KEY POINTS
The small intestine performs a diverse set of functions.
Small bowel obstruction is one of the most common surgical diagnoses.
Most cases of small bowel obstruction are due to adhesions from previous surgery and resolve with conservative management.
Tumors and malignancies of the small bowel are rare and difficult to diagnose.
Small bowel may be the source of gastrointestinal bleeding, which may be difficult to diagnose.
If following surgical resection, less than 200 cm of small bowel remains, patients are at risk of developing short bowel syndrome.
INTRODUCTION
The small intestine is the raison d’être of the gastrointestinal tract as it is the principle site of nutrient digestion and absorption.1 The small intestine is also the body’s largest reservoir of immunologically active and hormone-producing cells and hence can be conceptualized as the largest organ of the immune and endocrine systems, respectively. It achieves this diversity of action through unique anatomic features, which provide it with a massive surface area, a diversity of cell types, and a complex neural network to coordinate these functions.
Despite its size and importance, diseases of the small intestine are relatively infrequent and present diagnostic and therapeutic challenges. Treatments for common conditions such as postoperative ileus are hardly more effective than those used at the dawn of the last century. Mortality rates associated with acute mesenteric ischemia have not improved during the past 50 years.
Despite introduction of novel imaging techniques such as capsule endoscopy and double balloon endoscopy, diagnostic tests lack sufficient predictive power to definitively guide clinical decision making for individual patients. Furthermore, few high-quality, controlled data on the efficacy of surgical therapies for small bowel diseases are available.
Therefore, sound clinical judgment and a thorough understanding of anatomy, physiology, and pathophysiology remain essential to the care of patients with intestinal disorders.
GROSS ANATOMY
The small intestine is a tubular structure that extends from the pylorus to the cecum. The estimated length of this structure varies depending on whether radiologic, surgical, or autopsy measurements are made. In the living, it is thought to measure 4 to 6 m.2 The small intestine consists of three segments lying in series: the duodenum, the jejunum, and the ileum. The duodenum, the most proximal segment, lies in the retroperitoneum immediately adjacent to the head and inferior border of the body of the pancreas. The duodenum is demarcated from the stomach by the pylorus and from the jejunum by the ligament of Treitz. The jejunum and ileum lie within the peritoneal cavity and are tethered to the retroperitoneum by a broad-based mesentery. No distinct anatomic landmark demarcates the jejunum from the ileum; the proximal 40% of the jejunoileal segment is arbitrarily defined as the jejunum and the distal 60% as the ileum. The ileum is demarcated from the cecum by the ileocecal valve.
The small intestine contains internal mucosal folds known as plicae circulares or valvulae conniventes that are visible upon gross inspection. These folds are also visible radiographically and help in the distinction between small intestine and colon, which does not contain them, on abdominal radiographs. These folds are more prominent in the proximal intestine than in the distal small intestine. Other features evident on gross inspection that are more characteristic of the proximal than distal small intestine include larger circumference, thicker wall, less fatty mesentery, and longer vasa recta (Fig. 28-1). Gross examination of the small-intestinal mucosa also reveals aggregates of lymphoid follicles. Those follicles, located in the ileum, are the most prominent and are designated Peyer’s patches.
Most of the duodenum derives its arterial blood from branches of both the celiac and the superior mesenteric arteries. The distal duodenum, the jejunum, and the ileum derive their arterial blood from the superior mesenteric artery. Their venous drainage occurs via the superior mesenteric vein. Lymph drainage occurs through lymphatic vessels coursing parallel to corresponding arteries. This lymph drains through mesenteric lymph nodes to the cisterna chyli, then through the thoracic duct, and ultimately into the left subclavian vein. The parasympathetic and sympathetic innervation of the small intestine is derived from the vagus and splanchnic nerves, respectively.
HISTOLOGY
The wall of the small intestine consists of four distinct layers: mucosa, submucosa, muscularis propria, and serosa (Fig. 28-2).
The mucosa is the innermost layer, and it consists of three layers: epithelium, lamina propria, and muscularis mucosae. The epithelium is exposed to the intestinal lumen and is the surface through which absorption from and secretion into the lumen occurs. The lamina propria is located immediately external to the epithelium and consists of connective tissue and a heterogeneous population of cells. It is demarcated from the more external submucosa by the muscularis mucosae, a thin sheet of smooth muscle cells.
The mucosa is organized into villi and crypts (crypts of Lieberkuhn). Villi are finger-like projections of epithelium and underlying lamina propria that contain blood and lymphatic (lacteals) vessels that extend into the intestinal lumen. Intestinal, epithelial cellular proliferation is confined to the crypts, each of which carries 250 to 300 cells. All epithelial cells in each crypt are derived from an unknown number of multipotent stem cells located at or near the crypt’s base. Our understanding of these crypt cells is rapidly expanding. It appears that there are two subgroups of intestinal stem cells, with specific cell markers. Bmi1-positive cells are usually quiescent, radiation-resistant cells that are induced by injury, whereas LGR5-positive cells facilitate homeostatic vs. injury-induced regeneration and are radiation sensitive.3
The stem cells can differentiate along one of four pathways that ultimately yield enterocytes and goblet, enteroendocrine, and Paneth cells. With the exception of Paneth cells, these lineages complete their terminal differentiation during an upward migration from each crypt to adjacent villi. The journey from the crypt to the villus tip is completed in 2 to 5 days and terminates with cells being removed by apoptosis and/or exfoliation. Thus, the small-intestinal epithelium undergoes continuous renewal, making it one of the body’s most dynamic tissues. The high cellular turnover rate contributes to mucosal resiliency but also makes the intestine uniquely susceptible to certain forms of injury such as that induced by radiation and chemotherapy.
Enterocytes are the predominant absorptive cell of the intestinal epithelium. Their apical (lumen-facing) cell membrane contains specialized digestive enzymes, transporter mechanisms, and microvilli that are estimated to increase the absorptive surface area of the small intestine by up to 40-fold. Goblet cells produce mucin believed to play a role in mucosal defense against pathogens. Enteroendocrine cells are characterized by secretory granules containing regulatory agents and are discussed in greater detail later in the Endocrine Function section. Paneth cells are located at the base of the crypt and contain secretory granules containing growth factors, digestive enzymes, and antimicrobial peptides. In addition, the intestinal epithelium contains M cells and intraepithelial lymphocytes. These two components of the immune system are discussed later.
The submucosa consists of dense connective tissue and a heterogeneous population of cells, including leukocytes and fibroblasts. The submucosa also contains an extensive network of vascular and lymphatic vessels, nerve fibers, and ganglion cells of the submucosal (Meissner’s) plexus.
The muscularis propria consists of an outer, longitudinally oriented layer and an inner, circularly oriented layer of smooth muscle fibers. Located at the interface between these two layers are ganglion cells of the myenteric (Auerbach’s) plexus.
The serosa consists of a single layer of mesothelial cells and is a component of the visceral peritoneum.
DEVELOPMENT
The first recognizable precursor of the small intestine is the embryonic gut tube, formed from the endoderm during the fourth week of gestation. The gut tube is divided into forgut, midgut, and hindgut. Other than duodenum, which is a forgut structure, the rest of the small intestine is derived from the midgut. The gut tube initially communicates with the yolk sac; however, the communication between these two structures narrows by the sixth week to form the vitelline duct. The yolk sac and vitelline duct usually undergo obliteration by the end of gestation. Incomplete obliteration of the vitelline duct results in the spectrum of defects associated with Meckel’s diverticulum.
Also during the fourth week of gestation, the mesoderm of the embryo splits. The portion of mesoderm that adheres to the endoderm forms the visceral peritoneum, while the portion that adheres to the ectoderm forms the parietal peritoneum. This mesodermal division results in the formation of a coelomic cavity that is the precursor of the peritoneal cavity.
At approximately the fifth week of gestation, the bowel begins to lengthen to an extent greater than that which can be accommodated by the developing abdominal cavity, resulting in the extracoelomic herniation of the developing bowel. The bowel continues to lengthen during the subsequent weeks and is retracted back into the abdominal cavity during the tenth week of gestation. Subsequently, the duodenum becomes a retroperitoneal structure. Coincident with extrusion and retraction, the bowel undergoes a 270° counterclockwise rotation relative to the posterior abdominal wall. This rotation accounts for the usual locations of the cecum in the right lower quadrant and the duodenojejunal junction to the left of midline (Fig. 28-3).
Figure 28-3.
Developmental rotation of the intestine. A. During the fifth week of gestation, the developing intestine herniates out of the coelomic cavity and begins to undergo a counterclockwise rotation about the axis of the superior mesenteric artery. B and C. Intestinal rotation continues, as the developing transverse colon passes anterior to the developing duodenum. D. Final positions of the small intestine and colon resulting from a 270° counterclockwise rotation of the developing intestine and its return into the abdominal cavity.
The celiac and superior mesenteric arteries and veins are derived from the vitelline vascular system, which in turn is derived from blood vessels formed within the splanchnopleuric mesoderm during the third week of gestation. Neurons found in the small intestine are derived from neural crest cells that begin to migrate away from the neural tube during the third week of gestation. These neural crest cells enter the mesenchyme of the primitive foregut and subsequently migrate to the remainder of the bowel.
During the sixth week of gestation, the lumen of the developing bowel becomes obliterated as bowel epithelial proliferation accelerates. Vacuoles form within the bowel substance during the subsequent weeks and coalesce to form the intestinal lumen by the ninth week of gestation. Errors in this recanalization may account for defects such as intestinal webs and stenoses. Most intestinal atresias, however, are believed to be related to ischemic episodes occurring after organogenesis has been completed rather than to errors in recanalization.
During the ninth week of gestation, the intestinal epithelium develops intestine-specific features such as crypt-villus architecture. Organogenesis is complete by approximately the twelfth week of gestation.
PHYSIOLOGY
The intestinal epithelium is the interface through which absorption and secretion occur. It has features characteristic of absorptive epithelia in general including epithelial cells with cellular membranes possessing distinct apical (luminal) and basolateral (serosal) domains demarcated by intercellular tight junctions and an asymmetric distribution of transmembrane transporter mechanisms that promotes vectorial transport of solutes across the epithelium.
Solutes can traverse the epithelium by active or passive transport. Passive transport of solutes occurs through diffusion or convection and is driven by existing electrochemical gradients. Active transport is the energy-dependent net transferof solutes in the absence of or against an electrochemical gradient.
Active transport occurs through transcellular pathways (through the cell), whereas passive transport can occur through either transcellular or paracellular pathways (between cells through the tight junctions). Transcellular transport requires solutes to traverse the cell membranes through specialized membrane proteins, such as channels, carriers, and pumps. The molecular characterization of transporter proteins is evolving rapidly, with different transporter families, each containing many individual genes encoding specific transporters, now identified. Similarly, understanding of the paracellular pathway is evolving. In contrast to what was once believed, it is becoming apparent that paracellular permeability is substrate-specific, dynamic, and subject to regulation by specific tight junction proteins.
Eight to nine L of fluid enters the small intestine daily. Most of this volume consists of salivary, gastric, biliary, pancreatic, and intestinal secretions. Under normal conditions, the small intestine absorbs over 80% of this fluid, leaving approximately 1.5 L that enters the colon (Fig. 28-4). Small-intestinal absorption and secretion are tightly regulated; derangements in water and electrolyte homeostasis characteristic of many of the disorders discussed in this chapter play an important role in contributing to their associated clinical features.
Gut epithelia have two pathways for water transport: (a) the paracellular route, which involves transport through the spaces between cells, and (b) the transcellular route, through apical and basolateral cell membranes. Although most water absorption was thought to occur through the paracellular pathway, it is now well documented that most intestinal water transport occurs through the transcellular pathway.4 The specific transport mechanisms mediating this transcellular transport are not completely characterized and may involve passive diffusion through the phospholipid bilayer, cotransport with other ions and nutrients, or diffusion through water channels called aquaporins. Many different types of aquaporins have been identified; however, their contribution to overall intestinal water absorption appears to be relatively minor.5
The prevailing model for intestinal epithelial Na+ absorption is shown in Fig. 28-5. Activity of the Na+/K+ ATPase enzyme, which is located in the basolateral membrane and exchanges three intracellular Na+ for every two extracellular K+ in an energy-dependent process, generates the electrochemical gradient that drives the transport of Na+ from the intestinal lumen into the cytoplasm of enterocytes. Na+ ions traverse the apical membrane through several distinct transporter mechanisms including nutrient-coupled sodium transport (e.g., sodium glucose cotransporter-1 [SGLT1]), sodium channels, and sodium-hydrogen exchangers (NHEs). Absorbed Na+ ions are then extruded from enterocytes through the Na+/K+ ATPase located in the basolateral membrane. Similar mechanistic models that account for the transport of other common ions such as K+ and HCO3– also exist.
Figure 28-5.
Model of transepithelial sodium (Na+) absorption. Na+ traverses the apical membrane of enterocytes through a variety mechanisms, including nutrient-coupled Na+ transport, Na+/H+ exchange, and Na+ channels. Activity of the Na+/K+ ATPase located on the basolateral membrane generates the electrochemical gradient that provides the driving force for Na+ absorption.
Substantial heterogeneity, with respect to both crypt-villus and craniocaudal axes, exists for intestinal epithelial transport mechanisms. This spatial distribution pattern is consistent with a model in which absorptive function resides primarily in the villus and secretory function in the crypt.
Intestinal absorption and secretion are subject to modulation under physiologic and pathophysiologic conditions by a wide array of hormonal, neural, and immune regulatory mediators (Table 28-1).
Agents that stimulate absorption or inhibit secretion of water Aldosterone Glucocorticoids Angiotensin Norepineprhine Epinephrine Dopamine Somatostatin Neuropeptide Y Peptide YY Enkephalin Agents that simulate secretion or inhibit absorption of water Secretin Bradykinin Prostaglandins Acetylcholine Atrial natriuretic factor Vasopressin Vasoactive intestinal peptide Bombesin Substance P Serotonin Neurotensin Histamine |
Approximately 45% of energy consumption in the average Western diet consists of carbohydrates, approximately one half of which is in the form of starch (linear or branched polymers of glucose) derived from cereals and plants. Other major sources of dietary carbohydrates include sugars derived from milk (lactose), fruits and vegetables (fructose, glucose, and sucrose), or purified from sugar cane or beets (sucrose). Processed foods contain a variety of sugars including fructose, oligosaccharides, and polysaccharides. Glycogen derived from meat contributes only a small fraction of dietary carbohydrate.
Pancreatic amylase is the major enzyme of starch digestion, although salivary amylase initiates the process. The terminal products of amylase-mediated starch digestion are oligosaccharides, maltotriose, maltose, and α-limit dextrins (Fig. 28-6). These products, as well as the major disaccharides in the diet (sucrose and lactose), are unable to undergo absorption in this form. They must first undergo hydrolytic cleavage into their constituent monosaccharides; these hydrolytic reactions are catalyzed by specific brush border membrane hydrolases that are expressed most abundantly in the villi of the duodenum and jejunum. The three major monosaccharides that represent the terminal products of carbohydrate digestion are glucose, galactose, and fructose.
Figure 28-6.
Carbohydrate digestion. Dietary carbohydrates, including starch and the disaccharides sucrose and lactose, must undergo hydrolysis into constituent monosaccharides glucose, galactose, and fructose before being absorbed by the intestinal epithelium. These hydrolytic reactions are catalyzed by salivary and pancreatic amylase and by enterocyte brush border hydrolases.
Under physiologic conditions, most of these sugars are absorbed through the epithelium via the transcellular route. Glucose and galactose are transported through the enterocyte brush border membrane via intestinal Na+-glucose cotransporter, SGLT1 (Fig. 28-7). Fructose is transported through the brush border membrane by facilitated diffusion via GLUT5 (a member of the facilitative glucose transporter family). All three monosaccharides are extruded through the basolateral membrane by facilitated diffusion using GLUT2 and GLUT5 transporters. Extruded monosaccharides diffuse into venules and ultimately enter the portal venous system.
Figure 28-7.
Hexose transporters. Glucose and galactose enter the enterocyte through secondary active transport via the sodium-glucose cotransporter (SGLT1) located on the apical (brush border) membrane. Fructose enters through facilitated diffusion via glucose transporter 5 (GLUT5). Glucose and galactose are extruded basolaterally through facilitated diffusion via glucose transporter 2 (GLUT2). Fructose is extruded basolaterally via GLUT5.
There is evidence of overexpression of hexose transporters, specifically SGLT1, in disease states such as diabetes.6 Several approaches aimed at downregulation of intestinal glucose transporter are being investigated as a novel therapy for disease states such as diabetes and obesity.7
Ten to fifteen percent of energy consumption in the average Western diet consists of proteins. In addition to dietary proteins, approximately one half of the protein load that enters the small intestine is derived from endogenous sources including salivary and gastrointestinal secretions and desquamated intestinal epithelial cells. Protein digestion begins in the stomach with action of pepsins. This is not, however, an essential step, since surgical patients who are acholorhydric or who have lost part or all their stomach are still able to successfully digest proteins. Digestion continues in the duodenum with the actions of a variety of pancreatic peptidases. These enzymes are secreted as inactive proenzymes. This is in contrast to pancreatic amylase and lipase, which are secreted in their active forms. In response to the presence of bile acids, enterokinase is liberated from the intestinal brush border membrane to catalyze the conversion of trypsinogen to active trypsin; trypsin in turn activates itself and other proteases. The final products of intraluminal protein digestion consist of neutral and basic amino acids and peptides two to six amino acids in length (Fig. 28-8). Additional digestion occurs through the actions of peptidases that exist in the enterocyte brush border and cytoplasm. Epithelial absorption occurs for both single amino acids and di- or tripeptides via specific membrane-bound transporters. Absorbed amino acids and peptides then enter the portal venous circulation.
Figure 28-8.
Protein digestion. Dietary proteins must undergo hydrolysis into constituent single amino acids and di- and tripeptides before being absorbed by the intestinal epithelium. These hydrolytic reactions are catalyzed by pancreatic peptidases (e.g., trypsin) and by enterocyte brush border peptidases.
Of all amino acids, glutamine appears to be a unique, major source of energy for enterocytes. Active glutamine uptake into enterocytes occurs through both apical and basolateral transport mechanisms.
Approximately 40% of the average Western diet consists of fat. Over 95% of dietary fat is in the form of long-chain triglycerides; the remainder includes phospholipids such as lecithin, fatty acids, cholesterol, and fat-soluble vitamins. Over 94% of the ingested fats are absorbed in the proximal jejunum.
Since fats are normally water insoluble, key to successful digestion of ingested fats is solubalization of them into an emulsion by the mechanical actions of mastication and antral peristalsis. Although lipolysis of triglycerides to form fatty acids and monoglyciderides is initiated in the stomach by gastric lipase, its principal site is the proximal intestine, where pancreatic lipase is the catalyst (Fig. 28-9).
Figure 28-9.
Fat digestion. Long-chain triglycerides, which constitute the majority of dietary fats, must undergo lipolysis into constituent log-chain fatty acids and monoglycerides before being absorbed by the intestinal epithelium. These reactions are catalyzed by gastric and pancreatic lipases. The products of lipolysis are transported in the form of mixed micelles to enterocytes, where they are resynthesized into triglycerides, which are then packaged in the form of chylomicrons that are secreted into the intestinal lymph (chyle). Triglycerides composed of short- and medium-chain fatty acids are absorbed by the intestinal epithelium directly, without undergoing lipolysis, and are secreted into the portal venous circulation.
Bile acids act as detergents that help in solubalization of the lipolysis by forming mixed micelles. These micelles are polymolecular aggregates with a hydrophobic core of fat and a hydrophilic surface that act as shuttles, delivering the products of lipolysis to the enterocyte brush border membrane, where they are absorbed. The bile salts, however, remain in the bowel lumen and travel to the terminal ileum, where they are actively resorbed. They enter the portal circulation and are resecreted into bile, thus completing the enterohepatic circulation.
Dissociation of lipids from the micelles occurs in a thin layer of water (50–500 μm thick) with an acidic microenvironment immediately adjacent to the brush border called the unstirred water layer. Most lipids are absorbed in the proximal jejunum, whereas bile salts are absorbed in the distal ileum through an active process. Fatty acid binding proteins (FABPs) are a family of proteins located on the brush border membrane, facilitating diffusion of long-chain fatty acids across the brush border membrane. Cholesterol crosses the brush border membrane through an active process that is yet to be completely characterized. Within the enterocytes, triglycerides are resynthesized and incorporated into chylomicrons that are secreted into the intestinal lymphatics and ultimately enter the thoracic duct. In these chylomicrons, lipoproteins serve a detergent-like role similar to that served by bile salts in the mixed micelles.
The steps described above are required for the digestion and absorption of triglycerides containing long-chain fatty acids. However, triglycerides containing short- and medium-chain fatty acids are more hydrophilic and are absorbed without undergoing intraluminal hydrolysis, micellular solubilization, mucosal re-esterification, and chylomicron formation. Instead, they are directly absorbed and enter the portal venous circulation rather than the lymphatics. This information provides the rationale for administering nutritional supplements containing medium-chain triglycerides to patients with gastrointestinal diseases associated with impaired digestion and/or malabsorption of long-chain triglycerides.
Vitamin B12 (cobalamin) malabsorption can result from a variety of surgical manipulations. The vitamin is initially bound by saliva-derived R protein. In the duodenum, R protein is hydrolyzed by pancreatic enzymes, allowing free cobalamin to bind to gastric parietal cell–derived intrinsic factor. The cobalamin-intrinsic factor complex is able to escape hydrolysis by pancreatic enzymes, allowing it to reach the terminal ileum, which expresses specific receptors for intrinsic factor. Subsequent events in cobalamin absorption are poorly characterized, but the intact complex probably enters enterocytes through translocation. Because each of these steps is necessary for cobalamin assimilation, gastric resection, gastric bypass, and ileal resection can each result in vitamin B12 insufficiency.
Other water-soluble vitamins for which specific carrier-mediated transport processes have been characterized include ascorbic acid, folate, thiamine, riboflavin, pantothenic acid, and biotin. Fat-soluble vitamins A, D, and E appear to be absorbed through passive diffusion. Vitamin K appears to be absorbed through both passive diffusion and carrier-mediated uptake.
Calcium is absorbed through both transcellular transport and paracellular diffusion. The duodenum is the major site for transcellular transport; paracellular transport occurs throughout the small intestine. A key step in transcellular calcium transport is mediated by calbindin, a calcium-binding protein located in the cytoplasm of enterocytes. Regulation of calbindin synthesis is the principle mechanism by which vitamin D regulates intestinal calcium absorption. Abnormal calcium levels are increasingly seen in surgical patients who have undergone a gastric bypass. Although usual calcium supplementation is often in the form of calcium carbonate, which is cheap, in such patients with low acid exposure, calcium citrate is a better formulation for supplemental therapy.
Iron and magnesium are each absorbed through both transcellular and paracellular routes. A divalent metal transporter capable of transporting Fe2+, Zn2+, Mn2+, Co2+, Cd2+, Cu2+, Ni2+, and Pb2+ that has recently been localized to the intestinal brush border may account for at least a portion of the transcellular absorption of these ions.8
Although the intestinal epithelium allows for the efficient absorption of dietary nutrients, it must discriminate between pathogens and harmless antigens such as food proteins and commensal bacteria, and it must resist invasion by pathogens. Factors contributing to epithelial defense include immunoglobulin A (IgA), mucins, and the relative impermeability of the brush border membrane and tight junctions to macromolecules and bacteria. Recently described factors likely to play important roles in intestinal mucosal defense include antimicrobial peptides such as the defensins.9 The intestinal component of the immune system, known as the gut-associated lymphoid tissue (GALT), contains over 70% of the body’s immune cells.
The GALT is conceptually divided into inductive and effector sites.10 Inductive sites include Peyer’s patches, mesenteric lymph nodes, and smaller isolated lymphoid follicles scattered throughout the small intestine (Fig. 28-10). Peyer’s patches are macroscopic aggregates of B-cell follicles and intervening T-cell areas found in the lamina propria of the small intestine, primarily the distal ileum. Overlying Peyer’s patches are a specialized epithelium containing microfold (M) cells. These cells possess an apical membrane with microfolds rather than microvilli, which is characteristic of most intestinal epithelial cells. Using transepithelial vesicular transport, M cells transfer microbes to underlying professional antigen-presenting cells (APCs), such as dendritic cells. Dendritic cells, in addition, may sample luminal antigens directly through their dendrite-like processes that extend through epithelial tight junctions. APCs interact with and prime naïve lymphocytes, which then exit through the draining lymphatics to enter the mesenteric lymph nodes where they undergo differentiation. These lymphocytes then migrate into the systemic circulation via the thoracic duct and ultimately accumulate in the intestinal mucosa at effector sites. Alternative induction mechanisms, such as antigen presentation within mesenteric lymph nodes, are also likely to exist.
Figure 28-10.
Gut-associated lymphoid tissue. Select components of the gut-associated lymphoid tissue (GALT) are schematically represented. Peyer’s patches consist of a specialized follicle-associated epithelium (FAE) containing M cells, a subepithelial dome (SED) rich in dendritic cells, and B-cell follicle-containing germinal centers (GC). Plasma cells in the lamina propria produce immunoglobulin A (IgA), which is transported to the intestinal lumen where it serves as the first line of defense against pathogens. Other components of GALT include isolated lymphoid follicles, mesenteric lymph nodes, and regulatory and effector lymphocytes.
Effector lymphocytes are distributed into distinct compartments. IgA-producing plasma cells are derived from B cells and are located in the lamina propria. CD4+ T cells are also located in the lamina propria. CD8+ T cells migrate preferentially to the epithelium, but are also found in the lamina propria. These T cells are central to immune regulation; in addition, the CD8+ T cells have potent cytotoxic (CTL) activity. IgA is transported through the intestinal epithelial cells into the lumen, where it exists in the form of a dimer complexed with a secretory component. This configuration renders IgA resistant to proteolysis by digestive enzymes. IgA is believed to both help prevent the entry of microbes through the epithelium and to promote excretion of antigens or microbes that have already penetrated into the lamina propria.
It has been increasingly recognized that the gastrointestinal tract is colonized with many bacteria that are essential for health. Communication between the microbiota and the host defense allows for protective immune responses against pathogens while preventing adverse inflammatory responses to harmless commensal microbes, which could lead to chronic inflammatory disorders such as celiac disease and Crohn’s disease.11
Myocytes of the intestinal muscle layers are electrically and mechanically coordinated in the form of syncytia. Contractions of the muscularis propria are responsible for small-intestinal peristalsis. Contraction of the outer longitudinal muscle layer results in bowel shortening; contraction of the inner circular layer results in luminal narrowing. Contractions of the muscularis mucosa contribute to mucosal or villus motility, but not to peristalsis.
Several distinctive patterns of muscularis propria activity have been observed to occur in the small intestine. These patterns include ascending excitation and descending inhibition in which muscular contraction occurs proximal to a stimulus, such as the presence of a bolus of ingested food, and muscular relaxation occurs distal to the stimulus (Fig. 28-11). These two reflexes are present even in the absence of any extrinsic innervation to the small intestine and contribute to peristalsis when they are propagated in a coordinated fashion along the length of the intestine. The fed or postprandial pattern begins within 10 to 20 minutes of meal ingestion and abates 4 to 6 hours afterward. Rhythmic segmentations or pressure waves traveling only short distances also are observed. This segmenting pattern is hypothesized to assist in mixing intraluminal contents and in facilitating their contact with the absorptive mucosal surface. The fasting pattern or interdigestive motor cycle (IDMC) consists of three phases. Phase I is characterized by motor quiescence, phase II by seemingly disorganized pressure waves occurring at submaximal rates, and phase III by sustained pressure waves occurring at maximal rates. This pattern is hypothesized to expel residual debris and bacteria from the small intestine. The median duration of the IDMC ranges from 90 to 120 minutes. At any given time, different portions of the small intestine can be in different phases of the IDMC.
Figure 28-11.
Ascending excitation and descending inhibition. The presence of a food bolus within the intestinal lumen is sensed by a sensory neuron (SN) that relays signals to (a) excitatory motor neurons (EMN) that have projections to intestinal muscle cells located proximal to the food bolus and (b) inhibitory motor neurons (IMN) that have projections to intestinal muscle cells located distal to the food bolus. This stereotypical motor reflex is controlled by the enteric nervous system and occurs in the absence of extraintestinal innervations. It contributes to peristalsis.
The regulatory mechanisms driving small-intestinal motility consist of both pacemakers intrinsic to the small intestine and external neurohumoral modulatory signals. The interstitial cells of Cajal are pleomorphic mesenchymal cells located within the muscularis propria of the intestine that generate the electrical slow wave (basic electrical rhythm or pacesetter potential) that plays a pacemaker role in setting the fundamental rhythmicity of small-intestinal contractions. The frequency of the slow wave varies along the longitudinal axis of the intestine: it ranges from 12 waves per minute in the duodenum to 7 waves per minute in the distal ileum. Smooth muscle contraction occurs only when an electrical action potential (spike burst) is superimposed on the slow wave. Thus, the slow wave determines the maximum frequency of contractions; however, not every slow wave is associated with a contraction.
This intrinsic contractile mechanism is subject to neural and hormonal regulation. The enteric nervous system (ENS) provides both inhibitory and excitatory stimuli. The predominant excitatory transmitters are acetylcholine and substance P, and the inhibitory transmitters include nitric oxide, vasoactive intestinal peptide, and adenosine triphosphate. In general, the sympathetic motor supply is inhibitory to the ENS; therefore, increased sympathetic input into the intestine leads to decreased intestinal smooth muscle activity. The parasympathetic motor supply is more complex, with projections to both inhibitory and excitatory ENS motor neurons. Correspondingly, the effects of parasympathetic inputs into intestinal motility are more difficult to predict.
Endocrinology as a discipline was born with the discovery of secretin, an intestinal regulatory peptide that was the first hormone to be identified. Our improving understanding of the physiology of the small intestine has lead to identification of many additional intestinal-derived hormones that make this the largest hormone-producing organ in the body. Over 30 peptide hormone genes have been identified as being expressed in the gastrointestinal tract. Because of differential posttranscriptional and posttranslational processing, over 100 distinct regulatory peptides are produced. In addition, monoamines, such as histamine and dopamine, and eicosanoids with hormone-like activities are produced in the intestine.
“Gut hormones” were previously conceptualized as peptides produced by the enteroendocrine cells of the intestinal mucosa that are released into the systemic circulation to reach receptors in target sites in the gastrointestinal tract. Now it is clear that “gut hormone” genes are widely expressed throughout the body, not only in endocrine cells, but also in central and peripheral neurons. The products of these genes are general intercellular messengers that can act as endocrine, paracrine, autocrine, or neurocrine mediators. Thus, they may act as true blood-borne hormones as well as through local effects.
There are notable homology patterns among individual regulatory peptides found in the gastrointestinal tract. Based on these homologies, approximately one half of the known regulatory peptides can be classified into families.12 For example, the secretin family includes secretin, glucagon, and glucagon-like peptides, glucose-dependent insulinotropic peptide, vasoactive intestinal polypeptide, peptide histidine isoleucine, growth hormone–releasing hormone, and pituitary adenylyl cyclase–activating peptide. Other peptide families include those named for insulin, epidermal growth factor, gastrin, pancreatic polypeptide, tachykinin, and somatostatin.
Receptor subtype multiplicity and cell-specific expression patterns for these receptor subtypes that are characteristic of these regulatory mediators make definition of their actions complex. Detailed description of these actions is beyond the scope of this chapter; however, examples of regulatory peptides produced by enteroendocrine cells of the small-intestinal epithelium and their most commonly ascribed functions are summarized in Table 28-2. Some of these peptides, or their analogues, are used in routine clinical practice. For example, therapeutic applications of octreotide, a long-acting analogue of somatostatin, include the amelioration of symptoms associated with neuroendocrine tumors (e.g., carcinoid syndrome), postgastrectomy dumping syndrome, enterocutaneous fistulas, and the initial treatment of acute hemorrhage due to esophageal varices. The gastrin secretory response to secretin administration forms the basis for the standard test used to establish the diagnosis of Zollinger-Ellison syndrome. Cholecystokinin is used in evaluations of gallbladder ejection fraction, a parameter that may have utility in patients who have symptoms of biliary colic but are not found to have gallstones. Of the peptides listed in Table 28-2, glucagon-like peptide-2 (GLP-2) has been identified as a specific and potent intestinotrophic hormone and is currently under clinical evaluation as an intestinotrophic agent in patients suffering from the short bowel syndrome, as discussed in the later Short Bowel Syndrome section.
HORMONE | SOURCEa | ACTIONS |
---|---|---|
Somatostatin | D cell | Inhibits gastrointestinal secretion, motility, and splanchnic perfusion |
Secretin | S cell | Stimulates exocrine pancreatic secretion, stimulates intestinal secretion |
Cholecystokinin | I cell | Simulates pancreatic exocrine secretion, simulates gallbladder emptying, inhibits sphincter of Oddi contraction |
Motilin | M cell | Simulates intestinal motility |
Peptide YY | L cell | Inhibits intestinal motility and secretion |
Glucagon-like peptide 2 | L cell | Stimulates intestinal epithelial proliferation |
Neurotensin | N cell | Stimulates pancreatic and biliary secretion, inhibits small bowel motility, stimulates intestinal mucosal growth |
The small intestine has the capacity to adapt in response to varying demands imposed by physiologic and pathologic conditions. Of particular relevance to many of the diseases discussed in this chapter is the adaptation that occurs in the remnant intestine following surgical resection of a large portion of the small intestine (massive small bowel resection). Postresection intestinal adaptation has been studied extensively using animal models. Within a few hours after bowel resection, the remnant small intestine displays evidence of epithelial cellular hyperplasia. With additional time, villi lengthen, intestinal absorptive surface area increases, and digestive and absorptive functions improve. Postresection intestinal adaptation in human patients is less well studied, but seems to follow similar steps as those seen in experimental models, and takes 1 to 2 years to complete.13
The mechanisms responsible for inducing postresection intestinal adaptation are under active investigation. Several classes of effectors that stimulate intestinal growth include specific nutrients, peptide hormones and growth factors, pancreatic secretions, and some cytokines. Nutritional components with intestinal growth-stimulating effects include fiber, fatty acids, triglycerides, glutamine, polyamines, and lectins.
Postresection adaptation serves to compensate for the function of intestine that has been resected. Jejunal resection is generally better tolerated, as ileum shows better capacity to compensate. However, the magnitude of this response is limited. If enough small intestine is resected, a devastating condition known as the short bowel syndrome results. This condition is discussed in the Short Bowel Syndrome section at the end of this chapter.
SMALL BOWEL OBSTRUCTION
Mechanical small bowel obstruction is the most frequently encountered surgical disorder of the small intestine. Although a wide range of etiologies for this condition exists, the obstructing lesion can be conceptualized according to its anatomic relationship to the intestinal wall as:
Intraluminal (e.g., foreign bodies, gallstones, or meconium)
Intramural (e.g., tumors, Crohn’s disease–associated inflammatory strictures)
Extrinsic (e.g., adhesions, hernias, or carcinomatosis)
Intra-abdominal adhesions related to prior abdominal surgery account for up to 75% of cases of small bowel obstruction. Over 300,000 patients are estimated to undergo surgery to treat adhesion-induced small bowel obstruction in the United States annually. A 20-year trend analysis between 1988 and 2007 has documented no decrease in this rate during this time period, highlighting the ongoing problem with this “old” disease.14
Less prevalent etiologies for small bowel obstruction include hernias, malignant bowel obstruction, and Crohn’s disease. The frequency with which obstruction related to these conditions is encountered varies according to the patient population and practice setting. Cancer-related small bowel obstructions are commonly due to extrinsic compression or invasion by advanced malignancies arising in organs other than the small bowel; few are due to primary small bowel tumors. The most commonly encountered etiologies of small bowel obstruction are summarized in Table 28-3. Although congenital abnormalities capable of causing small bowel obstruction usually become evident during childhood, they sometimes elude detection and are diagnosed for the first time in adult patients presenting with abdominal symptoms. For example, intestinal malrotation and mid-gut volvulus should not be forgotten when considering the differential diagnosis of adult patients with acute or chronic symptoms of small bowel obstruction, especially those without a history of prior abdominal surgery. A rare etiology of obstruction is the superior mesenteric artery syndrome, characterized by compression of the third portion of the duodenum by the superior mesenteric artery as it crosses over this portion of the duodenum. This condition should be considered in young asthenic individuals who have chronic symptoms suggestive of proximal small bowel obstruction.
Adhesions Neoplasms Primary small bowel neoplasms Secondary small bowel cancer (e.g., melanoma-derived metastasis) Local invasion by intra-abdominal malignancy (e.g., desmoid tumors) Carcinomatosis Hernias External (e.g., inguinal and femoral) Internal (e.g., following Roux-en-Y gastric bypass surgery) Crohn’s disease Volvulus Intussusception Radiation-induced stricture Postischemic stricture Foreign body Gallstone ileus Diverticulitis Meckel’s diverticulum Hematoma Congenital abnormalities (e.g., webs, duplications, and malrotation) |
With onset of obstruction, gas and fluid accumulate within the intestinal lumen proximal to the site of obstruction. The intestinal activity increases in an effort to overcome the obstruction, accounting for the colicky pain and the diarrhea that some experience even in the presence of complete bowel obstruction. Most of the gas that accumulates originates from swallowed air, although some is produced within the intestine. The fluid consists of swallowed liquids and gastrointestinal secretions (obstruction stimulates intestinal epithelial water secretion). With ongoing gas and fluid accumulation, the bowel distends and intraluminal and intramural pressures rise. The intestinal motility is eventually reduced with fewer contractions. With obstruction, the luminal flora of the small bowel, which is usually sterile, changes, and a variety of organisms have been cultured from the contents. Translocation of these bacteria to regional lymph nodes has been demonstrated, although the significance of this process is not well understood. If the intramural pressure becomes high enough, intestinal microvascular perfusion is impaired, leading to intestinal ischemia and, ultimately, necrosis. This condition is termed strangulated bowel obstruction.
With partial small bowel obstruction, only a portion of the intestinal lumen is occluded, allowing passage of some gas and fluid. The progression of pathophysiologic events described earlier tends to occur more slowly than with complete small bowel obstruction, and development of strangulation is less likely.
A particularly dangerous form of bowel obstruction is closed-loop obstruction in which a segment of intestine is obstructed both proximally and distally (e.g., with volvulus). In such cases, the accumulating gas and fluid cannot escape either proximally or distally from the obstructed segment, leading to a rapid rise in luminal pressure and a rapid progression to strangulation.
The symptoms of small bowel obstruction are colicky abdominal pain, nausea, vomiting, and obstipation. Vomiting is a more prominent symptom with proximal obstructions than distal. The character of vomitus is important because with bacterial overgrowth, the vomitus is more feculent, suggesting a more established obstruction. Continued passage of flatus and/or stool beyond 6 to 12 hours after onset of symptoms is characteristic of partial rather than complete obstruction. The signs of small bowel obstruction include abdominal distention, which is most pronounced if the site of obstruction is in the distal ileum and may be absent if the site of obstruction is in the proximal small intestine. Bowel sounds may be hyperactive initially, but in late stages of bowel obstruction, minimal bowel sounds may be heard. Laboratory findings reflect intravascular volume depletion and consist of hemoconcentration and electrolyte abnormalities. Mild leukocytosis is common.
Features of strangulated obstruction include abdominal pain often disproportionate to the degree of abdominal findings, suggestive of intestinal ischemia. Patients often have tachycardia, localized abdominal tenderness, fever, marked leukocytosis, and acidosis. Any of these findings should alert the clinician to the possibility of strangulation and need for early surgical intervention.
The diagnostic evaluation should focus on the following goals: (a) distinguish mechanical obstruction from ileus, (b) determine the etiology of the obstruction, (c) discriminate partial from complete obstruction, and (d) discriminate simple from strangulating obstruction.
Important elements to obtain on history include prior abdominal operations (suggesting the presence of adhesions) and the presence of abdominal disorders (e.g., intra-abdominal cancer or inflammatory bowel disease) that may provide insights into the etiology of obstruction. Upon examination, a meticulous search for hernias (particularly in the inguinal and femoral regions) should be conducted.
The diagnosis of small bowel obstruction is usually confirmed with radiographic examination. The abdominal series consists of (a) a radiograph of the abdomen with the patient in a supine position, (b) a radiograph of the abdomen with the patient in an upright position, and (c) a radiograph of the chest with the patient in an upright position. The finding most specific for small bowel obstruction is the triad of dilated small bowel loops (>3 cm in diameter), air-fluid levels seen on upright films, and a paucity of air in the colon. The sensitivity of abdominal radiographs in the detection of small bowel obstruction ranges 70% to 80%.15 Specificity is low, because ileus and colonic obstruction can be associated with findings that mimic those observed with small bowel obstruction. False-negative findings on radiographs can result when the site of obstruction is located in the proximal small bowel and when the bowel lumen is filled with fluid but no gas, thereby preventing visualization of air-fluid levels or bowel distention. The latter situation is associated with closed-loop obstruction. Despite these limitations, abdominal radiographs remain an important study in patients with suspected small bowel obstruction because of their widespread availability and low cost (Fig. 28-12).
Computed tomography (CT) scanning is 80% to 90% sensitive and 70% to 90% specific in the detection of small bowel obstruction.15 The findings of small bowel obstruction include a discrete transition zone with dilation of bowel proximally, decompression of bowel distally, intraluminal contrast that does not pass beyond the transition zone, and a colon containing little gas or fluid (Figs. 28-13 and 28-14). CT scanning may also provide evidence for the presence of closed-loop obstruction and strangulation. Closed-loop obstruction is suggested by the presence of a U-shaped or C-shaped dilated bowel loop associated with a radial distribution of mesenteric vessels converging toward a torsion point. Strangulation is suggested by thickening of the bowel wall, pneumatosis intestinalis (air in the bowel wall), portal venous gas, mesenteric haziness, and poor uptake of intravenous contrast into the wall of the affected bowel (Fig. 28-15). CT scanning also offers a global evaluation of the abdomen and may therefore reveal the etiology of obstruction. This feature is important in the acute setting when intestinal obstruction represents only one of many diagnoses in patients presenting with acute abdominal conditions.
Figure 28-13.
Small bowel obstruction. A computed tomography scan of a patient presenting with signs and symptoms of bowel obstruction. Image shows grossly dilated loops of small bowel, with decompressed terminal ileum (I) and ascending colon (C), suggesting a complete distal small bowel obstruction. At laparotomy, adhesive bands from a previous surgery were identified and divided.
Figure 28-14.
Chronic partial small bowel obstruction. This patient presented with a several-month history of chronic abdominal pain and intermittent vomiting. The coronal computed tomography image shows grossly dilated loops of proximal small bowel on the left side (wide arrow), with decompressed loops of small bowel on the right side (narrow arrow). The dilated segment shows evidence of feculization of bowel contents, consistent with the chronic nature of the obstruction. Patient’s vomitus had characteristic feculent smell and quality. At exploratory laparotomy, adhesive band were identified and divided.
The CT scan is usually performed after administration of oral water-soluble contrast or diluted barium. The water-soluble contrast has been shown to have prognostic and therapeutic value too. Several studies and a subsequent meta-analysis have shown that appearance of the contrast in the colon within 24 hours is predictive of nonsurgical resolution of bowel obstruction.16 Although use of oral contrast did not alter the rate of surgical intervention, it did reduce the overall length of hospital stay in those presenting with small bowel obstruction.
A limitation of CT scanning is its low sensitivity (<50%) in the detection of low-grade or partial small bowel obstruction. A subtle transition zone may be difficult to identify in the axial images obtained during CT scanning. In such cases, contrast examinations of the small bowel, either small bowel series (small bowel follow-through) or enteroclysis, can be helpful. For standard small bowel series, contrast is swallowed or instilled into the stomach through a nasogastric tube. Abdominal radiographs are then taken serially as the contrast travels distally in the intestine. Although barium can be used, water-soluble contrast agents, such as Gastrografin, should be used if the possibility of intestinal perforation exists. These examinations are more labor-intensive and less rapidly performed than CT scanning but may offer greater sensitivity in the detection of luminal and mural etiologies of obstruction, such as primary intestinal tumors. For enteroclysis, 200 to 250 mL of barium followed by 1 to 2 L of a solution of methylcellulose in water are instilled into the proximal jejunum via a long nasoenteric catheter. The double-contrast technique used in enteroclysis permits a better assessment of mucosal surface and detection of relatively small lesions, even through overlapping small bowel loops. Enteroclysis is rarely performed in the acute setting but offers greater sensitivity than small bowel series in the detection of lesions that may be causing partial small bowel obstruction. Recently, CT enteroclysis has been used and reported to be superior to plain x-ray small bowel contrast studies.
Small bowel obstruction is usually associated with a marked depletion of intravascular volume due to decreased oral intake, vomiting, and sequestration of fluid in bowel lumen and wall. Therefore, fluid resuscitation is integral to treatment. Isotonic fluid should be given intravenously, and an indwelling bladder catheter may be placed to monitor urine output. Central venous or pulmonary artery catheter monitoring may be necessary to assist with fluid management in patients with underlying cardiac disease and severe dehydration. Broad-spectrum antibiotics are given by some because of concerns that bacterial translocation may occur in the setting of small bowel obstruction; however, there are no data to support this approach.
The stomach should be continuously evacuated of air and fluid using a nasogastric (NG) tube. Effective gastric decompression decreases nausea, distention, and the risk of vomiting and aspiration. Longer nasoenteric tubes, with tips placed into the jejunum or ileum, were favored in the past but are rarely used today, as they are associated with higher complication rates than NG tubes, with no proven greater efficacy in several studies.
The standard therapy for complete small bowel obstruction has generally been expeditious surgery, with the dictum that “the sun should never rise and set on a complete bowel obstruction.”Recently, however, some have advocated nonoperative approaches in management of these patients, provided closed-loop obstruction is ruled out and there is no evidence of intestinal ischemia. Such patients need to be observed closely and undergo serial exams. The rationale for those favoring early surgical intervention is to minimize the risk for bowel strangulation, which is associated with an increased risk for morbidity and mortality. Clinical signs and currently available laboratory tests and imaging studies do not reliably permit the distinction between patients with simple obstruction and those with strangulated obstruction prior to the onset of irreversible ischemia. Therefore, the goal is to operate before the onset of irreversible ischemia. Others note, however, that a period of observation and nasogastric decompression, provided no tachycardia, tenderness, or an increase in white cell count is noted, are appropriate (see Fig. 28-16 for a proposed management algorithm).
However, conservative therapy, in the form of NG decompression and fluid resuscitation, is commonly recommended in the initial recommendation for:
Partial small bowel obstruction
Obstruction occurring in the early postoperative period
Intestinal obstruction due to Crohn’s disease
Carcinomatosis
In partial obstruction, progression to strangulation is unlikely to occur, and an attempt at nonoperative resolution is warranted. Nonoperative management has been documented to be successful in 65% to 81% of patients with partial small bowel obstruction. Of those successfully treated nonoperatively, only 5% to 15% have been reported to have symptoms that were not substantially improved within 48 hours after initiation of therapy.17 Therefore, most patients with partial small bowel obstruction whose symptoms do not improve within 48 hours after initiation of nonoperative therapy should undergo surgery. In a recent study, using the National Inpatient Sample, this principle was further highlighted. The authors concluded that a 2-day limit of watchful waiting before surgery is not associated with an increase in mortality or postoperative morbidity, although inpatient costs were higher.18
Patients undergoing nonoperative therapy should be closely monitored for signs suggestive of peritonitis, the development of which would mandate urgent surgery. As stated before, the administration of hypertonic water-soluble contrast agents, such as Gastrografin used in upper gastrointestinal (GI) and small bowel follow-through examinations, causes a shift of fluid into the intestinal lumen, thereby increasing the pressure gradient across the site of obstruction. This effect may accelerate resolution of partial small bowel obstruction; however, there is less evidence that administration of water-soluble contrast agents increases the probability that an episode of bowel obstruction will be successfully managed nonoperatively.16
Obstruction presenting in the early postoperative period has been reported to occur in 0.7% patients undergoing laparotomy.19 Patients undergoing pelvic surgery, especially colorectal procedures, have the greatest risk for developing early postoperative small bowel obstruction. The presence of obstruction should be considered if symptoms of intestinal obstruction occur after the initial return of bowel function or if bowel function fails to return within the expected 3 to 5 days after abdominal surgery. Plain radiographs may demonstrate dilated loops of small intestine with air-fluid levels but are interpreted as normal or nonspecific in up to a third of patients with early postoperative obstruction. CT scanning or a small bowel series is often required to make the diagnosis. Obstruction that occurs in the early postoperative period is usually partial and only rarely is associated with strangulation. Therefore, a period of extended nonoperative therapy (2–3 weeks) consisting of bowel rest, hydration, and total parental nutrition (TPN) administration is usually warranted. However, if complete obstruction is demonstrated or if signs suggestive of peritonitis are detected, expeditious reoperation should be undertaken without delay.
Crohn’s disease as a cause of small bowel obstruction is discussed in more detail later in the Crohn’s Disease section.
Twenty-five to thirty-three percent of patients with a history of cancer who present with small bowel obstruction have adhesions as the etiology of their obstruction and therefore should not be denied appropriate therapy.20 Even in cases in which the obstruction is related to recurrent malignancy, palliative resection or bypass can be performed. Patients with obvious carcinomatosis pose a difficult challenge, given their limited prognosis. Management must be tailored to an individual patient’s prognosis and desires, and relief of the obstruction may be best achieved by a bypass procedure, avoiding a potentially difficult bowel resection.
The operative procedure performed for small bowel obstruction varies according to the etiology of the obstruction. For example, adhesions are lysed, tumors are resected, and hernias are reduced and repaired. Regardless of the etiology, the affected intestine should be examined, and nonviable bowel resected. Criteria suggesting viability are normal color, peristalsis, and marginal arterial pulsations. Usually, visual inspection alone is adequate in judging viability. In borderline cases, a Doppler probe may be used to check for pulsatile flow to the bowel, and arterial perfusion can be verified by visualizing intravenously administered fluorescein dye in the bowel wall under ultraviolet illumination. However, neither technique has been found to be superior to clinical judgment. In general, if the patient is hemodynamically stable, short lengths of bowel of questionable viability should be resected and primary anastomosis of the remaining intestine performed. However, if the viability of a large proportion of the intestine is in question, a concerted effort to preserve intestinal tissue should be made. In such situations, the bowel of uncertain viability should be left intact and the patient re-explored in 24 to 48 hours in a “second-look” operation. At that time, definitive resection of nonviable bowel is completed.
Successful laparoscopic surgery for bowel obstruction is being reported with greater frequency.22,23 Those who undergo successful laparoscopic procedure have a quicker recovery, less complications, and lower costs. Since distended loops of bowel can interfere with adequate visualization, early cases of proximal small bowel obstruction that are likely due to a single adhesive band are best suited for this approach. Presence of bowel distention and multiple adhesions can cause these procedures to be difficult and potentially hazardous. Conversion rate to open surgery is between 17% and 33%.21,22,23
Prognosis is related to the etiology of obstruction. The majority of patients who are treated conservatively for adhesive small bowel obstruction do not require future readmissions; less than 20% of such patients will have a readmission over the subsequent 5 years with another episode of bowel obstruction.23
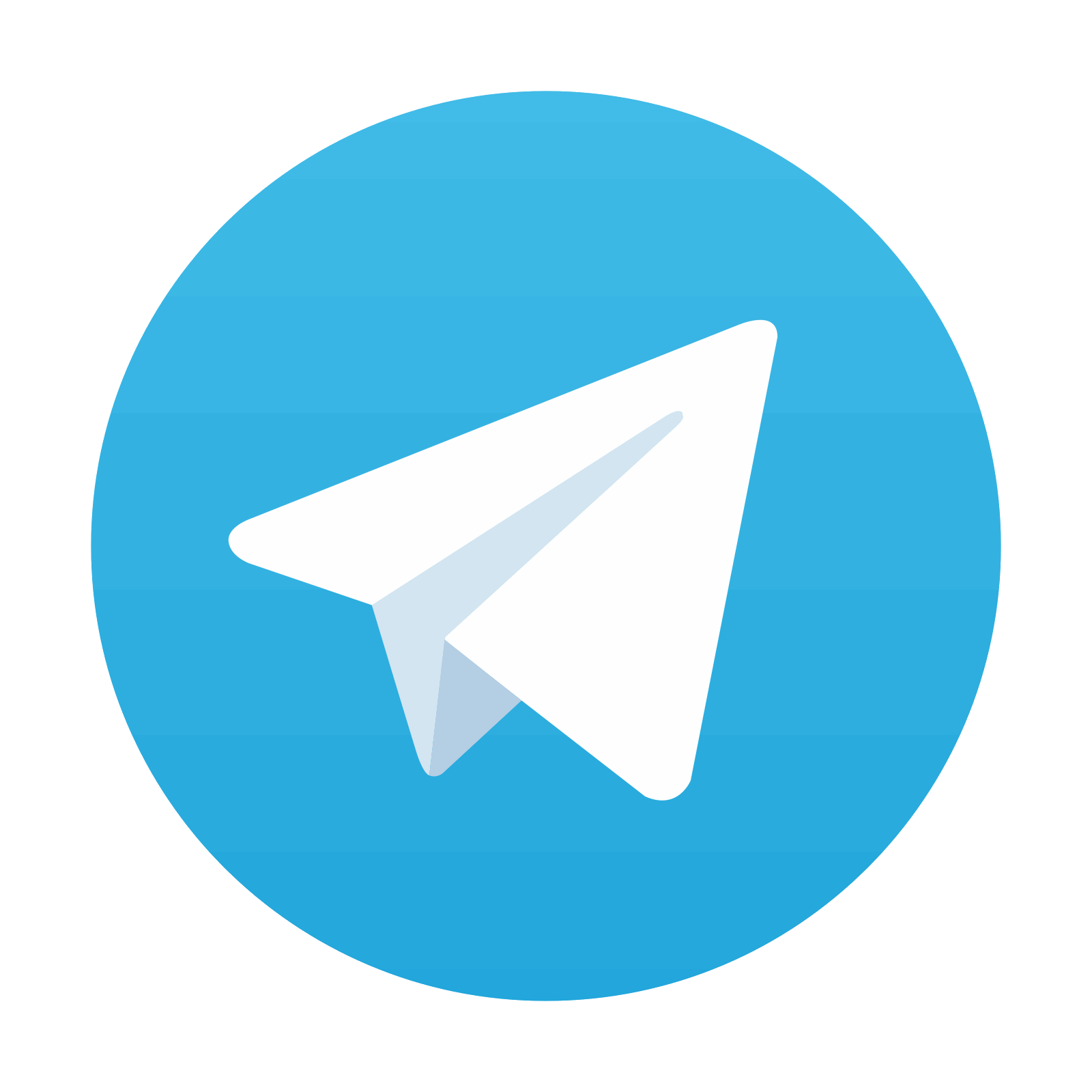
Stay updated, free articles. Join our Telegram channel

Full access? Get Clinical Tree
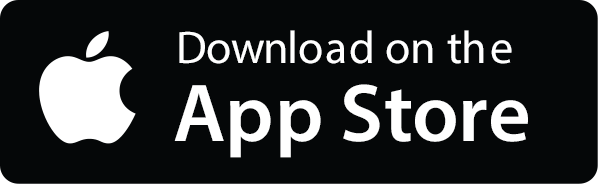
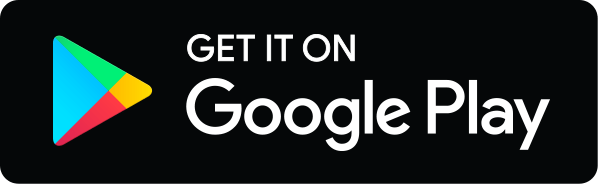