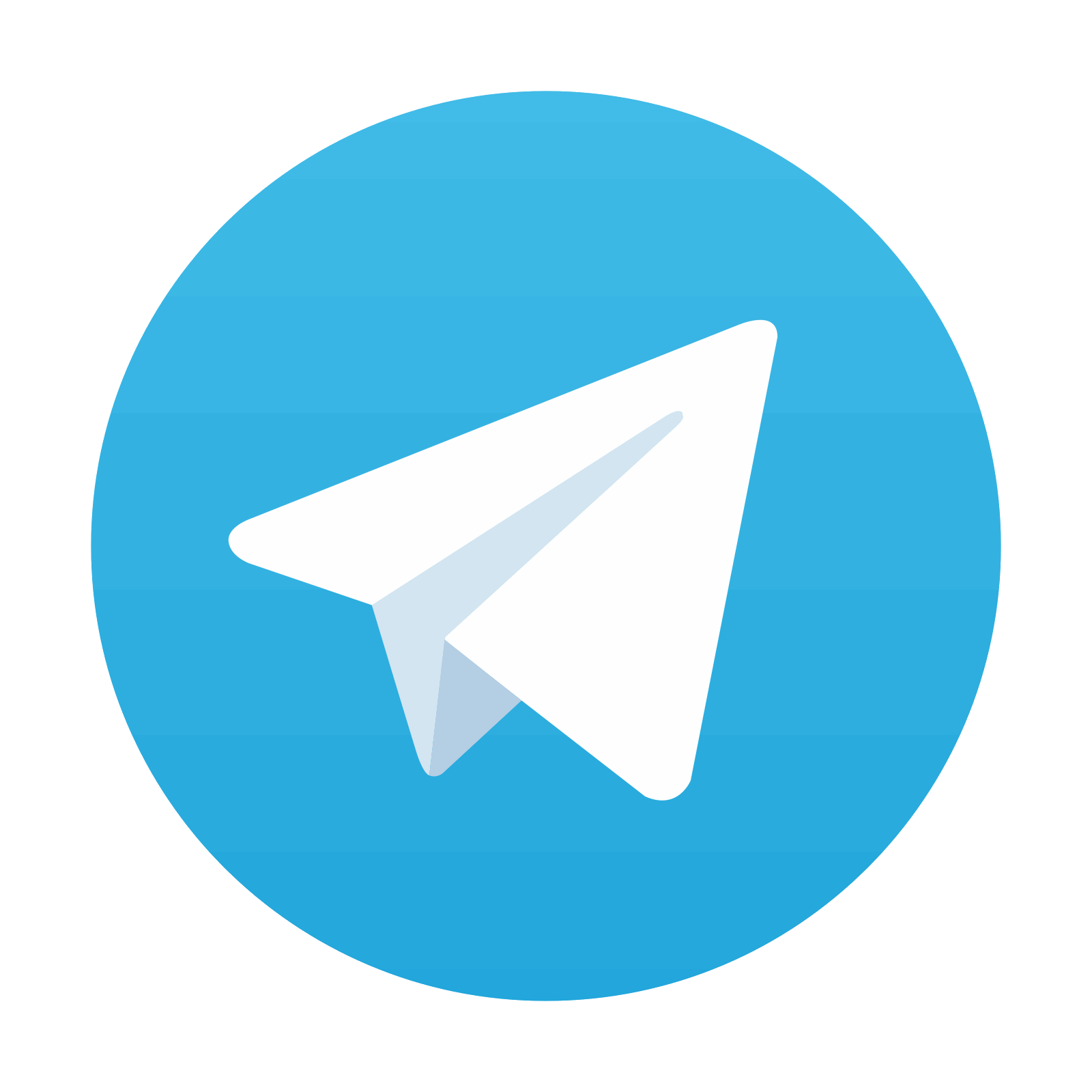
Stay updated, free articles. Join our Telegram channel

Full access? Get Clinical Tree
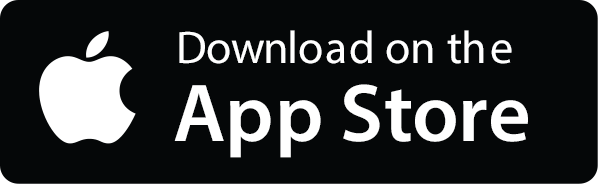
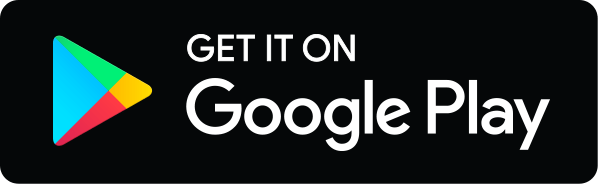